ABSTRACT
Twenty-three crude-oil-degrading bacteria were isolated from oil-contaminated sites near the Red Sea. Based on a high growth rate on crude oil and on hydrocarbon degradation ability, four strains were selected from the 23 isolated strains for further study. These four strains were selected on the basis of dichlorophenolindophenol assay. The nucleotide sequences of the 16S rRNA gene showed that these isolated strains belonged to genus Pseudomonas and Nitratireductor. Among the four isolates, strains S5 (Pseudomonas sp., 95%) and 4b (Nitratireductor sp., 70%) were the most effective ones in degrading crude oil. Using a spectrophotometer and gas chromatography–mass spectrometry, degradation of more than 90% of the crude oil was observed after two weeks of cultivation in Bushnell–Haas medium. The results showed that these strains have the ability to degrade crude oil and may be used for environmental remediation.
Introduction
Since the mid-1950s crude oil has gained importance as a source of energy. About 50%–80% of crude oil consist of alkanes, cycloalkanes and aromatic alkanes, and these constituents are called petroleum hydrocarbons.[Citation1] However, there have been serious problems worldwide due to environmental contamination with such petroleum hydrocarbons.
In fact, oil spills are one of the major environmental issues in the last decades. There are a number of notable ongoing oil spills in the world. One such example is the Taylor Energy well spill in the Gulf of Mexico (USA), which was caused by a hurricane on 16 September 2004 and oil leakage still continues today. On 21 January 2011, another oil spill happened in Mumbai (India), resulting in the leakage of about 55 tons of crude oil in the Arabian Sea. Recently, in December 2014, yet another oil spill was caused in the Sundarbans (Bangladesh) due to the collision of a cargo vessel with an oil tanker. Accidents such as these may happen at any time and can cause damage to the environment.
Bioremediation is a promising approach to this problem, as it employs microorganisms to degrade or remove different pollutants, such as organic compounds,[Citation2,Citation3] hydrocarbons and heavy metals,[Citation4,Citation5] from the environment. Various levels of ability to degrade recalcitrant compounds in petroleum have been reported in many bacterial strains and some of them have played a role as primary degraders of spilled oil in the environment. These bacteria can produce biosurfactants, active agents which emulsify these hydrocarbons.[Citation6–8] Representatives of some bacterial genera, such as Acinetobacter, Burkholderia, Gordonia, Dietzia, Brevibacterium, Aeromicrobium, Celeribacter, Mycobacterium and Sphingomonas, isolated from petroleum-contaminated soil have proved to show potential for hydrocarbon degradation.[Citation3,Citation9,Citation10]
For the identification of these bacteria, 16S rRNA gene sequence analysis is often used together with bioinformatics tools. Also, a lot of methods have been developed for screening and evaluating hydrocarbon-degrading bacteria. One of the screening methods for oil-degrading bacteria is the DCPIP (dichlorophenolindophenol) assay. 2,6-DCPIP is an indicator for oxidation of NADH to NAD+ used by oil-degrading bacteria. This assay has been used for rapid and simple isolation of hydrocarbon-degrading bacteria.[Citation11] Quantitative analysis of hydrocarbons is often done by using gas chromatography–mass spectrometry (GC–MS). GC–MS analysis shows that Acinetobacter baumannii and Pseudomonas spp. can degrade more than 50% of hydrocarbons present in the environment.[Citation12,Citation13]
In Saudi Arabia, the petroleum sector accounts for roughly 45% of the budget revenues, 55% of the gross domestic product (GDP) and 90% of the export earnings according the 2014 statistics. On the other hand, Saudi Arabia has an extended coastline on the Arabian Gulf (also known as Persian Gulf) and the Red Sea, where most oil and petrochemical products are exported, viz. Ras Tanura and Yanbu, with continuously increasing risk of water and soil pollution in these areas. This adds to the serious environmental damage that Saudi Arabia experienced in the 1991 Gulf War. As seafloor animals and plants lie at the basis of the food chain, any damage done to the shoreline will ultimately affect the whole shallow-water ecosystem. Considering all this, it became very important to adopt an effective and economic approach to overcome such highly probable contamination.
The aim of this work was to isolate, screen and identify bacterial strains from the coastal area of Yanbu (Saudi Arabia) with potent ability to utilize and degrade hazardous compounds resulting from either oil leaks and accidental spills or petrochemical industry waste contamination. This area was chosen because it plays an important role in the crude-oil export and petrochemicals industry in Saudi Arabia.
Materials and methods
Sampling
For the isolation of crude-oil-degrading bacteria, soil samples were collected from the Red Sea shore of Saudi Arabia. All of the seven collection points were located near the Yanbu industrial Area (24.0833° N, 38.0000° E). Soil samples were taken from 1 to 12 cm below the surface, using a sterile knife.
Isolation of polyaromatic-hydrocarbon–degrading bacteria
The collected soil samples were weighed and 1 g of each was added to 100 mL freshly prepared Bushnell–Haas medium (BHM) and incubated at 30 °C and 180 r/min for one week. Bacteria showing over 1.0 optical density (OD) at 660 nm were spread on Luria–Bertani (LB) agar plates in a biohazard cabinet to isolate bacterial colonies. Colonies from the plates were aseptically taken with a sterile loop and inoculated into 5 mL of LB broth and then incubated at 30 °C and 180 r/min overnight. Later microorganisms were inoculated into 5 mL of BHM containing 1% (w/v) of crude oil obtained from Aramco Refinery and cultivated at 30 °C and 180 r/min for two weeks. After incubation, 1 mL of the suspension was taken from each flask and the OD was checked. Samples with OD over 1.0 at 660 nm were selected for further study.[Citation14,Citation15]
Microscopy
Scanning electron microscope (Hitachi S 5200) was used to characterize microbes morphologically. Samples were prepared and observed under the microscope for the presence or absence of flagella.[Citation16]
Analysis of 16S rRNA
GeneJET Genomic DNA Purification Kit (Thermo Scientific) was used for extraction. Genomic DNA was extracted according to the manufacturer's instructions. Part of each extracted DNA sample was used for 16S rDNA amplification. The 16S rDNA was amplified using 16F27 and 16R1525 primers.[Citation17] The polymerase chain reaction (PCR) programme was set at 30 cycles and the amplification was carried out as follows: 92 °C for 2 min, 42 °C for 30 s, 72 °C for 4 min and 4 °C incubation at the end of the last cycle.[Citation18,Citation19] The amplified fragments were sequenced by Macrogen (Seoul, South Korea) and a phylogenetic tree was constructed using MEGA version 4.[Citation20,Citation21]
Measurement of degradation ratio of long-chain hydrocarbons
Microbial strains were pre-cultured in 5 mL of LB broth medium and incubated at 30 °C and 180 r/min for 24 h. BHM was prepared and added to flasks. To measure the ratio of hydrocarbon degradation, microbes from the pre-cultured flasks were inoculated in 50 mL BHM containing 1% crude oil and cultivated at 30 °C and 180 r/min for two weeks. After incubation, the residual oil was extracted using the chloroform–methanol extraction method. Chloroform and methanol were added in a 2:1 ratio in a separatory funnel; then sample was added to this mixture, it was shaken for 15 s and placed in a stand for 18 h. Oil was separated by phase separation and was recovered in a clean flask. This oil was used for analyzing the quantity of long-chain hydrocarbons using a spectrophotometer (Jenway, Genova) at 400 nm.[Citation22]
2,6-DCPIP assay to estimate hydrocarbon-degrading ability
Colonies from the plates were aseptically taken with a sterile loop and inoculated into 5 mL of LB broth, incubated at 30 °C and 180 r/min to an OD of over 1.0 at 660 nm. Then, 1 mL samples were centrifuged at 4000g for 5 min. The supernatants were discarded and pellets were washed with 0.9% saline. The cell density was adjusted to an OD of 1.0 at 660 nm. The reagents and medium were sterilized; 750 µL of BHM, 50 µL of FeCl3⋅6H2O solution (150 µg/mL) and 50 µL of 2,6-DCPIP solution (37.5 µg/mL) were taken in a 1.5 mL tube. About 80 µL of cell suspension and 5 µL of crude oil were added to this tube and incubated at 30 °C and 100 r/min for 48 h. The colour of the tube was observed after 48 h of incubation. Colourless tubes indicate the presence of microbes that degrade hydrocarbons, and blue colour indicates that hydrocarbons have not been degraded and hydrocarbon-degrading bacteria are absent in the sample.[Citation23]
Gas chromatography–mass spectrometry
Metabolic end-products of crude oil degradation by S5 (Pseudomonas sp.) culture were determined by GC–MS (Shimadzu GC-Q2010, Japan) flame ionization detector (FID) as described in [Citation24]. After two weeks of culture, oil was extracted from the liquid medium. Culture was done in BHM at 30 °C and 180 r/min for two weeks and the remaining oil after degradation was extracted using a separating funnel in the presence of dichloromethane (analytical grade). Magnesium sulphate was used in order to remove the remaining moisture. The programme set for GC–MS was 60 °C for 2 min, then the temperature was increased by 6 °C until it reached 300 °C for 15 min. The operating temperature for the injector was 300 °C and for the detector, 320 °C. Nitrogen was used as a carrier gas. No internal standards were employed. The total time for one GC run was less than 30 min.
Results and discussion
Microorganisms that display remarkable catabolic activities are omnipresent and it is unarguably clear now that microorganisms can inhabit polluted sites and expedite the degradation of crude oil that is polluting the soil. Crude-oil contaminated soils not only affect human health but also take a toll on marine life, animals, birds and crops, as well as on naturally occurring desert plants and the environmental quality as a whole.
This study was carried out to screen for microorganisms in the soil that could potentially utilize crude oil as a sole carbon and energy source, and can thus be used as potential entities for bioremediation of petroleum-contaminated soil breaking down hydrocarbons into carbon dioxide, water and humus.[Citation25,Citation26] In our study, 23 bacterial strains were isolated from the Yanbu region. Four of them were selected for further studies, among which isolate S5 showed high degradation activity. An electron micrograph (SEM) of Pseudomonas sp. S5 is shown in . The procedures for its isolation and characterization are discussed in greater detail in the following subsections.
Figure 1. Bacterial isolate S5 from Yanbu soil on nutrient agar plate (a); Gram staining of isolate S5 with high degradation rate (b); SEM images of isolate S5 at 500× magnification (c) and 5000× magnification (d).
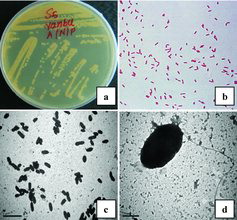
16S rRNA gene sequence analysis
Twenty-three bacterial strains were isolated from enrichment cultures that were maintained at 30 °C for one week. Four isolates that showed higher growth rates on crude oil were selected for further study. They were designated as S3, S4, S5 and 4b. After sequencing of the 16S rRNA gene, data were submitted to the genetic sequence database at the National Center for Biotechnology Information (NCBI). The GenBank ID (http://www.ncbi.nlm.nih.gov) of these strains are KP262042, KP262043, KP262044 and KP262045, respectively. They were molecularly identified as Pseudomonas aeruginosa (KP262042 and KP262043), Pseudomonas sp. (KP262044) and Nitratireductor sp. (KP262045). The phylogenetic tree of these four 16S rRNA gene sequences (KP262042, KP262043, KP262044 and KP262045) was constructed against 16 different P. aeruginosa, Pseudomonas sp. and Nitratireductor sp. from GenBank [Citation20,Citation21] as shown in . The optimal tree with a branch length sum of 47.28 is shown. The data showed that the 16S rRNA gene sequences of the three Pseudomonas species lie in the same cluster with many other P. aeruginosa and Pseudomonas sp. strains from GenBank, reflecting high genetic similarity with these strains. On the other hand, the Nitratireductor sp. isolate (KP262045) was located some distance away from the three other strains, in another cluster. Similar studies have been conducted by different scientists to identify oil-degrading bacteria.[Citation27–29]
Hydrocarbon-degrading bacteria screening
There are three indicators that confirmed the ability of these bacteria to biodegrade crude oil: (i) the change in colour of the culture media from blue to colourless in the DCPIP test, (ii) the disappearance of crude oil from the culture media and (iii) the appearance of bacterial colonies at the bottom of the culture medium. In the DCPIP test, the mechanism of biodegradation of crude oil is evaluated by the introduction of an electron acceptor such as DCPIP to the culture medium. In this colourimetric technique, the ability of bacterial strains to degrade crude oil is assayed by observing the change in DCPIP colour from blue (oxidized) to colourless (reduced).[Citation11] Similar works have reported that bacterial cultures can completely reduce DCPIP at 75, 87, 125 and 138 h for mineral oil, used oil, semi-synthetic oil and synthetic oil, respectively.[Citation30] Mariano et al. [Citation31] performed the DCPIP assay in order to confirm that diesel oil could be degraded by bacterial consortia. Our isolates caused a colour change from blue to colourless, indicating that they have the ability to degrade crude oil.
Growth rate and crude-oil removal by the studied strains
All bacterial strains were grown in 1% crude oil for one week with shaking. After one week, the levels of microbial growth and crude oil biodegradation were analyzed using spectrometry-based methods. Strains S3 (P. aeruginosa), S4 (P. aeruginosa), S5 (Pseudomonas sp.) and 4b (Nitratireductor sp.) showed highest levels of crude-oil biodegradation (), degrading 66%, 65%, 95% and 70% of the oil, respectively. Strain S5 (Pseudomonas sp.) exhibited the highest levels of crude-oil removal (95%) among all isolates. In a similar study, Leahy et al. [Citation32] determined the hydrocarbon-degrading abilities of bacteria spectrophotometrically. Hydrocarbon-degrading percentages were presented by initial and final measurements at 400 nm wavelength. The petroleum-degrading kinetics of isolated bacteria were spectrophotometrically measured at 540 nm.
Table 1. Growth characteristics and crude oil removal by the studied bacterial isolates after one week of incubation.
Crude oil GC–MS analysis
As a next step in our study, the growth medium of the most potent oil-degrading isolate (S5) was analyzed by GC–MS (). The GC–MS profiles of the metabolic intermediates produced during degradation of crude oil by this isolate are shown in b). The major peak compounds at retention times of 9.205, 9.925, 10.595, 11.230, 11.825, 12.388, 12.754 and 12.925 min were identified based on a standard compound library as tridecane, hexadecane, heptadecane, tetracosane, heptacosane, octacosane, nonacosane and hexatriacontane as shown in a). These compounds were present in the crude oil without biodegradation (control) but in the presence of isolate S5 all of the compounds were degraded except for hexatriacontane, which was about 25% degraded as compared to the control.
Figure 3. GC–MS analysis of biodegradation of crude oil (at 30 °C, two weeks of incubation) without microorganism (a) and using isolate S5 (b). Note: Red stars indicate polyaromatic hydrocarbons (PAHs).
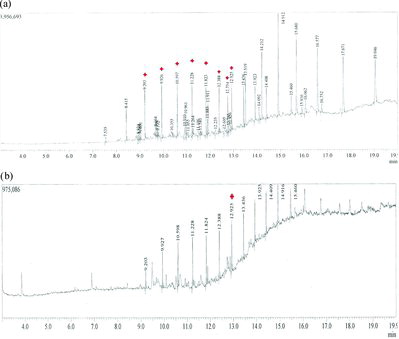
Nearly all the hydrocarbons present in crude oil that were detected using GC–MS analysis were effectively degraded by the isolate from Yanbu region. Thus, the results obtained spectrophotometrically were corroborated by GC–MS analysis. The isolate was able to utilize hydrocarbons such as tridecane, hexadecane, heptadecane, tetracosane, heptacosane, octacosane and nonacosane.[Citation33] The only hydrocarbon that was slightly degraded was hexatriacontane, which remained detectable in the crude oil after degradation by the bacterial isolates.[Citation34] Similar GC–MS analysis has been performed after degradation of oil by A. baumannii.[Citation12] In another work, isolated bacterial organisms were able to reduce at least 50% of the relative abundance of various hydrocarbons present in crude oil compared to control as evaluated by GC–MS analysis.[Citation13] Future research should aim at identification and study of other groups of microorganisms that can act on long-chain hydrocarbons, such as soil fungi and actinomycetes, in the Yanbu area.
Conclusions
Saudi Arabia is one of the biggest oil exporters in the world and most crude oil is exported through Yanbu. This signifies the importance of the city as an economic hub but at a high price. Crude oil spills not only affect the natural environment but also pose severe danger to human health. This highlights the great potential of crude-oil-degrading bacteria in bioremediation. This study confirmed the presence of crude-oil-degrading bacteria in Yanbu soil showing over 90% efficacy of degradation. Of all the isolates, Pseudomonas sp. S5, which showed the highest oil-degrading ability, can possibly be used for bioremediation purposes. This would expedite the process of remediation of the environment in Saudi Arabia from oil pollution.
Acknowlegments
This work was supported by the NSTIP strategic technologies programme in the Kingdom of Saudi Arabia [Project code 12-ENV 2715-3]. The authors also acknowledge assistance from the Science & Technology Unit, Deanship of Scientific Research and Deanship of Graduate Studies, King Abdulaziz University, Jeddah, KSA.
Disclosure statement
No potential conflict of interest was reported by the authors.
Additional information
Funding
References
- Feng JY. Isolation and study of the degradation character of petroleum predominant strains [dissertation]. Xi'an: Xi'an University of Architecture and Technology; 2004.
- Wang W, Zhong R, Shan D, et al. Indigenous oil-degrading bacteria in crude oil-contaminated seawater of the Yellow Sea, China. Appl Microbiol Biotechnol. 2014;98:7253–7269.
- Cao J, Lai Q, Yuan J, et al. Genomic and metabolic analysis of fluoranthene degradation pathway in Celeribacter indicus P73T. Sci Rep. 2015;13(5):7741–7753.
- Alisi C, Musella R, Tasso F, et al. Bioremediation of diesel oil in a co-contaminated soil by bioaugmentation with a microbial formula tailored with native strains selected for heavy metals resistance. Sci Total Environ. 2009;407:3024–3032.
- Plociniczak T, Kukla M, Wątroba R, et al. The effect of soil bioaugmentation with strains of Pseudomonas on Cd, Zn and Cu uptake by Sinapis alba L. Chemosphere. 2013;91:1332–1337.
- Calvo C, Toledo FL, Gonzalez-Lopez J. Surfactant activity of a naphthalene degrading Bacillus pumilus strain isolated from oil sludge. J Biotechnol. 2004;109:255–262.
- Magdalena PP, Plaza GA, Poliwoda A, et al. Characterization of hydrocarbon-degrading and biosurfactant-producing Pseudomonas sp. P-1 strain as a potential tool for bioremediation of petroleum-contaminated soil. Environ Sci Pollut Res. 2014;21:9385–9395.
- Pan XC, Geng S, Mei R, et al. Nitratireductor shengliensis sp. nov., isolated from an oil-polluted saline soil. Curr Microbiol. 2014;69:561–566.
- Chaillan F, Le Fleche A, Bury E, et al. Identification and biodegradation potential of tropical aerobic hydrocarbon-degrading microorganisms. Res Microbiol. 2004;7:587–595.
- Daugulis AJ, McCracken CM. Microbial degradation of high and low molecular weight polyaromatic hydrocarbons in a two-phase partitioning bioreactor by two strains of Sphingomonas sp. Biotechnol Lett. 2003;17:1441–1444.
- Hanson KG, Desai JD, Desai AJ. A rapid and simple screening technique for potential crude oil degrading microorganisms. Biotechnol Tech. 1993;7:745–748.
- Palanisamy N, Ramya J, Kumar S, et al. Diesel biodegradation capacities of indigenous bacterial species isolated from diesel contaminated soil. J Environ Health Sci Eng. 2014;12:142–150.
- Dasgupta D, Ghosh R, Sengupta TK. Biofilm-mediated enhanced crude oil degradation by newly isolated Pseudomonas species. ISRN Biotechnol. 2013;2013:1–13.
- Edward JH, Joanne MJM, Barry JS, et al. Enrichment, isolation, and phylogenetic identification of polycyclic aromatic hydrocarbon-degrading bacteria from Elizabeth River sediments. Appl Environ Microb. 2008;74:1176–1182.
- Ling J, Zhang G, Sun H, et al. Isolation and characterization of a novel pyrene-degrading Bacillus vallismortis strain JY3A. Sci Total Environ. 2011;409:1994–2000.
- Tang YB, Yang X, Chen FY, et al. Screening, identification and degrading gene assignment of a chrysene-degrading strain. African J Biotech. 2011;10(34):6549–6557.
- Hauben L, Vauterin L, Swings J, et al. Comparison of 16S ribosomal DNA sequences of all Xanthomonas species. Int J Syst Bacteriol. 1997;47:328–335.
- Kubota K, Koma D, Matsumiya Y, et al. Phylogenetic analysis of long-chain hydrocarbon-degrading bacteria and evaluation of their hydrocarbon-degradation by the 2,6-DCPIP assay. Biodegradation. 2008;19:749–757.
- Hanan IM, Linda MF, Taghleb MA. Mutational analysis of oil degrading genes in bacterial isolates from oil contaminated soil at the Jordanian oil refinery. World App Sci J. 2009;6(2):208–220.
- Zhao HP, Wang L, Ren JR, et al. Isolation and characterization of phenanthrene-degrading strains Sphingomonas sp. ZP1 and Tistrella sp. ZP5. J Hazard Mater. 2008;152:1293–1300.
- Tamura K, Dudley J, Nei M, et al. MEGA4: Molecular Evolutionary Genetics Analysis (MEGA) software version 4.0. Mol Biol Evol. 2007;24:1596–1599.
- Buckova M, Puskarova A, Chovanova K, et al. A simple strategy for investigating the diversity and hydrocarbon degradation abilities of cultivable bacteria from contaminated soil. World J Microb Biot. 2013;29:1085–1098.
- Thenmozhi R, Nagasathya A, Thajuddin N. Studies on biodegradation of used engine oil by consortium cultures. Adv Environ Biol. 2011;6:1051–1057.
- Joo MH, Kim JY. Characteristics of crude oil biodegradation by biosurfactant-producing bacterium Bacillus subtilis JK-1. J Korean Soc Appl Biol Chem. 2013;56:193–200.
- Sei A, Fathepure BZ. Biodegradation of BTEX at high salinity by an enrichment culture from hypersaline sediments of Rozel Point at Great Salt Lake. J Appl Microb. 2009;107:2001–2008.
- Tamura K, Nei M, Kumar S. Prospects for inferring very large phylogenies by using the neighbor-joining method. Proc Natl Acad Sci USA. 2004;101:11030–11035.
- Yan S, Qiuyu W, Lina Q, et al. Characterization of oil-degrading bacteria from oil-contaminated soil and activity of their enzymes. Biotechnol Biotechnol Equip. 2014;4:3932–3938.
- Mikolasch A, Omirbekova A, Schumann P, et al. Enrichment of aliphatic, alicyclic and aromatic acids by oil-degrading bacteria isolated from the rhizosphere of plants growing in oil-contaminated soil from Kazakhstan. Appl Microbiol Biotechnol. 2015;99(9):4071–4084.
- Hassanshahian M, Zeynalipour MS, Musa FH. Isolation and characterization of crude oil degrading bacteria from the Persian Gulf (Khorramshahr provenance). Mar Pollut Bull. 2014;82:39–44.
- Bidoia ED, Montagnolli RN, Lopes PRM. Microbial biodegradation potential of hydrocarbons evaluated by colorimetric technique: a case study. In: Mendez-Vilas A, editor. Current research, technology and education topics in applied microbiology and microbial biotechnology. Badajoz: Formatex Research Center; 2010. p. 1277–1288.
- Mariano AP, Bonotto DM, de Angelis DF, et al. Biodegradability of commercial and weathered diesel oils. Brazil J Microbiol. 2008;39:133–142.
- Leahy JG, Tracy KD, Eley MH. Degradation of mixtures of aromatic and chloroaliphatic hydrocarbons by aromatic hydrocarbon-degrading bacteria. FEMS Microbiol Ecol. 2003;43:271–276.
- Norman RS, Moeller P, McDonald TJ, et al. Effect of pyocyanin on a crude-oil-degrading microbial community. Appl Environ Microbiol. 2004;70(7):4004–4011.
- Hamamura N, Sarah HO, David MW, et al. Microbial population dynamics associated with crude-oil biodegradation in diverse soils. Appl Environ Microbiol. 2006;72(9):6316–6324.