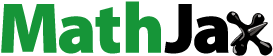
ABSTRACT
Silver nanoparticles have a high antimicrobial activity and are broadly utilized for several disinfection purposes including water and materials’ sanitization for medical purposes. There have been comparatively few studies on using silver against plant pathogenic fungi. In this study, silver nanoparticles (Ag NPs) were used at concentrations of 0.0, 0.0002, 0.0005, 0.0007, 0.0009, 0.0014 and 0.0019 mol/L. Six different Rhizoctonia solani anastomosis groups (AGs) infecting cotton plants were treated in vitro with Ag NPs on Czapek Dox agar (CDA) and potato dextrose agar plates. The results showed that various concentrations of Ag NPs have antifungal properties to control R. solani AGs. The obtained results also revealed that strong inhibition of R. solani AGs was noticed on CDA at all concentrations.
Introduction
Nanotechnology is a field of science which includes synthesis and development of several nanomaterials. Nanoparticles have unparalleled physicochemical, biological and optical characteristics, and are used as antimicrobials in different fields. They also have many applications in [Citation1] optical devices,[Citation2] catalytic processes,[Citation3] biological labelling,[Citation4] sensor technology [Citation5] and electronics [Citation6] and may suppress the expression of proteins associated with adenosine triphosphate production.[Citation7] In spite of that, some of the specific antimicrobial mechanisms which affect the silver are not well understood. Liu et al. [Citation8] found that micromolar dosages of silver and copper ions may kill bacteria in water, whereas high doses of silver ions may be toxic to mammals,[Citation9,Citation10] marine [Citation11] and freshwater organisms.[Citation12] Such concentrations of silver do not have adverse effects on human beings.[Citation13] As an alternative to synthetic fungicides, the use of silver nanoparticles (Ag NPs) as antifungal agents has become more widespread, as scientific progress make their production more cost-effective (). One of the applications that Ag NPs can be used in is plant diseases management. So far, there have been a few reports for silver nanoparticles’ applications against plant pathogenic fungi.[Citation14–22] The basic requirement for the possible usage of silver against plant diseases is gaining more information about the antimicrobial activity of several silver compounds. Another requirement is the development of strategies for the increase of the application effectiveness of Ag NPs in order to suppress plant pathogens. The aim of the present study was to synthesise silver NPs and to determine their inhibitory effect against R. solani groups, which infect cotton plants.
Table 1. Studies on the effect of silver nanoparticles against different micro-organisms.
Materials and methods
Synthesis of silver NPs
For the synthesis of Ag NPs, AgNO3 solution (1.0 mmol/L) was utilized as a metal salt precursor, whereas sodium citrate solution (1.0 mmol/L) was used as a reducing and stabilizing agent at 25 ºC ± 2 ºC. The clear colourless solution was changed to the characteristic pale yellow, when sodium citrate was utilized as a stabilizing agent. The incidence of colour indicated the formation of Ag NPs, which were purified by centrifugation (Hettich Instruments LP, Beverly, MA 01915, USA) at 9000 rpm for 14 min to discard any indissoluble material. To remove excess Ag+, the silver colloids were washed four times with deionized water (Deionized Water Unit, WDI-08/8 liter per hour, Republic of Korea). A powder of the nano-sized Ag+ was obtained after drying the sample in oven at 60 ºC for 24 h.
Characterization of NPs
The silver nanoparticles’ characterization by ultraviolet-visible (UV-Vis) absorption spectra was carried out by PerkinElmer LAMBDA 35 UV-Vis spectrophotometer (USA). The UV samples for the silver NPs were prepared by diluting 2 mL of the stock solution of pure silver NPs in 8 mL of water. The stock solutions were prepared by dispersing 5 mg of silver nanoparticles in 5 mL of water with 1 h of sonication (Sonicator® 740 and 740X Ultrasounds). The spectra of the sample in methanol were recorded in the range of 300–800 nm. The powder diffractometric assay (Japan) by Cu of the silver nanoparticles was carried out on a Rigaku Miniflex X-ray diffractometer (Rigaku, Kα (λ = 1.5406 Å) radiation according to the Scherrer formula (L = k ƛ/β × cos2θ). The patterns were recorded in the 2θ range from 10o to 90o with a scanning rate of 0.05 mV/s. The sizes of the prepared silver NPs were measured by using high-resolution transmission electron microscopy (HRTEM), which was performed on JEM-2100F (JEOL, USA) at 120 kV accelerated voltage. The specimens for the transmission electron microscopy (TEM) (JEOL, USA) were prepared by placing a drop of the primary specimen on a copper grid. Then, the sample was dried for 6 h at 80 °C in an oven and observed at high magnification for determination of the nanoparticles’ sizes. The morphology of the NPs was analysed by using both TEM and scanning electron microscope (SEM, JSM-6380LA, Japan) at an operating voltage of 2 kV. The silver NPs' specimens were firstly seated on metal studs, cut by razor blade and dried in vacuum drying oven (Model 1:53 litres’ vacuum oven, Vinci Technologies, France). Afterwards, the specimens where covered with a carbon layer, using a sputter coater (BAL-TEC SCD 005 Sputter Coater, Capovani Brothers Inc., Scotia, NY, USA). To confirm the purity of the product, the chemical structure of the silver nanoparticles was analysed by using energy-dispersive X-ray spectroscopy (Altima IV Make: Regaku, Japan).
R. solani isolates and pathogenicity test
Rhizoctonia solani isolates used in this study were obtained from the Cotton and Fiber Crops Diseases Research Department, Plant Pathology Research Institute, Giza, Egypt. The pathogenicity test of R. solani isolates on the Egyptian cotton cultivars (Gossypium barbadense L.) was performed under greenhouse conditions. All six isolates () from different R. solani anastomosis groups (AGs) used in this study were obtained from cotton and different crops’ roots. Fungal cultures were prepared in 500 mL flasks; each flask contained 100 g barley grains and 50 mL tap water. All flasks were autoclaved for 30 min. Flasks were inoculated with mycelial discs (five discs, 4 mm in diameter) taken from seven-day-old culture. Flasks were incubated at 27 ºC ± 2 ºC for three weeks. Six isolates of R. solani AGs representing several AGs () were used in this study. The batches of autoclaved clay loam soil were infected with inoculums of each isolate at a rate of 2 g/kg soil. The inoculum and soil were mixed, so that the inoculum could be evenly distributed in the soil. Pots containing both infected and uninfected soil ‘as a control’ were cultivated with 10 seeds/pot for each cultivar (Giza 80, Giza 86, Giza 88, Giza 90 and Giza 92). The percentages of the survived plants were calculated according to the following equation:
(1)
(1)
Table 2. Rhizoctonia solani isolates used in this study.
Inhibitory effect of silver nanoparticles against R. solani AGs
The inhibitory effect of the silver nanoparticles was evaluated on two growth media – potato dextrose agar (PDA) (39 g PDA / 1 L distilled water) and Czapek Dox agar (2 g sodium nitrate, 1 g dipotassium phosphate, 0.5 g potassium chloride, 0.5 g magnesium sulphate, 0.01 g ferrous sulphate, 30 g sucrose, 15 g agar / 1 L distilled water) with silver nanoparticles’ concentrations of 0.0, 0.0002, 0.0005, 0.0007, 0.0009, 0.0014 and 0.0019 mol/L. Silver nanoparticles’ solution was added in flasks and poured into petri plates. All plates were incubated at 28 ºC ± 2 ºC. After two days of incubation, a mycelial disc (5 mm) was taken from the edge of seven-day-old fungal cultures (plates containing mycelial of fungi) and placed in the centre of each Petri plate containing silver nanoparticles. Plates without silver served as controls. All plates were incubated at 28 ºC ± 2 ºC for seven days. The inhibition zones (%) were measured after five days according to the following equation:
(2)
(2) where R is the linear growth of the control plate and r is the radial growth of fungal culture on the plate treated with Ag NPs.
Statistical analysis
The experimental plan of this investigation was a randomized complete block and the treatments were conducted in three replicates. The MSTAT-C statistical package was used for the analysis of variance (ANOVA) of the data and correlation analyses. The least significant difference (LSD) was utilized to compare the means of the obtained treatments’ results. Also, the effective doses 50 (ED 50) and ED 90 were calculated by the Probit program.
Results and discussion
UV-Vis spectral analysis
The UV-Vis absorption spectra is an essential method to observe the forming and stabilization of metal nanoparticles in aqueous solutions, as the spectral response of nanoparticles is based on their diameter. The peak plasmon resonance moves to longer wavelengths and broadens as the diameter increases.[Citation23] Therefore, the aqueous bio-reduction of silver ions could be successfully observed by UV-Vis, which is sensitive to numerous factors, such as particle shape, size and particle–particle interaction with the medium.[Citation24] When the synthesized sample was subjected to UV-Vis spectral analysis, the obtained λ max value was 400–500 nm, which was corroborated by using HRTEM. The obtained UV-Vis spectrum is given in
Powder X-ray diffraction
The synthesized Ag NPs crystalline nature has been confirmed by the analysis of the X-ray diffraction (XRD) pattern. Five clear reflections in the diffractogram were observed at 81.13° (222), 76.89° (311), 63.91° (220), 44.13° (200) and 37.50° (111). The XRD pattern in indicated towards the face-centred cubic (fcc) structure of the silver nanoparticles. On the basis of the half-width (Λ) of the (111) reflection in the powder pattern, the average grain size L determined from the broadening of the (111) reflection by the Scherrer formula was roughly 42 nm.
TEM analysis
The morphology and size of the synthesized silver nanoparticles were examined by using TEM. The TEM images () show that the as synthesized NPs had spherical morphology, with a size range of 40–60 nm, in which few nanoparticles were conglomerated.
SEM and energy dispersive analysis X-ray (EDX) analyses
The SEM image of the Ag NPs is shown in A). It can be seen that different shapes of silver nanoparticles were obtained. The SEM micrograph of the silver nanoparticles did not show a uniform surface. The particles were not well-defined in shape and they appeared to be stacked in layers. Furthermore, the elemental structure of the synthesized specimen was also measured by EDX analysis. The EDX profile showed a strong Ag signal and weak carbon peaks, which may be due to the carbon tape utilized for the analysis. The EDX spectrum in B) revealed a clear identification of the elemental component profile of the synthesized silver nanoparticles. The intensive signal at 3 keV highly suggested that silver was the main element that showed an optical absorption in this range, as a result of the surface plasmon resonance.[Citation24,Citation25]
Pathogenicity of R. solani AGs on cotton cultivars
The ANOVA showed that the effects of the cultivars on the plant survival percentage were non-significant (data not shown), whereas the isolate was significant source of difference at P ≤ 0.05. On the other hand, isolate–cultivar interaction was a non-significant source of difference (). Data presented in revealed that the isolate was the most important factor affecting plant mortality. The results in also showed that the isolates resulted in 100% level of mortality in the case of R. solani AG-2-2, R. solani AG-6 and AG-10. It was noticed that there was no common trend correlation of AGs with virulence. These results were in agreement with the results obtained from other studies.[Citation26,Citation27] Similar results have also been reported by a number of authors.[Citation28,Citation29] Khedri et al. [Citation30] suggested that R. solani isolates obtained from various crops may infect and cause mortality of cotton plants.
Table 3. Effect of R. solani isolates on plant survival percentages of cotton cultivars under greenhouse conditions.
Inhibitory effect of silver nanoparticles against R. solani AGs on various culture media
ANOVA of Ag NPs revealed that R. solani AGs (R), concentrations (C) and their interactions (R × C) were highly significant sources of difference in the radial growth suppression of R. solani AGs growing on PDA and Czapek Dox medium (–). The inhibition effect of Ag NPs at different concentrations was examined in PDA and CDA. Generally, higher suppression of fungal radial growth was noticed at a concentration of 0.0019 mol/L (). The highest inhibition was observed on CDA medium against R. solani AG-2-2, R. solani AG-6 and R. solani AG-5 with 77.77%, 73.60% and 71.10% of linear growth inhibition, respectively, when compared to the control. The effective dose 50 (ED50) of Ag NPs was 0.0002 mol/L (R. solani AG-2-2), 0.0004 mol/L (R. solani AG-6) and 0.0002 mol/L (R. solani AG-5). The lowest level of reduction was noticed against R. solani AG-4-HGI CDA treated with a 0.0019 mol/L concentration of Ag NPs (ED50 = 0.0022 mol/L, ED95 = 0.0051 mol/L) ().
Table 4. Analysis of variance of the interaction between R. solani isolate and silver nanoparticles’ concentration on the linear growth of R. solani on PDA and CDA.
Table 5. Relative contribution of the interaction between R. solani isolate and silver nanoparticles’ concentration to the linear growth variation of R. solani grown on PDA and CDA.
Table 6. Analysis of variance of the interaction between silver nanoparticles’ concentration and R. solani isolate on the silver nanoparticles’ efficacy against R. solani isolate.
Table 7. Relative contribution of the interaction between silver nanoparticles' concentration and R. solani isolate to the efficacy variation of silver nanoparticles against R. solani isolate.
Table 8. Effect of different silver nanoparticles concentrations on the linear growth (inhibition (%)) of Rhizoctonia solani isolates grown on CDA medium.
The highest inhibition on PDA was observed against R. solani AG-6 () treated with a 0.0019 mol/L concentration of silver nanoparticles (73.60%), and the estimated ED50 and ED95 obtained by linear regression were 0.00069 and 0.0061 mol/L, respectively, whereas 0.0019 mol/L concentration of Ag NPs resulted in 69.16% inhibition against R. solani AG-2-2 with 0.0007 mol/L (ED50) and 0.0126 mol/L (ED95) on PDA (). On the other hand, the lowest inhibition was noticed against R. solani AG-1 treated with a 0.0019 mol/L concentration of Ag NPs on PDA () and the estimated ED50 and ED95 obtained by linear regression were 0.0019 and 0.0184 mol/L, respectively. The present study suggested that Ag NPs are able to inhibit R. solani AGs; nevertheless, different results were obtained according to the concentration of the silver nanoparticles. Most Ag NPs exhibited a high antifungal effect at a concentration of 0.002 mmol/L. Moreover, the results showed that a higher suppression rate was noticed on CDA, when compared with the obtained suppression rate on PDA. In general, the inhibition increased with the increase of the silver nanoparticles’ concentration. These results are in agreement with those obtained from Park et al. [Citation31]; Kim et al.,[Citation32] Min et al. [Citation33] and Kabir et al. [Citation15] reported that Ag+ and NPs had a significant effect on plant pathogenic fungi, Bipolaris sorokiniana and sclerotium-forming phytopathogenic fungi and Colletotrichum species. This is probably due to the high intensity at which the solution is capable to saturate and adhere to the fungal hyphae and to control the plant diseases. Feng et al. [Citation34] reported that DNA loses its ability to duplicate when the fungal culture was treated with Ag+, which may lead to a deactivated expression of ribosomal subunit proteins and to the synthesis of disabled enzymes and cellular proteins, important for the adenosine triphosphate production.[Citation7,Citation35] Bragg and Rainnie [Citation36] and McDonnell and Russell [Citation37] hypothesized that the silver ions mostly affect the function of the membrane bound enzymes, for example those in the respiratory chain. The results from the in vitro analysis in the present study showed that the silver nanoparticles may be used as antifungal agents by destructing the membrane integrity. Thus, it was concluded that silver nanoparticles have significant antimicrobial activity. There is a need to further investigate the field applications of these findings.
Table 9. Effect of different silver nanoparticles’ concentrations on the linear growth (inhibition (%)) of R. solani isolate grown on PDA medium.
Conclusions
In the present study, the AgNPs were synthesized by a traditional way. The silver nanoparticles were characterized by using UV-Vis spectral analysis, TEM, SEM, XRD and EDX. We also studied the pathogenicity of six R. solani AGs on five cotton cultivars and evaluated the silver nanoparticles’ efficacy against R. solani AGs. The obtained results from the present study presented that silver nanoparticles exhibited reduction in the growth of R. soalni AGs.
Acknowledgment
The authors would like to extend their sincere appreciation to the Deanship of Scientific Research at King Saud University for its funding this Research group No (RG-1436-09).
Disclosure statement
No potential conflict of interest was reported by the authors.
Additional information
Funding
References
- Jain J, Arora S, Rajwade JM, et al. Silver nanoparticles in therapeutics: development of an antimicrobial gel formulation for topical use. Mol Pharm. 2009;6:1388–1401.
- Rauter H, Matyushin V, Alguel Y, et al. Nanotechnology for smart polymer optical devices. Macromol Sym. 2004;217:109–134.
- Jin R. The impacts of nanotechnology on catalysis by precious metal nanoparticles. Nanotechnol Rev. 2012;1:31–56.
- Amanda MS, Braydich-Stolle LK, John JS, et al. Can silver nanoparticles be useful as potential biological labels? Nanotechnol. 2008;19:235104. Available from: http://iopscience.iop.org/0957-4484/19/23/235104
- Zhang H, Peter G, Zhou S, et al. Development of smart nanoparticle aptamer sensing technology. Farad. 2011;149:319–332.
- Francesco B, Giuseppe I, Tomas P, et al. Electronics based on two-dimensional materials. Nat Nanotechnol. 2014;9:768–779.
- Yamanaka M, Hara K, Kudo J. Bactericidal actions of a silver ion solution on Escherichia coli, studied by energy filtering transmission electron microscopy and proteomic analysis. Appl Environ Microbiol. 2005;71:7589–7593.
- Liu Z, Stout JE, Tedesco L, et al. Controlled evaluation of copper-silver ionization in eradicating Legionella pneumophila from a hospital water distribution system. J Infect Dis. 1994;169:919–922.
- Conrad AH, Tramp CR, Long CJ, et al. Ag+ alters cell growth, neurite extension, cardiomyocyte beating, and fertilized egg constriction. Aviat Space Environ Med. 1999;70:1096–1105.
- Hirasawa F, Kawarada Y, Sato M, et al. The effect of silver administration on the biosynthesis and the molecular properties of rat ceruloplasmin. Biochim Biophys Acta. 1997;1336:195–201.
- Morgan TP, Guadagnolo CM, Grosell M, et al. Effects of water hardness on toxicological responses to chronic waterborne silver exposure in early life stages of rainbow trout (Oncorhynchus mykiss). Environ Toxicol Chem. 2005;24:1642–1647.
- Bianchini A, Grosell M, Gregory SM, et al. Acute silver toxicity in aquatic animals is a function of sodium uptake rate. Environ Sci Technol. 2002;36:1763–1766.
- Berger TJ, Spadaro JA, Chapin SE, et al. Electrically generated silver ions: Quantitative effects on bacterial and mammalian cells. Antimicrob Agents Chemother. 1976;9:357–358.
- Jo, YK, Kim BH, Jung G. Antifungal activity of silver ions and nanoparticles on phytopathogenic fungi. Plant Dis. 2009;93:1037–1043.
- Kabir L, Sang WK, Jin HJ, et al. Application of silver nanoparticles for the control of Colletotrichum species in vitro and pepper anthracnose disease in field. Mycobiol. 2011;39:194–199.
- Young-Ki J, William C, Hae-Kwon J, et al. Use of silver nanoparticles for managing Gibberella fujikuroi on rice seedlings. Crop Prot. 2015;74:65–69.
- Young-Ki J, Byung HK, Geunhwa J. Antifungal activity of silver ions and nanoparticles on phytopathogenic fungi. Plant Dis. 2009;10:1037–1043.
- Anna G, Ewa P, Marek K, et al. Effect of nanosilver in wheat seedlings and Fusarium culmorum culture systems. Eur J Plant Pathol. 2015;142:251–261.
- Kannan B, Narayanan M, Hyun HP. Antifungal activity of silver nanoparticles synthesized using turnip leaf extract (Brassica rapa L.) against wood rotting pathogens. Eur J Plant Pathol. 2015;140:185–192.
- Kim SH, Hyeong-Seon L, Deok-Seon R, et al. Antibacterial activity of silver-nanoparticles against Staphylococcus aureus and Escherichia coli. Korean J Microbiol Biotechnol. 2011;39:77–85.
- Pettegrew C, Dong Z, Muhi ZM, et al. Silver nanoparticle synthesis using monosaccharides and their growth inhibitory activity against gram-negative and positive bacteria. ISRN Nanotechnol. 2014;480284. Available from: http://dx.doi.org/10.1155/2014/480284
- Woo KJ, Hye CK, Ki WK, et al. Antibacterial activity and mechanism of action of the silver ion in Staphylococcus aureus and Escherichia coli. Appl Envir Microbiol. 2008;7:2171–2178.
- Shivaraj N, Vandana R, Dattu S. Characterization and biosynthesis of silver nanoparticles using a fungus Aspergillus niger. Int Lett Nat Sci. 2014;15:49–57.
- Khan M, Khan M, Adil SF, et al. Green synthesis of silver nanoparticles mediated by Pulicaria glutinosa extract. Int J Nanomedicine. 2013;8:1507–1516.
- Mallikarjuna K, Sushma NJ, Narasimha G, et al. Phytochemical fabrication and characterization of silver nanoparticles by using pepper leaf broth. Arabian J Chem. 2014;7(6)1099–1103.
- Bacharis C, Gouziotis A, Kalogeropoulou P, et al. Characterization of Rhizoctonia spp. isolates associated with damping-off disease in cotton and tobacco seedlings in Greece. Plant Dis. 2010;94:1314–1322.
- Rani VD, Reddy PN, Devi GU. Banded leaf and sheath blight of maize incited by Rhizoctonia solani f.sp sasakii and its management – a review. Int J Appl Biol Pharma Tech. 2013;4:52–56.
- Monga D, Sheo-Raj RS. Cultural and pathogenic variations in the isolates of Rhizoctonia species causing root rot of cotton. Indian Phytopathol. 1994;47:403–407.
- Aqil T, Batson EW. Evaluation of radical assay for screening cotton cultivars for resistance to the pathogens of seedling disease complex. Pakistan J Phytopathol. 1999;11:11–16.
- Khedri Z, Asghar H, Hossein A. Pathogenecity of different isolates of Rhizoctonia solani on commonly cultivated cotton varieties in Iran. Intl J Agri Crop Sci. 2014;7:537–542.
- Park HJ, Kim SH, Kim HJ, et al. A new composition of nanosized silica-silver for control of various plant diseases. Plant Pathol. 2006;22:295–302.
- Kim SW, Kim KS, Lamsal K, et al. An in vitro study of the antifungal effect of silver nanoparticles on oak wilt pathogen Raffaelea sp. J Microbiol Biotechnol. 2009;19:760–764.
- Min JS, Kim KS, Kim SW, et al. Effects of colloidal silver nanoparticles on sclerotium-forming phytopathogenic fungi. Plant Pathol J. 2009;25:376–80.
- Feng QL, Wu J, Chen GQ, et al. A mechanistic study of the antibacterial effect of silver ions on Escherichia coli and Staphylococcus aureus. Biomed Mater Res. 2000;52:662–668.
- Sang WK, Jin HJ, Kabir L, et al. Antifungal effects of silver nanoparticles (Ag NPs) against various plant pathogenic fungi. Mycobiol. 2012;40:415–427.
- Bragg PD, Rainnie DJ. The effect of silver ions on the respiratory chains of Escherichia coli. Can J Microbiol. 1974;20:883–889.
- McDonnell G, Russell AD. Antiseptics and disinfectants: activity, action, and resistance. Clin Microbiol Rev. 1999;12:147–79.