ABSTRACT
A new tray bioreactor was developed for the production of diosgenin from Dioscorea zingiberensis with Trichoderma reesei and Aspergillus fumigatus. The influence of initial moisture content, temperature, tray bed depth and mixing times was investigated. The best fermentation condition is initial moisture content of 75%, bioreactor temperature of 35°C, solid bed depth of 1.5 cm and three mixings carrying out on the first, third and fifth day. Under the optimized fermentation conditions, after 144 h incubation, maximum diogenin concentration of 68.2 μmol/g was detected.
Introduction
Diosgenin is one of the widely used steroidal precursors, as shown in the synthesis of oral contraceptives, sex hormones and other steroids in pharmaceutical industry.[Citation1,Citation2] It usually occurs in plant tubers in glycosidal forms called saponins. Preparation diosgenin from plant tubers mainly depends on hydrolyzation of the glycoside side chain from saponins.[Citation3] Recently, because of the low cost and mild conditions, microbial transformation has been applied for this purpose.[Citation4] The main raw material used in China is Dioscorea zingiberensis C.H. Wright (DZW) for the high concentration of saponins in its tubers.[Citation5] However, until now, most studies have been carried out on a small scale for two reasons. First, the diosgenin yield obtained by means of microbial transformation was lower than that achieved by the traditional method of acid hydrolysis. Second, the microbial transformation reported was carried out through submerged fermentation, which requires high energy and offers low production yield per volume. In order to scale up microbial transformation, a mixed culture solid-state fermentation (SSF) method using Trichoderma reesei and Aspergillus fumigatus was proposed in our previous work. The maximum diosgenin yield of 68.21 μmol/g was obtained after a 156 h incubation period. This value was 7.71% higher than that obtained by submerged fermentation with pure culture.[Citation6]
When SSF is carried out on a large scale, many types of bioreactors can be employed. Among these equipments, the tray bioreactor is the most popular system because of its simple technology.[Citation7] In this study, a new solid-state tray bioreactor was developed. Compared to the traditional one, it provides better air transfer and two sporulations. To obtain a maximum diosgenin yield, the operation parameters, initial moisture content, temperature, bed depth and mixing times were optimized. The mixed culture SSF carried out in the new solid-state tray bioreactor provides a feasible guidance for large-scale operation.
Materials and methods
Materials
DZW tubers were purchased from Yunxi City, Hubei province, China. Cellulase (10,000 IU/g) and α-amylase (2000 IU/g) were purchased from Shandong Longda Bio-product Co., Ltd., Shangdong, China. p-Nitrophenyl-β-D-glucopyranoside (pNPG), p-nitrophenyl-α-L-rhamnopyranoside (pNPR) and standard of diosgenin were supplied by Sigma Company, Shanghai, China. The standard of diosgenin-3-O-[β-D-glucopyranosyl(1→4)]-α-L-rhamnopynosyl (diosgenin-glucoside-rhamnoside) was supplied by Nantong Kanmaike Co., Ltd (Jiangsu, China). The standard of diosgenin-3-O-[β-D-glucopyranosyl(1→4)]-β-D-glucopyranside (diosgenin-diglucoside) and the standard of trillin (diosgenin-glucoside) were from Zhongkangweiye Co., Ltd. (Shanghai, China).
All other chemicals used were of analytical grade and supplied by Sinopharm Chemical Reagent Shanghai Co., Ltd (Shanghai, China).
Micro-organisms
T. reesei (ACCC 30597) was purchased from the Agricultural Culture Collection of China (Beijing, China). A. fumigatus was isolated from natural fermented DZW tubers in our laboratory and identified by SICC (Sichuan Microbiological Resources Infrastructure & Culture Collection Center, China). The two fungal strains were acclimated with 50% (w/v) of saponins and stored at 4 °C with potato dextrose agar (PDA) and sub-cultured every 14 days. To prepare the spores, T. reesei and A. fumigatus were cultured on PDA slopes for seven days. Spores were then harvested with 5 mL of 0.1% (v/v) Tween 80 solution and inoculated to sterilized PDA medium to prepare inoculums.
Pretreatment of DZW
DZW tubers were pretreated to recover starch and fibre from the plant tubers with the method we described previously.[Citation8] The residue was pretreated DZW (PDZW) and was used as the substrate for SSF.
Solid-state fermentation in a new tray bioreactor
A new bioreactor (2 m ×1.5 m × 1 m) was fabricated from plexiglass. Three vertical aluminium shelves 55 cm long, 35 cm wide and 1 m high were placed in the bioreactor. As shown in , each shelf contained four tiers. The layers were made of perforated stainless steel pipes which support the tray. Compared to the traditional tray bioreactor, with the perforated stainless steel, the accessibility of O2 to the tray was increased and the metabolic heat accumulated in the tray bed was released. In order to prevent bacterial contamination, air was filtered through a 0.45 μm cellulose acetate membrane before it was flown into the bioreactor. The fermentation tray with dimensions of 50 cm × 30 cm ×15 cm was made of stainless steel wire-mesh frame. The bottom and the sides of the tray are covered with eight pieces of gauze; the cover of the tray was covered with four pieces of gauze to prevent contamination. The inside of the tray lid was fixed with a rake to ensure uniform distribution of mass and heat transfer in the fermentation process. The temperature and humidity in the bioreactor were auto-controlled by the air conditioner and humidifier in the bioreactor.
Figure 1. Two-dimensional (a) and three-dimensional (b) diagram of the new solid-fermentation bioreactor. 1: ultraviolet (UV) light source; 2: wet conditioner; 3: air conditioner; 4: aluminum shelves; 5: air pump; 6: perforated pipes; 7: tray; 8: sterilized filter; 9: water container; 10: gauze filter: 11: gas exit; and 12: rake.
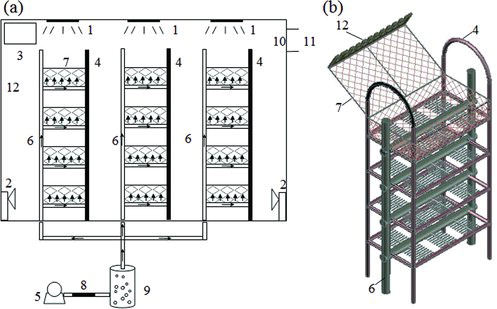
The scaled-up SSF was carried out in the above new bioreactor. Based on the composition of the flask-level experiment, the substrate solid was prepared with medium containing 2.67% peptone and 0.27% K2HPO4 at pH 5.8 and placed in the trays.[Citation9] The solid substrate PDZW and fermentation medium were sterilized at 121 °C for 20 min. Each fermentor tray with fermentation medium containing 7.5 × 106 T. reesei spores/g PDZW was prepared. Fermentation was carried out at 30 ± 1 °C and 200 mL/min of air flow rate. After the first 48 h of fermentation, 100 mL of fermentation medium containing 1.5 × 107 A. fumigatus spores/g PDZW was added and mixed with the metal rake in the tray lid. The experimental conditions were as follows: initial moisture content of 70%–85%, temperature of 30–45 °C, medium thickness from 1 to 2.5 cm and mixing 1–4 times. In this study, four different mixing strategies were applied. In Strategy 1, one mixing was carried out on the second day. In Strategy 2, two mixings were carried out, respectively, on the second and the fourth day. In Strategy 3, three mixings were carried out, respectively, on the first, the third and the fifth day. In Strategy 4, four mixings were carried out on the first, the third, the fourth and the fifth day, respectively.
The duration of the whole fermentation was seven days. For each treatment, samples of 10 g were harvested from the centre and the four sides of the substrate bed every 24 h and the diosgenin concentration was determined. The SSF was then conducted under optimized conditions and the fungal biomass,[Citation10] α-rhamnase and β-glucosidase activities and the saponins concentration in the substrate were detected. All experiments were carried out in triplicate and data presented in figures are mean values.
Analysis
Diosgenin concentration
The fermentation sample was dried at 60 °C and weighed, then mixed with five times its volume of CHCl3 (w/v) and ultrasonicated for 30 min. The CHCl3 extract was filtered through a 0.45 μm membrane and concentrated to dryness. The dried residue was then dissolved in 1 mL of 100% (v/v) methanol. The diosgenin concentration was determined by high-pressure liquid chromatography (HPLC) according to the method described by Huang et al. [Citation5].
Saponins concentration
The change in the saponins (diosgenin-diglucoside, diosgenin-glucoside-rhamnoside, diosgenin-glucoside) in PDZW in the SSF process was determined by HPLC–mass spectrometry (HPLC–MS). The fermentation sample was dried, weighed and extracted with five times its volume of n-butanol (w/v). An Agilent 1100 HPLC coupled with Agilent mass selective detector (MSD) Ion Trap was applied to analyse the n-butanol extracts. The instrument was run with a Zorbax Eclipse XDB-C18 LC column (Agilent 2.1 × 150 mm, particle size 5 μm, pore size 80 Å, monomeric double-end capped) at 40 °C in negative ionization mode in the range of m/z 400–1000 with a flow rate of 1 mL/min. The mobile phase was water (A) and CH3CN (B). The gradient programme was 50–75% (B, v/v) for the first 15 min, 75–92% (B, v/v) for 15–17 min and 92% (B, v/v) for the last 16 min.
α-Rhamnase and β-glucosidase activities
Fermentation samples were suspended in 40 mL water, centrifuged at 10,000 r/min (Eppendorf 5424 centrifuge, Germany) and 4 °C for 15 min. The residue was mixed with 30 mL water and then centrifuged. The solution was combined and adjusted to 100 mL. The enzyme (α-rhamnase or β-glucosidase) activity in the supernatant was detected using pNPR or pNPG as a substrate following the method we reported [Citation10]. One unit (IU) of α-rhamnase (or β-glucosidase) activity was defined as the amount of enzyme releasing 1 μmol of p-nitrophenol in 1 min.
Results and discussion
In our previous paper, the operational parameters of mixed culture SSF were optimized on a shake flask level.[Citation6] When the experiment was scaled up in a tray bioreactor, the initial moisture content, fermentation temperature, tray bed thickness and mixing times were needed to be addressed again.
Initial moisture content
The initial moisture content of the substrate is a key parameter of SSF, which must be taken into consideration to achieve maximum transformation efficiency. It plays important roles in microbial growth, the enzyme productivity and the biotransformation reaction in the SSF process. Fungal growth and product accumulation in this system occur near or at the surface of the solid substrate with low moisture content.[Citation11,Citation12] Higher moisture content might result in the reduction of oxygen transfer and inter-particle spaces, which would hinder the growth of micro-organisms, while lower moisture content might cause a reduc-tion of enzyme yield because of suboptimal growth, high osmotic and less substrate swelling. Thus, providing optimum water level to control the water activity of the substrate to achieve a maximum product yield is critical in SSF. The initial moisture content was investigated in our research in the range of 70%–85%, with an increment of 5%. Every day, samples were collected from the trays and the diosgenin concentration and water loss were determined. As shown in , in all of the experiment runs, a maximum diosgenin concentration of 61.6 μmol/g DZW was observed after six days of fermentation with initial moisture content of 75%. Under these conditions, the moisture dropped to 70.5% after six days of operation. The diosgenin concentration was 52.3, 51.2, 37.7 μmol/g when the initial water content was 70%, 80% and 85%, respectively. The moisture content dropped to 66.7%, 71.2% and 77.9% at the seventh day. The moisture loss during the fermentation was low because of the near-saturated atmosphere in the bioreactor. This feature was attributed to the bioreactor design (outer membrane) and its operation (air is saturated before it enters the cabinet).
Figure 2. Kinetics of diosgenin production (a) in the new SSF reactor with different initial moisture content: 70% (left diagonal






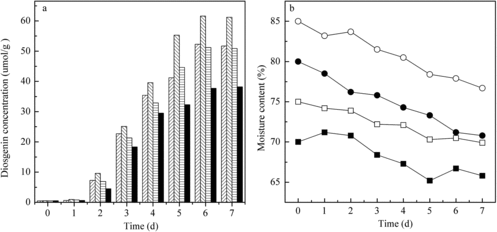
Temperature
Effect of temperature was determined by adjusting the air conditioner on values ranging from 25 to 40 °C with an increment of 5 °C (). It was observed that highest diosgenin concentration (63.2 μmol/g) was produced at 35 °C. Lower or higher temperatures led to the production of diosgenin with slightly lower yields. When the air conditioner was set at 40 °C, the temperature in the tray bed reached 46.9 °C in the fermentation process, which is too high for fungal growth and enzyme production.
Figure 3. Kinetics of diosgenin production (a) in the new SSF reactor at different incubation temperatures: 25 °C (left diagonal






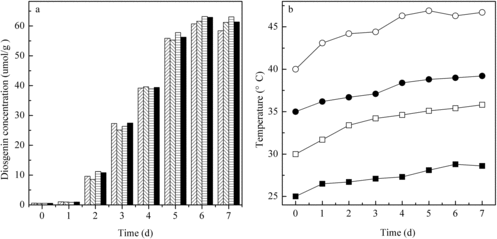
Bed depth
The bed depth is another crucial factor in tray bioreactors. It affects the fungal growth and enzyme production.[Citation7] This parameter was adjusted at values ranging from 1 to 2.5 cm with an increment of 0.5 cm and diosgenin concentration, temperature change and water loss were determined every day. The highest diosgenin concentration was achieved when the PDZW substrate on the tray was 1.5 cm (). As the bed thickness decreased, water loss in the substrate bed was high, which resulted in low diosgenin yield. By increasing the bed depth to 2 cm, the temperature of the PDZW substrate increased significantly during the fermentation process. The metabolic heat produced in the biotransformation reaction accumulated in the bed, which affected the enzyme activity and led to low diosgenin yield. Furthermore, in trays with a greater bed depth, the fugal mycelium accumulated on the cover of the bed, which obstructed the air circulation. As mixing the inoculated substrate can solve this problem, the influence of mixing time was also studied.
Figure 4. Kinetics of diosgenin production (a) in the new SSF reactor with different tray depths: 1 cm (left diagonal






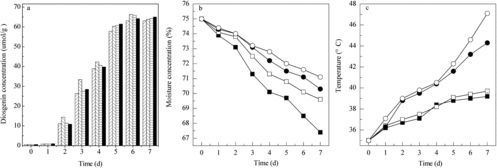
Mixing time
Mixing operation is essential in tray bioreactors. Mixing can break the dense network of fungal mycelium and crush the solid surface to expose the surface area for new sporulation.[Citation13,Citation14] By mixing, the metabolic heat accumulated is released and the high temperature in the fermentation bed can be avoided.
The obtained results indicated that mixing Strategy 3 (i.e. three mixings on alternate days) was the most favourable method for SSF (). In the experiment, mixing can break the PDZW agglomerates to small pieces in the range of 3–6 mm. Thus, new sporulation surface area was formed. With the mixing strategy, the temperature in the tray bed was in the range of 35–38.5 °C and the water content was 70%–75%, which is suitable for fungal growth. Under these conditions, 68.2 μmol/g diosgenin was produced. On the other hand, with less mixing, a dense network was formed on the surface of the PDZW substrate and less new surface was exposed. The diosgenin concentrations obtained with mixing Strategies 1 and 2 were 66.3 and 67.3 μmol/g, respectively. However, more mixings did not result in more diosgenin yield, 67.5 μmol/g diosgenin was achieved with mixing Strategy 4. Excessive mixing may lead to excessive disruption of fungal mycelia, which decreases the sporulation. Compared with continuous mixing operation, Strategy 3 of three mixing times can reduce the bacterial contamination risk and is more economical. These results are similar to the ones reported by Schutyser et al. [Citation15], who found that when wet corn distilled grains were transformed by T. reesei under SSF conditions, frequent mixing operation was not desirable.
Figure 5. Kinetics of diosgenin production (a) in the new SSF reactor with different mixing times: once (left diagonal






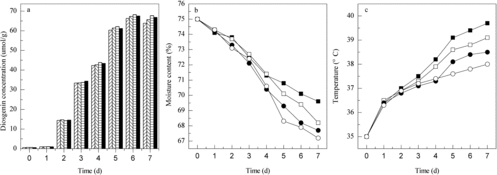
Fungal growth and enzyme production in tray bioreactor
Under the above optimized conditions, the kinetics of fungal growth and enzyme activities in the biotransformation phase were studied. When the two fungal strains were inoculated in PDZW, their growth was exponential in the first two days ((a)). Then, because of the consumption of the carbon source, the fungal growth continued to increase but more slowly and reached a maximum at the sixth day. The α-rhamnase activity increased slowly to reach a maximum value of 26.8 IU/g PDZW at the third day, and then decreased slightly. The highest β-glucosidase activity was observed at the fifth day ((b)).
Figure 6. Kinetics of biomass growth (a) and enzyme activity (b) of α-rhamnase (


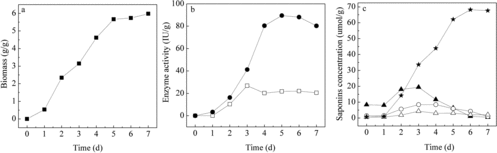
Saponins biotransformation in tray bioreactor
The biotransformation of four main intermediate saponins with time in the SSF system was also studied ((c)). Before biotransformation, the concentrations of diosgenin-diglucoside, diosgenin-gluocoside-rhamnoside, diosgenin-glucoside and diosgenin in the substrate were 8.33, 1.46, 0.58 and 0.66 μmol/g, respectively. In the fermentation phase, the content of diosgenin-diglucoside increased gradually to a value of 19.4 μmol/g in the first three days and then decreased to 0.54 μmol/g slowly in the next four days. The content of diosgenin-glucoside-rhamnoside increased slowly to a value of 8.49 μmol/g in the first four days and then decreased. On the seventh day, 0.98 μmol/g diosgenin-gluocoside-rhanmoside was detected in the PDZW substrate. The concentration of diosgenin-glucoside increased gradually to a value of 4.33 μmol/g in the first three days and then decreased to 2.11 μmol/g slightly in the next four days. The content of diosgenin in PDZW increased sharply and reached a maximum value of 68.2 μmol/g (0.028 g/g) on the sixth day. The results are in agreement with the change of biomass and enzyme activities in the SSF system. During the biotransformation, with α-rhamnase and β-glucosidase produced by T. reesei and A. fumigates, diosgenin-diglucoside, diosgenin-glucoside-rhamnoside and diosgenin-glucoside were produced from longer sugar chain saponins and hydrolyzed to shorter sugar chain saponins. Saponins glycoside, which is bound to diosgenin in saponins, was hydrolysed stepwise from the terminal sugar until an aglycone was released. Moreover, compared to our earlier shake flask experiment,[Citation6] the saponins biotranformation in the tray bioreactor was similar.
Although further optimization using response surface methodology may still be needed, the results obtained in this study suggest that the new tray reactor could be considered promising for large-scale production of diosgenin, as it has the benefits of easy operation, cost effectiveness and an eco-friendly bioreactor design.
Conclusions
A new tray bioreactor for the production of diosgenin from PDZW with T. reesei and A. fumigatus was presented in this study. The operation conditions were optimized for highest transformation efficiency. Maximal diosgenin production was achieved with initial water content of 75%, which is suitable for microbial biotransformation. Diosgenin production was maximized at 35 °C because of the optimal growth temperature for fungal growth and enzyme production. The tray bed depth and mixing times were also optimized. The highest diosgenin concentration was achieved when the PDZW substrate on the trays was 1.5 cm thick and three mixings were carried out on the first, the third and the fifth day. Under the optimum conditions, 68.2 μmol/g (0.028 g/g) diosgenin was obtained. The new tray reactor has the benefits of easy operation, cost effectiveness and an eco-friendly bioreactor design for large-scale production of diosgenin.
Disclosure statement
No potential conflict of interest was reported by the authors.
Additional information
Funding
References
- Lepage C, Leger DY, Bertrand J, et al. Diosgenin induces death receptor-5 through activation of p38 pathway and promotes TRAIL-induced apoptosis in colon cancer cells. Cancer Lett. 2011;301:193–202.
- Mu SM, Tian XS, Ruan YM, et al. Diosgenin induces apoptosis in IGF-1-stimulated human thyrocytes through two caspase-dependent pathways. Biochem Biophys Res Commun. 2012;418:347–352.
- Zhu YL, Zhu HC, Qiu MQ, et al. Investigation on the mechanisms for biotransformation of saponins to diosgenin. World J Microbiol Biotechnol. 2014;30:143–152.
- Fernandes P, Cruz A, Angelova B, et al. Microbial conversion of steroid compounds: recent developments. Enzyme Microb Technol. 2003;32:688–705.
- Huang W, Zhao HZ, Ni JR, et al. The best utilization of D. zingiberensis C. H. Wright by an eco-friendly process. Bioresour Technol. 2008;99:7407–7411.
- Cheng YT, Dong C, Huang CC, et al. Enhanced production of diosgenin from Dioscorea zingiberensis in mixed culture solid state fermentation with Trichoderma reesei and Aspergillus fumigatus. Biotechnol Biotechnol Equip. 2015;29(4):773–778.
- Vaseghi Z, Najafpour GD, Mohseni S, et al. Production of active lipase by Rhizopus oryzae from sugarcane bagasse: solid state fermentation in a tray bioreactor. Int J Food Sci Tech. 2013;48:283–289.
- Zheng TX, Yu LD, Zhu YL, et al. Evaluation of different pretreatments on microbial transformation of saponins in Dioscorea zingiberensis for diosgenin production. Biotechnol Biotechnol Equip. 2014;28(4):740–746.
- Zhu YL, Huang W, Ni JR. Process optimization for production of diosgenin with Trichoderma reesei. Bioprocess Biosyst Eng. 2010;33(5):647–655.
- Zhu YL, Huang W, Ni JR, et al. Production of diosgenin from Dioscorea zingiberensis tubers through enzymatic saccharification and microbial transformation. Appl Microbiol Biotecnol. 2010;85:1409–1416.
- Zhang YY, Liu JH, Zhou YM, et al. Spore Production of Clonostachys rosea in a new solid-state fermentation reactor. Appl Biochem Biotechnol. 2014;174:2951–2959.
- Kumar NSM, Ramasamy R, Manonmani HK. Production and optimization of L-asparaginase from Cladosporium sp. using agricultural residues in solid state fermentation. Ind Crop Prod. 2013;43:150–158.
- Flodman HR, Noureddini H. Effects of intermittent mechanical mixing on solid-state fermentation of wet corn distillers grain with Trichoderma reesei. Biochem Eng J. 2013;81:24–28.
- Casas-Flores S, Rios-Momberg M, Bibbins M, et al. BLR-1 and BLR-2, key regulatory elements of photoconidiation and mycelial growth in Trichoderma atroviride. Microbiology. 2004;150(11):3561–3569.
- Schutyser MAI, de Pagter P, Weber FJ, et al. Substrate aggregation due to aerial hyphae during discontinuously mixed solid-state fermentation with Aspergillus oryzae: experiments and modeling. Biotechnol Bioeng. 2014;83(5):503–513.