ABSTRACT
The aim of this study was to evaluate the effects of crude extract of Eupatorium lindleyanum DC. (CEEL) on reducing hyperlipidemia and preventing atherosclerosis induced with a high cholesterol diet (HCD) in rabbits. The levels of total cholesterol, triglyceride and low-density lipoprotein cholesterol were lower in the serum of rabbits fed HCD plus CEEL or simvastatin than in the serum of rabbits fed HCD. Rabbits in the CEEL and simvastatin groups showed a significant decrease in the expression of C-reactive protein, an increase in the level of NO and low level expression of vascular cell adhesion molecule-1 compared with the model group (fed with HCD). Feeding CEEL to rabbits significantly reduced severe atherosclerosis in the aorta. Histopathological examination showed that CEEL reduced foam cell formation and inhibited smooth muscle cell migration in the blood vessel of rabbits. These effects may account for beneficial effects of CEEL in hyperlipidemia and atherosclerosis.
Introduction
Atherosclerosis (AS), the complex interaction of serum cholesterol with the cellular components of the arterial wall, is the leading cause of cardiovascular disease worldwide, and continues to result in a high incidence of death.[Citation1–3] It has become a major health ailment and a serious social problem. Hypercholesterolemia or more specifically elevated plasma low-density lipoprotein cholesterol (LDL-C) is a key risk factor for development and progression of atherosclerosis.[Citation4] During atherosclerosis, uncontrolled cholesterol and lipid accumulation in macrophages and smooth muscle cells lead to foam cell formation and to progression of the atherosclerotic plaque.[Citation5]
Over the years, intensive efforts have been made to develop new and more effective agents for controlling serum lipids, lipoproteins and atherosclerosis. This has led to the discovery of a wide range of natural and synthetic hypolipidemic and antihypercholesterolemic agents. Statins and fibrates are the most commonly used cholesterol-lowering agents because of their lipid-lowering, anti-inflammation and endothelial protection activities.[Citation6] They have achieved good clinical results but also cause certain side effects.[Citation7–9]
Pharmacological studies have shown that several traditional Chinese medicines (TCMs) possess similar capabilities to decrease the risk of cardiovascular disease as statins, thereby making them candidates for atherosclerosis therapy.[Citation10–16] It is generally assumed that the active constituents contributing to these protective effects are antioxidant molecule such as flavonoid, phenolic acid and anthocyanin.
Eupatorium lindleyanum DC., a perennial herbaceous plant, is a traditional Chinese herb. Its aerial part is called ‘Yemazhui’. It has been used in China and other Asian countries as a traditional medicine for the treatment of cough, fever and tracheitis.[Citation17–20] E. lindleyanum has been shown to contain volatile oil, flavonoids and alkaloids, coumarins, sesquiterpenes and esters, and some of these compounds are well-known antioxidants.[Citation21–23] Its extract also demonstrates bacteriostatic, anti-inflammatory and antiviral activities in pharmacological experiments. For example, Ji et al. [Citation19] reported that acidic environment or medium helps enhance the antimicrobial activity of a decoction of E. lindleyanum. Yan et al. [Citation24] found that the water extract and residue water extract have the strongest antioxidant activities, and the n-butanol fraction of the ethanol extract also possesses high antioxidant abilities, except for metal-chelating activity, while the ethanol extract and petroleum ether, ethyl acetate and water fractions were relatively weak antioxidants. However, information is scanty on the hypolipidemic and antiatherogenic effects of E. lindleyanum.
In this study, we aimed to examine for the first time the effects of crude extract of E. lindleyanum (CEEL) on reducing hyperlipidemia and preventing atherosclerosis induced by a high cholesterol diet (HCD) in rabbits. The important findings of this research are that evidence is provided for the hypolipidemic and antiatherogenic capacities of CEEL and its possible use in the prevention of cardiovascular diseases.
Materials and methods
Chemicals and reagents
Simvastatin (SVS) was produced by Merck Sharp & Dohme Pharmaceutical Co., Ltd. (Hangzhou, China). A total cholesterol (TC) kit, triglyceride (TG) kit, LDL-C kit, high-density lipoprotein cholesterol (HDL-C) kit and NO kit were purchased from Jiancheng Bioengineering Institute (Nanjing, China). An enzyme-linked immunosorbent assay C-reactive protein (CRP) kit was purchased from Kangyuanruide Bio-technology Co., Ltd. (Beijing, China). The reagents for RNA extraction and reverse transcription PCR (RT-PCR) were from Takara Biotech (Dalian, China). All other reagents were commercial products of the highest purity grade available.
Plant material and extraction
The whole plant of E. lindleyanum was obtained from Xuyu County, Jiangsu Province, China, in September 2012 and was identified at the Institute of Material Medical Planting, Chongqing Academy of Chinese Materia Medica, Chongqing, China, by a plant taxonomist. A voucher specimen was deposited in the Institute of Material Medical Planting for reference purposes.
The aerial part of E. lindleyanum was dried at 50 °C–60 °C until the moisture content lowered to 6%, and then it was ground into a powder of about 60 mesh. The dried powder of E. lindleyanum (100 g) was extracted three times with 95% ethanol by refluxing for 2 h. After removal of the ethanol under reduced pressure, a dark green residue (9.7 g) was obtained, which was suspended in H2O (0.12 L) to form a suspension. The suspension was filtered with Whatman No. 1 paper and the filtered liquor was subjected to a column (40 cm × 3 cm) packed with D101 macroporous resin, and eluted by the stepwise addition of H2O (0.3 L) and 70% ethanol (0.15 L) to collect two major fractions. The 70% ethanol fraction was concentrated and freeze-dried to produce the solid extract (2.2 g), CEEL. The CEEL was stored at 4 °C until use. The content of total flavonoids in CEEL was determined spectrophotometrically as described by Chen et al. [Citation25].
Animals and experimental design
Sixty healthy male New Zealand rabbits were obtained from the Laboratory Animal Center, Chongqing Academy of Chinese Materia Medica. The average age of the rabbits was approximately 16 weeks (2.0–2.1 kg). At the start of treatment, rabbits were housed individually in separate steel cages maintained at 24 °C and 50%–60% relative humidity and a 12 h light/dark cycle throughout the experiment. All experiments were performed in accordance with the Experimental Animal Regulations approved by the China National Science and Technology Commission.
The rabbits were acclimatized to laboratory conditions for 7 days prior to treatment and fed standard chow and water ad libitum. After stabilization, the rabbits were divided randomly into six groups of 10 rabbits each. The control group consumed the standard chow. The model group and the other four groups were fed with an HCD, containing 95.7% standard chow, 3% lard oil and 1.3% cholesterol to provoke atherosclerotic process.[Citation26] At the same time, one of the above four groups, the SVS group, was orally treated with SVS 2.2 mg/kg body weight in addition to HCD. And the groups H-CEEL, M-CEEL and L-CEEL were fed with HCD and orally administered CEEL at the doses of 8.34, 4.17 and 2.08 g/kg body weight, respectively. Food intake for each rabbit was standardized to 120 g/day throughout the experiment for 10 weeks. The animals were clinically observed and weighed weekly.
Serum lipid profile
On the 6th and 10th week, blood samples were collected from the marginal ear vein, after an overnight fast. Serum was separated by centrifugation (2000×g, 20 min, 4 °C) and used for the measurement of TC, TG, LDL-C and HDL-C, according to the manufacturers’ protocols by using an automatic biochemical analyzer (GFD-800, Gaomi Caihong Analyzer Co., Ltd., Shandong China). The serum concentrations of NO and CRP were measured using commercially available kits, according to the manufacturers’ protocols.
Evaluation of atherosclerotic lesions
At the end of the 10-week experiment, all rabbits were sacrificed by exsanguinations after deep anaesthesia with sodium pentobarbital (150 mg/kg) via the marginal ear vein. The aorta arch and thoracic aortas were gently removed to protect the endothelial lining, and cleaned carefully of adhering soft tissue with ice cold sterile physiologic saline. Thoracic aortas were rapidly dissected, opened longitudinally and stained with Sudan III. The aortic luminal surface was photographed using a camera (DSC-S85, Sony, Japan). The surface area of lesion and whole aorta were measured by analysis of the photos. Target areas were traced by an investigator blind to the treatment. The areas and intima-media thickness (IMT) were measured using Image-Pro Express 5.0 software (Image-Pro, Sydney, Australia). The percentage of the lesion area in the aortic area was calculated as the ratio of the plaque area to the total aorta area.
Aortic arches were rapidly dissected and kept at −80 °C or in 10% neutral-buffer formalin. The specimens were embedded in paraffin and cut serially to make cross-sectional samples. The sections were stained with hematoxylin and eosin (H&E). The stained sections were examined blindly to evaluate the presence of atherosclerotic lesions. The pathological changes were observed under an optical microscope (BX51, Olympus Company, Tokyo, Japan).
Reverse transcription PCR
The expression of vascular cell adhesion molecule-1 (VCAM-1) mRNA was tested using RT-PCR. Primers for VCAM-1 and glyceraldehyde-3-phosphate dehydrogenase (GAPDH, as an internal control gene) were designed on the basis of the mRNA sequence in GeneBank. The primers were 5'-GAACACTCTTACCTGTGC ACAGC-3' and 5'-GCCATCCTCATAGCAATTAAGGTGAG-3' for VCAM-1, resulting in a 567 bp product; 5'-GTGAAGGTCGGAGTCAACG-3' and 5'-GGTGAAGACGCCAGTGGACTC-3' for GADPH, with a 299 bp product. The PCR conditions were 94 °C for 240 s, followed initially by 32 cycles of 94 °C for 40 s, 60 °C for 60 s, and 72 °C for 60 s with a final step of 72 °C for 60 s. The PCR products were analyzed by agarose gel (2%) electrophoresis (DYCP-31E, Liuyi Instrument Factory, Beijing, China).
Statistical analysis
Statistical analyses were conducted using SPSS ver 11.5 (SPSS, Chicago, IL, USA). Data are the mean values with standard deviations (± SD). Comparisons between groups were evaluated by one-way ANOVA and comparisons between two groups were analyzed using Fisher's protected least significant difference test. A p-value of <0.05 was taken to indicate a significant difference.
Results and discussion
Measurement of serum lipids
The total flavonoid content in CEEL was 70.3%. Serum levels of TC, TG, HDL-C and LDL-C were detected at the end of the 6th and 10th week (). In comparison with the control group, higher serum levels of TC, TG and LDL-C in all the other groups were detected during the whole experiment. As shown in (A), the serum TC value of the control group was at a low level, 1.39 ± 0.23 mmol/L at 6th week and 1.46 ± 0.31 mmol/L at 10th week, whereas the serum TC value greatly increased in the model group. However, SVS and CEEL treatment significantly lowered the serum TC in rabbits on HCD. After a six-week treatment with SVS, the serum TC level was lowered by 30% in the SVS group (16.90 ± 2.87 mmol/L) compared to the model group (24.05 ± 3.48 mmol/L). The serum TC level was lowered by 22% in the H-CEEL group (18.76 ± 3.05 mmol/L), 18% in the M-CEEL group (19.75 ± 4.33 mmol/L) and 12% in the L-CEEL group (21.14 ± 4.01 mmol/L), accordingly. At the end of the experiment, the serum TC level was lowered by 56% in the H-CEEL group (19.97 ± 3.61 mmol/L), 46% in the M-CEEL group (24.85 ± 4.53 mmol/L) and 39% in the L-CEEL group (28.13 ± 4.93 mmol/L) compared to the model group (46.30 ± 6.73 mmol/L).
Figure 1. Effect of CEEL on the serum level of TC (A), TG (B), LDL-C (C) and HDL-C (D). Data are mean values (± SD) from 10 rabbits per group. *p < 0.05, #p < 0.01 versus the model group.
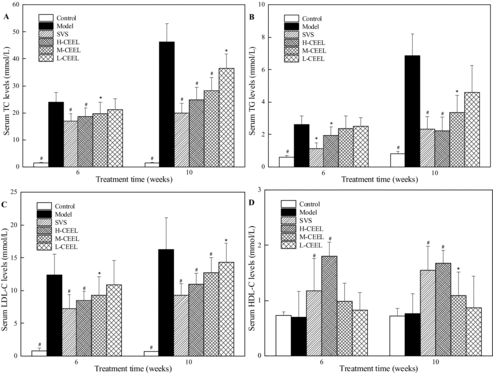
In addition, CEEL showed potential to control the serum TG to a similar extent as the serum TC in hyperlipidemic rabbits ((B)). After a 6- and a 10-week treatment with CEEL, the TG value was decreased by 26% and 67% in the H-CEEL group (1.94 ± 0.54 and 2.23 ± 0.84 mmol/L), by 10% and 51% in the M-CEEL group (2.36 ± 0.79 and 3.35 ± 1.08 mmol/L) and by 4% and 33% in the L-CEEL group (2.52 ± 0.53 and 4.59 ± 1.65 mmol/L) compared to the model group (2.63 ± 0.52 and 6.85 ± 1.36 mmol/L), respectively. Moreover, CEEL could also significantly decrease the serum LDL-C concentration ((C)). In comparison with the model group (12.38 ± 3.14 and 16.26 ± 4.85 mmol/L), the LDL-C value decreased by 31% and 32% in the H-CEEL group (8.45 ± 1.43 and 10.95 ± 1.73 mmol/L), by 25% and 22% in the M-CEEL group (9.26 ± 2.87 and 12.71 ± 2.34 mmol/L) and by 12% and again 12% in the L-CEEL group (10.87 ± 3.74 and 14.29 ± 2.91 mmol/L) after a 6- and a 10-week treatment with CEEL, respectively. In contrast, an increase in the HDL-C level was detected in the groups that were given CEEL and SVS by oral administration, whereas in the model group, no significant effect on the HDL-C level was observed ((D)).
The high-fat diet induced a significant increase in the serum levels of TC, TG and LDL-C in the model group compared with the control group. Not only was a significant reduction in the levels of TC, TG, and LDL-C observed, but also an increase in the HDL-C level, in the three CEEL groups. The H-CEEL and the SVS group can result in the same efficacy of reduction in the levels of TC, TG and LDL-C and augmentation of the HDL-C level. Moreover, CEEL had a dose-dependent hypolipidemic effect on all the examined indicators. These results suggested that CEEL could prevent the development of AS by regulating the serum level of cholesterol.
Expression of CRP
AS is a chronic inflammatory disease involving several key molecules, such as CRP, a marker of cardiovascular disease.[Citation27,Citation28] The effect of CEEL treatment upon the expression of CRP is summarized in . Compared with the control group, the concentration of CRP in the model group increased significantly. The rabbits in the SVS group showed a significant decrease in the expression of CRP compared with the model group. The rabbits in the CEEL group exhibited a significant decrease in the CRP levels when compared with the model group. Hence, the anti-atherosclerotic effect of CEEL correlated with the suppression of inflammatory responses in rabbits.
Concentration of NO in blood
The decreased level of NO is associated with endothelial dysfunction, which has been suggested to be an early marker of AS.[Citation29] Compared with the NO level in the control group rabbits, that in the model rabbits was reduced significantly (). However, the level of NO increased significantly in the SVS and CEEL groups. In the SVS group, the NO level (125.81 ± 11.08 μmol/L) increased by 166% compared to the model group (47.29 ± 8.29 μmol/L). The NO level increased by 148% in the H-CEEL group (117.41 ± 9.61 μmol/L), 85% in the M-CEEL group (87.87 ± 13.47 μmol/L) and 36% in the L-CEEL group (64.34 ± 11.18 μmol/L). These results suggested that administration of CEEL could produce beneficial effects in AS rabbits by protecting the endothelium.
Measurement of atherosclerotic lesion
Atherosclerotic lesions were found in the model, SVS and CEEL groups but not in the control group. The model group rabbits developed more serious lesions than those in the SVS and the CEEL groups. The area of atherosclerotic lesion as a ratio to that of the whole aorta was calculated to evaluate the size of lesion (). The percentage of the plaque area of the normal, model, SVS group, H-CEEL, M-CEEL and L-CEEL groups are given in The atherosclerotic lesion was significantly reduced in the SVS and the CEEL groups compared to the model group.
Table 1. Effect of CEEL on the plaque area and IMT of the aortic in hyperlipidemic rabbits on high cholesterol diets.
The IMT was measured to assess changes in the aortic arch after CEEL treatment (). Compared with the control group (0 mm), the IMT of the aortic arch of the model rabbits (245.8 ± 34.6 mm) was significantly thicker. After the rabbits were treated with SVS or CEEL, the IMT was significantly thinner compared with the model rabbits.
Histopathological findings
The histopathological changes in the rabbit aorta were examined by H&E staining as shown in . In the control group, no histopathological changes were observed by optical microscopy. The vessel wall of the control group showed uniform thickness with no bulging in the lumen and the endothelial lining was intact without any interruption. On the other hand, all rabbits on HCD developed a large number of foam cells. In the model group, the vessel wall appeared to be thicker and less smooth. Extensive atherosclerotic plaques containing foam cells, inflammatory cells, cholesterol crystals and tissue calcification were observed. Compared with the model group, the SVS and CEEL groups showed a visible reduction of pathological changes. Also, the vessel walls were slightly rougher and thicker, and fewer atherosclerotic plaques were observed than in the model group. Moreover, the preventive treatment with CEEL dose-dependently improved the vascular remodeling induced by HCD.
Expression of VCAM-1
As an initial event in the pathogenesis of atherosclerosis, local adherence of circulating monocytes to the vascular endothelial cells is one of the earliest morphological alterations. The expression of adhesion molecules, particularly VCAM-1, is a key factor in the recruitment of circulating monocytes into intima.[Citation30] VCAM-1, a member of the immunoglobulin gene superfamily, is involved in atherogenesis by aiding firm adhesion of monocytes to vascular endothelium.[Citation31] Some animal studies have demonstrated that VCAM-1 plays an important role in initiation of atherosclerosis.[Citation32,Citation33] As shown in , the expression level of VCAM-1 was low in the control group, whereas it significantly increased in the model group. This result indicated that diet-induced hyperlipidemia up-regulated the expression of VCAM-1. The expression of VCAM-1 in the SVS and the CEEL groups was significantly down-regulated with respect to that in the model group. It could be speculated that the administration of CEEL could possibly reduce the expression of VCAM-1 by blocking the activation of nuclear factor-kappa B (NF-κB).[Citation34]
Conclusions
The present findings suggested that the crude extract of CEEL could not only decrease the levels of serum lipids and inflammatory responses, but could also regulate the endothelial function of atherosclerotic rabbits. In combination, these effects may account for its beneficial effects in hyperlipidemia and atherosclerosis. Therefore, further investigations need to be performed in order to explore the precise mechanism and therapeutic potential of CEEL in treating hyperlipidemia and the related cardiovascular diseases.
Disclosure statement
No potential conflict of interest was reported by the authors.
Additional information
Funding
References
- Glass CK, Witztum JL. Atherosclerosis: the road ahead. Cell. 2001;104:503–516.
- Pang J, Xu Q, Xu X, et al. Hexarelin suppresses high lipid diet and vitamin D3-induced atherosclerosis in the rat. Peptides. 2010;31:630–638.
- Weber C, Noels H. Atherosclerosis: current pathogenesis and therapeutic options. Nat Med. 2011;17:1410–1422.
- Wald NJ, Law MR. Serum cholesterol and ischemic heart disease. Atherosclerosis. 1995;118:15–55.
- Zingg JM, Ricciarelli R, Azzi A. Scavenger receptor regulation and atherosclerosis. Biofactors. 2000;11:189–200.
- Wierzbicki AS, Mikhailidis DP, Wray R. Drug treatment of combined hyperlipidemia. Am J Cardiovasc Drug. 2001;1:327–336.
- Beltowski J, Wójcicka G, Jamroz-Wiśniewska A. Adverse effects of statins mechanisms and consequences. Curr Drug Safety. 2009;4:209–228.
- Florentin M, Liberopoulos EN, Mikhailidis DP, et al. Fibrate associated adverse effects beyond muscle and liver toxicity. Curr Pharm Des. 2008;14:574–587.
- Vasudevan AR, Hamirani YS, Jones PH. Safety of statins: effects on muscle and the liver. Cleveland Clin J Med. 2005;72:990–993.
- Chen J, Mao D, Yong Y, et al. Hepatoprotective and hypolipidemic effects of water-soluble polysaccharidic extract of Pleurotus eryngii. Food Chem. 2012;130:687–694.
- Li J, Chen C, Shen Y. Effects of total glucosides from paeony (Paeonia lactiflora Pall) roots on experimental atherosclerosis in rats. J Ethnopharmacol. 2011;135:469–475.
- Verma PR, Deshpande SA, Kamtham YN, et al. Hypolipidemic and antihyperlipidemic effects from an aqueous extract of Pachyptera hymenaea (DC.) leaves in rats. Food Chem. 2012;132:1251–1257.
- Wang L, Geng C, Jiang L, et al. The anti-atherosclerotic effect of olive leaf extract is related to suppressed inflammatory response in rabbits with experimental atherosclerosis. Eur J Nutr. 2008;47:235–243.
- Godard M, Decorde K, Ventura E, et al. Polysaccharides from the green alga Ulva rigida improve the antioxidant status and prevent fatty streak lesions in the high cholesterol fed hamster, an animal model of nutritionally-induced atherosclerosis. Food Chem. 2009;115:176–180.
- Wang Y, Lin R, Cheng H, et al. Anti-atherogenic effect of San-Huang-Xie-Xin-Tang, a traditional Chinese medicine, in cultured human aortic smooth muscle cells. J Ethnopharmacol. 2011;133:442–447.
- Zha X, Xiao J, Zhang H, et al. Polysaccharides in Laminaria japonica (LP): extraction, physicochemical properties and their hypolipidemic activities in diet-induced mouse model of atherosclerosis. Food Chem. 2012;134:244–252.
- Yan G, Ji L, Luo Y, et al. Preparative isolation and purification of three sesquiterpenoid lactones from Eupatorium lindleyanum DC. by high-speed counter-current chromatography. Molecules. 2012;17:9002–9009.
- Huo J, Yang S, Ding J, et al. Two new cytotoxic sesquiterpenoids from Eupatorium lindleyanum DC. J Integr Plant Biol. 2006;48:473–477.
- Ji L, Luo Y, Yan G. Studies on the antimicrobial activities of extracts from Eupatorium lindleyanum DC against food spoilage and food-borne pathogens. Food Control. 2008;19:995–1001.
- Ye G, Huang X, Li Z, et al. A new cadinane type sesquiterpene from Eupatorium lindleyanum (Compositae). Biochem Syst Ecol. 2008;36:741–744.
- Yang N, Qian S, Duan J, et al. Studies on the chemical constituents of Eupatorium lindleyanum. J China Pharm Univ. 2003;34:220–221.
- Yang N, Qian S, Duan J, et al. Two new sesquiterpenes from Eupatorium lindleyanum. Chin Chem Lett. 2006;16:1223–1226.
- Yang N, Duan J, Shang E, et al. Analysis of sesquiterpene lactones in Eupatorium lindleyanum by HPLC-PDA-ESI-MS/MS. Phytochem Anal. 2009;21:144–149.
- Yan G, Ji L, Luo Y. Antioxidant activities of extracts and fractions from Eupatorium lindleyanum DC. Molecules. 2011;16:5998–6009.
- Chen C, Hsu J, Wang S, et al. Hibiscus sabdariffa extract inhibits the development of atherosclerosis in cholesterol-fed rabbits. J Agric Food Chem. 2003;51:5472–5477.
- Bocan TM, Mueller SB, Mazur MJ, et al. The relationship between the degree of dietary induced hypercholesterolemia in the rabbit and atherosclerotic lesion formation. Atherosclerosis. 1998;102:9–22.
- Nishida M, Moriyama T, Ishii K, et al. Effects of IL-6, adiponectin, CRP and metabolic syndrome on subclinical atherosclerosis. Clinica Chimica Acta. 2007;384:99–104.
- Ross R. Atherosclerosis – an inflammatory disease. New England J Med. 1999;340:115–126.
- Félétou M, Vanhoutte PM. Endothelial dysfunction: a multifaceted disorder (The Wiggers Award Lecture). Am J Physiol. 2006;3:985–1002.
- Deem TL, Cook-Mills JM. Vascular cell adhesion molecule 1 (VCAM-1) activation of endothelial cell matrix metalloproteinases: role of reactive oxygen species. Blood. 2004; 104: 2385–2393.
- Dansky HM, Barlow CB, Lominska C, et al. Adhesion of monocytes to arterial endothelium and initiation of atherosclerosis are critically dependent on vascular cell adhesion molecule-1 gene dosage. Arteriosclerosis Thromb Vasc Biol. 2001;21:1662–1667.
- Blankenberg S, Barbaux S, Tiret L. Adhesion molecules and atherosclerosis. Atherosclerosis. 2003;170:191–203.
- Cybulsky MI, Iiyama K, Li H, et al. A major role for VCAM-1, but not ICAM-1, in early atherosclerosis. J Clin Investig. 2001;107:1255–1262.
- Carluccio MA, Siculella L, Ancora MA, et al. Olive oil and red wine antioxidant polyphenols inhibit endothelial activation: antiatherogenic properties of mediterranean diet phytochemicals. Arteriosclerosis Thromb Vasc Biol. 2003;23:622–629.