ABSTRACT
The effects of six non-dissolved redox mediators (RM) immobilized in cellulose acetate beads on enhancing Cr(VI) reduction by Mangrovibacter plantisponsor CR1 were investigated. In addition, the voltammetric behaviours and electron transfer capacities of the redox mediators were examined using electrochemical methods. Compared to the control beads, the Cr(VI) bioreduction rate with 1-chloroanthraquinone cellulose acetate beads (1-CAQ/CA beads) was increased up to 4.5-fold, which was mainly attributed to enhanced electron transfer by 1-CAQ. The redox mediators also improved the oxidation–reduction potential values of the Cr(VI) bioreduction processes, which might assist in Cr(VI) bioreduction. The role of the redox mediators was discussed based on the cyclic voltammetric characteristics (E0') of the redox mediators and the electrochemical impedance spectroscopy characteristics (Rct) of the RM/CA beads. A linear correlation was found for the reaction constant k and the 1-CAQ concentration (C1-CAQ), which was k = 1.5674 C1-CAQ + 4.8506 (R2 = 0.9683). The Cr(VI) bioreduction was affected by temperature, and the optimum pH for the Cr(VI) bioreduction was 6.5. The results of repeated-batch operations showed that 1-CAQ/CA beads exhibited good stability and persistence. This study contributes to a better understanding of the effects of the redox mediator on Cr(VI) bioreduction process and demonstrates its promising potential for environmental bioremediation applications.
Introduction
Chromium (Cr), one of the most widespread elements on earth, is an important industrial material that is used extensively in electroplating, dye and pigment manufacturing, wood preservation, leather tanning, alloy production and other applications.[Citation1,Citation2] Large quantities of industry effluents containing chromium are often released into natural waters without proper treatment, resulting in severe environmental pollution.[Citation3] Chromium exists in different valence states ranging from −2 to +6, and only hexavalent chromium [Cr(VI)] and trivalent chromium [Cr(III)] are stable oxidation states in the natural environment. Cr(VI) and Cr(III) differ widely in physicochemical behaviour, mobility and toxicity.[Citation4] Cr(VI) is approximately 100 times more toxic and 1000 times more mutagenic than Cr(III) due to its solubility across nearly the entire pH range and its greater mobility.[Citation5] The European Union recommends total chromium limits of 0.05 and 0.1 mg/L for potable and industrial wastewater, respectively,[Citation6] and the United States Environmental Protection Agency has set a maximum contaminant level of 0.05 mg/L for Cr(VI) in domestic water.[Citation1] Thus, the transformation of Cr(VI) to Cr(III) is an attractive and useful process for the remediation of Cr(VI) pollution.
Conventional physicochemical treatment processes for Cr(VI) detoxification generally involve the reduction of Cr(VI) to Cr(III) by a strong reducing agent and precipitation of Cr(III) by adjusting the pH to a neutral range. However, these processes require large amounts of chemicals and energy and they produce secondary wastes. Biological reduction of Cr(VI) to Cr(III) is an ideal alternative because it is economical, safe and sustainable. A Cr(VI)-reducing ability has been demonstrated by many aerobic and anaerobic bacteria, such as Bacillus megaterium,[Citation7] Desulfovibrio vulgaris,[Citation8] Thermus scotoductus,[Citation9] Ochrobactrum spp.,[Citation10] Shewanella oneidensis,[Citation11] Escherichia coli [Citation12] and Cellulomonas spp.[Citation2] Nevertheless, the biological reduction of Cr(VI) occurs slowly due to toxic effects on bacteria or electron transfer limitations, and bioreduction processes invariably require several days or even weeks.
It has been shown that redox mediators, such as humus substances and their quinoid analogues, could enhance the biotransformation of azo dyes,[Citation13] nitroaromatics,[Citation14,Citation15] aliphatic and aromatic poly-halogenated compounds,[Citation16–18] nitrates,[Citation19] metals [Citation3,Citation20] and metalloids.[Citation21] Redox mediators could decrease electron transfer limitations, accelerating the biotransformation of these contaminations. Liu et al. [Citation3] evaluated the catalysis effect of certain quinones on Cr(VI) bioreduction, and the result showed that the average reduction rate of Cr(VI) was improved by nearly 3.3-fold in the presence of 0.2 mmol/L 2-hydroxy-1,4-naphthoquinone (lawsone). However, to maintain the increased conversion rates, the dissolved redox mediators had to be provided continuously, which was uneconomical and caused recontamination problems. Therefore, applications of non-dissolved redox mediators and effective methods to immobilize redox mediators have lately been explored. Redox mediators have been immobilized by entrapment in polyvinyl alcohol-H3BO3 and agar,[Citation13] by covalent binding on ceramsite [Citation22] and polyurethane foam,[Citation15,Citation23] by adsorption on metal oxide nanoparticles,[Citation24] by electrostatic attraction on the surface of anion-exchange resins [Citation25] and by electropolymerization on activated carbon felt.[Citation26,Citation27] Previously, Li et al. [Citation28] reported that non-dissolved redox mediators immobilized in cellulose acetate could enhance the nitrite denitrification efficiency, but little is known regarding how to affect the Cr(VI) bioreduction of non-dissolved redox mediators.
In this work, the characteristics of Cr(VI) bioreduction by Mangrovibacter plantisponsor CR1 with non-dissolved redox mediators immobilized in cellulose acetate beads were investigated. The electron transfer properties of the redox mediators were evaluated based on cyclic voltammetric characteristics and electrochemical impedance spectroscopy (EIS) characteristics. This work will support a better understanding of the effects of the redox mediators on Cr(VI) bioreduction process and improve practical bioremediation processes.
Materials and methods
Chemicals
Anthraquinone (AQ), 1-chloroanthraquinone (1-CAQ), 2-chloroanthraquinone (2-CAQ), 1,5-dichloroanthraquinone (1,5-DCAQ), 1,8-dichloroanthraquinone (1,8-DCAQ) and 1,4,5,8-tetrachloroanthraquinone (1,4,5,8-TCAQ) were of analytical grade and supplied by Sigma–Aldrich. The chemical structures of these redox mediators are shown in the Supplemental data (Figure S1). Cellulose acetate (CA) was purchased from Chemical Reagent Corporation (Shanghai, China); triolein (98% purity) was purchased from Jinlong Chemical Reagent Corporation (Beijing, China); and Tween 80 (A.R.), acetone (A.R.), Mg(ClO4)2 (A.R.) and liquid olefin were purchased from Chemical Reagent Corporation (Beijing, China). Other chemical reagents were of analytical grade and purchased from Xiandai Ltd. (Shijiazhuang, China).
Bacterial strains and culture conditions
The Cr(VI)-reducing bacterial strain CR1 was isolated from tanning sludge collected from a tannery in Xinji City (Hebei, China). It was identified as M. plantisponsor (GenBank accession no. KF530843) based on its biochemical properties and 16S rRNA gene sequence homology analysis.[Citation29] Cr(VI) bioreduction was performed in mineral medium containing the following: 1.0 g/L NH4Cl, 1.0 g/L NaHCO3, 0.2 g/L K2HPO4, 0.2 g/L MgSO4·7H2O, 1.0 g/L NaCl and 2.0 g/L glucose with 2 mL of a trace element solution. The trace element solution included the following: 15 g/L ethylene diamine tetraacetic acid (EDTA), 0.43 g/L ZnSO4·7H2O, 0.24 g/L CoCl2·6H2O, 0.99 g/L MnCl2·4H2O, 0.25 g/L CuSO4·5H2O, 0.22 g/L NaMoO4·2H2O, 0.19 g/L NiCl2·6H2O, 0.21 g/L NaSeO4·10H2O, 0.014 g/L H3BO4, and 0.05 g/L NaWO4·2H2O. Strain CR1 was cultivated aerobically in mineral medium at 30 °C until the optical density at 600 nm (OD600) reached 0.75. A stock solution of Cr(VI) was prepared by dissolving K2CrO4 in deionized water. A certain volume of Cr(VI) from the stock solution was taken, sterilized and added to the medium to obtain the desired Cr(VI) concentration. The biomass concentration of strain CR1 was 0.31 g of dry weight per litre in all of the experiments. The pH was adjusted with 0.1 mol/L NaOH or 0.1 mol/L HCl.
Immobilization of redox mediators
To prepare immobilized redox mediators (RM), 7.0 g of cellulose acetate was dissolved in 55 mL of acetone, and ultrasonication (Kunshan Co., KQ218, China) for 20 min with a fixed power of 200 W was used to promote dissolution. Then, 5 mL of deionized water was added to the mixture, followed by 1.5 g of Mg(ClO4)2 and 0.5 mL of triolein. Subsequently, different redox mediators were added in different amounts. The mixtures were kept at 35 °C for 48 h and stirred to achieve uniform admixture. Thus, viscous syrups were carefully prepared and homogeneous phases were obtained. Each viscous syrup was added drop by drop to a glass tube containing the liquid olefin (approximately 2/3 of the volume). The cellulose acetate solution and liquid olefin were maintained at room temperature. During the process of dripping the viscous syrup through the air to the column of liquid olefin, a spherical immobilization bead was formed by the action of surface tension and was collected at the bottom of the glass tube. Finally, the cellulose acetate beads with redox mediators (RM/CA beads) were washed with deionized water to remove all soluble impurities. The redox mediator concentration was calculated by the mass ratio of the added redox mediator and the number of beads. In addition, cellulose acetate beads without redox mediators (i.e. control beads) were prepared by the same procedures.
Cr(VI) bioreduction with redox mediators
Bioreduction assays were performed in 250 mL serum glass bottles with the mineral medium described above. The atmosphere in the headspace of the bottles was changed with a mixture of N2/CO2 (80%/20%) in order to create anaerobic conditions. Cr(VI) bioreduction was performed with different non-dissolved redox mediators to obtain the optimal redox mediator. Cr(VI) bioreduction with the optimum redox mediator was conducted under different redox mediator concentrations (0–0.072 mol/L), temperatures (20 °C–50 °C) and initial pH values (4.0–9.0) to optimize the parameters simultaneously. Six repeated batch operations were also studied to investigate the stability and persistence of enhancement of the Cr(VI) bioreduction. At pre-determined time intervals, 2 mL aliquots were withdrawn for reduction experiments at set time intervals. All of the experiments were performed in triplicate.
Analytical methods
Bacterial growth was monitored by measuring OD600 using an ultraviolet/visible (UV/vis) spectrophotometer (Tianmei Co., UV2600, China). Cr(VI) concentration was analysed according to the standard colorimetric method of the American Public Health Association involving a reaction with 1,5-diphenylcarbazide and measured via the same UV/vis spectrophotometer at 540 nm.[Citation30] The pH was measured using a digital pH meter (Mettler Toldedo Co., Delta-320, China). Electrochemical measurements were performed using the reported three-electrode configuration [Citation13] with a Voltalab Powersuite (Lanlike Co., LK98B, China).
EIS was used to explore the roles of redox mediators in Cr(VI) bioreduction. A platinum wire was used to string four beads along their radical direction. Due to good elasticity and toughness, the beads tightly contacted the platinum wire to produce a sufficient contact area for good conductivity. The bead-platinum composite electrode was used as the working electrode for the EIS measurement. EIS measurements were performed using an electrochemical workstation (CHI 660E, Chenhua Co., China) with a saturated calomel electrode as a reference electrode and a Pt counter electrode in a KCl electrolyte with 5 mmol/L Fe(CN)63-/4−. EIS was measured at the open circuit voltage over a frequency range of 100 kHz–0.01 Hz with a potential amplitude of 5 mV. The electric resistance of the beads was then evaluated according to the Nyquist plots of the impedance spectra.
Results and discussion
Effects of different non-dissolved redox mediators on Cr(VI) bioreduction
Without bacteria, the same volume of control beads and RM/CA beads were examined for their adsorption of Cr(VI). Control beads and RM/CA beads caused less than 3% adsorption of Cr(VI) within 48 h (data not shown) under a fixed initial Cr(VI) concentration (52 mg/L), which suggested that physicochemical processes, i.e. absorption or chemical reduction, were not responsible for the Cr(VI) bioreduction. Cr(VI) with the control beads was reduced by only 16.2% by strain CR1 within 10 h, and Cr(VI) was reduced by 90.4%, 76.1%, 69.8%, 54.7% and 49.6% with 0.036 mol/L 1-CAQ, 2-CAQ, 1,5-DCAQ, AQ and 1,8-DCAQ within 10 h, respectively (). The highest Cr(VI) reduction rate was obtained in the 1-CAQ/CA beads system, which showed an increase of nearly 4.5-fold in comparison with the control bead system. The accelerating effect of 1,4,5,8-TCAQ was not remarkable, and only 20.3% Cr(VI) was reduced. The acceleration order during the bioreduction process was 1-CAQ > 2-CAQ > 1,5-DCAQ > AQ > 1,8-DCAQ > 1,4,5,8-TCAQ > control. As the number of –Cl substituents increased, the acceleration effect decreased. Meanwhile, the position of the –Cl substituent influenced the acceleration effect, except for AQ. Previous studies have reported that the acceleration orders of redox mediators during the process of nitrite denitrification [Citation28] and azo dye decolourization [Citation19] were 1,4,5,8-TCAQ > 1,5-DCAQ > 1,8-DCAQ > AQ and 1,5-DCAQ > 1,8-DCAQ > AQ > 1,4,5,8-TCAQ, respectively. Compared to the reported results, the difference in acceleration order was due to the different biological reduction processes. The accelerating effect of the six quinone compounds was due to the presence and the position of the electrophilic functional groups in their structures, and the steric effects of the different targeted pollutants may also affect the acceleration efficiency.
Figure 1. Effects and changes in the oxidation–reduction potential (ORP) of different non-dissolved redox mediators on Cr(VI) reduction by strain CR1 under anaerobic conditions.
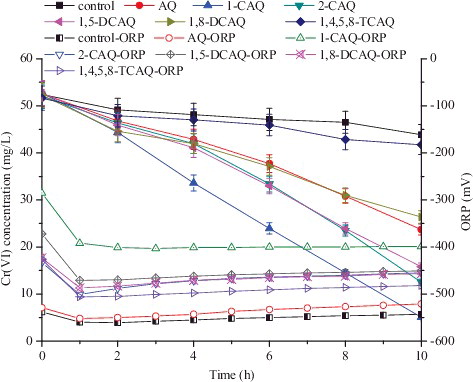
Different redox couples co-exist in different systems, and all of the redox couples contribute to the oxidation–reduction potential values (ORP) of the system. The ORP features of the Cr(VI) bioreduction processes may indirectly reflect specific chemical, biochemical and biological changes over time. Therefore, the ORP changes in the Cr(VI) bioreduction processes with different non-dissolved redox mediators were monitored. Throughout the bioreduction processes, all of the ORP values were negative. At the beginning of the bioreduction processes, a rapid drop in ORP occurred in both the control bead and the RM/CA bead systems (). In the control, 1-CAQ/CA, 2-CAQ/CA, 1,5-DCAQ/CA, AQ/CA, 1,8-DCAQ/CA and 1,4,5,8-TCAQ/CA systems, the ORP values stabilized at approximately −550, −400, −470, −460, −540, −470, and −495 mV, respectively (). The results showed that the redox mediators could improve the ORP values of the Cr(VI) bioreduction processes, and the optimal ORP values were approximately 150 mV higher with 1-CAQ than the controls. Previous research also demonstrated that low ORP values were required for Cr(VI) bioreduction.[Citation31] The results of this experiment indicated that higher ORP values (−400 mV with 1-CAQ/CA beads vs. −550 mV with control beads) were associated with faster reduction rates. The results vary due to the differences in the bioreduction systems, which are related to different bacterial species and their characteristics.
Effects of different 1-CAQ concentrations on Cr(VI) bioreduction
Mediated Cr(VI) bioreduction was studied with different initial 1-CAQ concentrations (0–0.072 mol/L). The bioreduction process with 1-CAQ followed a modified pseudo-zero order model, which was described by k = dCCr(VI)/dt (A). The reaction constant k (mg Cr(VI) g−1 dry cell weight h−1) was 3.87, 6.60, 9.45, 11.45, 12.54, 14.11 and 15.37 at 1-CAQ concentrations of 0, 0.012, 0.024, 0.036, 0.048, 0.060 and 0.072 mol/L, respectively. The linear correlation for the k value and the 1-CAQ concentration (0–0.072 mol/L) was k = 1.5674 C1-CAQ + 4.8506 (R2 = 0.9683) (B). The average reduction rates k increased with increasing concentrations of the redox mediator. Furthermore, the mediator gradients showed that the effect is especially strong at low mediator concentrations. The acceleration gradient levels were weak at higher concentration, possibly due to the lack of a sufficient electron source.[Citation32] Similar linear relationships were obtained in previous studies on Cr(VI) bioreduction with dissolved redox mediators.[Citation12]
Effects of temperature and pH on Cr(VI) bioreduction
As shown in (A), the reduction efficiency increased with rising the temperature from 20 °C to 50 °C, which was quite similar to the result observed in the case of E. coli BL21.[Citation12] The reduction rate constant k' (mg Cr(VI) g−1 dry cell weight h−1) was 7.44, 10.24, 12.01, 15.04 and 17.27 at 20 °C, 30 °C, 35 °C, 40 °C and 50 °C, respectively. The pH notably affected the chromium form in solution as well as the chromium binding sites on the cell surface. Therefore, experiments were performed to monitor the optimum pH. As shown in (B), the optimum pH for Cr(VI) reduction by CR1 was 6.5. The Cr(VI) bioreduction activity was nearly inhibited at pH 4.0, and it decreased gradually with increasing pH from 6.5 to 9.0. Previous research demonstrated that the standard redox potential for the Cr(VI)/Cr(III) couple decreased with increasing pH, which would affect the Cr(VI) bioreduction.[Citation33]
Repeat batch tests
The anaerobic bioreduction of Cr(VI) with 1-CAQ during repeated experiments was investigated. The results presented in showed that the bioreduction efficiency decreased by less than 5%, and the redox mediator immobilized in cellulose acetate beads exhibited good stability and persistence under the applied experimental conditions after six cycles. Compared to the calcium alginate beads with immobilized redox mediators, the RM/CA beads exhibited good reusability (data not shown), which might significantly reduce the operation costs in industrial applications.
Electrochemical performances of non-dissolved redox mediators
EIS analysis was conducted to evaluate the charge-transfer resistance of the redox mediators used in this study. A typical Nyquist plot for the three electrodes is shown in . The EIS Nyquist plots consist of a semicircular portion (high frequency region) and a subsequent straight line (low frequency region). The diameter of the former corresponds to the charge-transfer resistance (Rct) at an electrode/electrolyte interface, which usually represents the resistance of electrochemical reactions on the electrode, and a smaller Rct value indicates a faster rate of the electron transfer.[Citation34] The order of the Rct value was control > 1,8-DCAQ > AQ > 1,5-DCAQ > 1-CAQ > 1,4,5,8-TCAQ > 2-CAQ. The results showed that the Rct of the control beads was higher than the Rct of the RM/CA beads, and redox mediators could enhance the electron transfer process.
The effect of redox mediators on the biological reduction process may be directly related to the standard redox potential (E0'), which should ideally be between the values of the two eventual half reactions, the reduction of Cr(VI) and the oxidation of a primary electron donor.[Citation35] Therefore, the cyclic voltammetry characteristics of the six redox mediators were determined () at a 50 mV/s scan rate and a redox mediator concentration of 3.6 mmol/L. The six redox mediators displayed a reversible oxidation peak in the potential range of −0.120 to −0.226 V vs. Hg–Hg2Cl2(s) and a reversible reduction peak in the range of −0.224 to −0.271 V vs. Hg–Hg2Cl2(s). The E0' values for the six redox mediators are in the range of −0.175 to −0.249 V vs. Hg–Hg2Cl2(s), which are lower than the values for Cr(VI)/Cr(III).[Citation21] The redox mediator 1,4,5,8-TCAQ had the lowest reduction peaks (Ea) due to its four chloro substituents. The E0' order of the redox mediators was 2-CAQ ≥ 1,5-DCAQ ≥ 1-CAQ > 1,8-DCAQ > AQ > 1,4,5,8-TCAQ. Li et al. [Citation28] reported that the acceleration effect during the nitrite denitrification process was related to E0' of the redox mediators. However, the acceleration effect of the redox mediators obtained in this study (see ‘Effects of different non-dissolved redox mediators on Cr(VI) bioreduction’) did not agree with E0'. This result indicated that the acceleration effect of the non-dissolved redox mediators was determined by many factors, including the EIS characteristics (i.e. Rct) and cyclic voltammetry characteristics (i.e. E0') of the redox mediators. Further work is needed to elucidate the relationship between the electrochemical performances of the redox mediators and the acceleration effect of the redox mediators during the Cr(VI) bioreduction process.
Table 1. Cyclic voltammetric characteristic values of six redox mediators.
Conclusions
The characteristics of Cr(VI) bioreduction by M. plantisponsor CR1 with non-dissolved redox mediators immobilized in cellulose acetate beads were investigated. The non-dissolved redox mediators were found to enhance the reduction of Cr(VI) to Cr(III) and to improve the ORP values of the Cr(VI) bioreduction processes, which might contribute to accelerating the Cr(VI) bioreduction. There was a linear correlation between the reaction constant k value and C1-CAQ. The Cr(VI) bioreduction was affected by temperature and initial pH, and the RM/CA beads exhibited better stability and persistence performance. The electrochemical performances of the redox mediators showed that the role of the redox mediators is to accelerate the electron transfer of Cr(VI) bioreduction. This work will extend the practical application of redox mediators in accelerating the biotransformation of recalcitrant pollutants.
TBEQ-2015-0253-Online_supplementary_appendix.pdf
Download PDF (88.1 KB)Supplemental data for this article can be accessed at http://dx.doi.org/10.1080/13102818.2015.1134277.
Disclosure statement
No potential conflict of interest was reported by the authors.
Additional information
Funding
References
- Murugavelh S, Mohanty K. Bioreduction of hexavalent chromium by free cells and cell free extracts of Halomonas sp. Chem Eng J. 2012;203:415–422.
- Field EK, Gerlach R, Viamajala S, et al. Hexavalent chromium reduction by Cellulomonas sp. strain ES6: the influence of carbon source, iron minerals, and electron shuttling compounds. Biodegradation. 2013;24(3):437–450.
- Liu G, Yang H, Wang J, et al. Enhanced chromate reduction by resting Escherichia coli cells in the presence of quinone redox mediators. Bioresour Technol. 2010;101:8127–8131.
- Yan F, Wu C, Cheng Y, et al. Carbon nanotubes promote Cr(VI) reduction by alginate-immobilized Shewanella oneidensis MR-1. Biochem Eng J. 2013;77:183–189.
- Song H, Liu Y, Xu W, et al. Simultaneous Cr(VI) reduction and phenol degradation in pure cultures of Pseudomonas aeruginosa CCTCC AB91095. Bioresour Technol. 2009;100:5079–5084.
- Garavaglia L, Cerdeira SB, Vullo DL. Chromium (VI) biotransformation by β- and γ- Proteobacteria from natural polluted environments: a combined biological and chemical treatment for industrial wastes. J Hazard Mater. 2010;175:104–110.
- Cheung KH, Lai HY, Gu JD. Membrane-associated hexavalent chromium reductase of Bacillus megatelium TKW3 with induced expression. J Microbiol Biotechnol. 2006;16(6):855–862.
- Goulhen F, Gloter A, Guyot F, et al. Cr(VI) detoxification by Desulfovibrio vulgaris strain Hildenborough: microbe-metal interactions studies. Appl Microbiol Biotechnol. 2006;71(6):892–897.
- Opperman DJ, Van Heerden E. A membrane-associated protein with Cr(VI)-reducing activity from Thermus scotoductus SA-01. FEMS Microbiol Lett. 2008;280(2):210–218.
- He Z, Gao F, Sha T, et al. Isolation and characterization of a Cr(VI)-reduction Ochrobactrum sp. strain CSCr-3 from chromium landfill. J Hazard Mater. 2009;163:869–873.
- Belchik SM, Kennedy DW, Dohnalkova AC, et al. Extracellular reduction of hexavalent chromium by cytochromes MtrC and OmcA of Shewanella oneidensis MR-1. Appl Environ Microbiol. 2011;77(12):4035–4041.
- Guo J, Lian J, Xu Z, et al. Reduction of Cr(VI) by Escherichia coli BL21 in the presence of redox mediators. Bioresour Technol. 2012;123:713–716.
- Guo J, Zhou J, Wang D, et al. Biocatalyst effects of immobilized anthraquinone on the anaerobic reduction of azo dyes by the salt-tolerant bacteria. Water Res. 2007;41(2):426–432.
- Hakala JA, Chin YP, Weber EJ. Influence of dissolved organic matter and Fe(II) on the abiotic reduction of pentachloronitrobenzene. Environ Sci Technol. 2007;41:7337–7342.
- Wang J, Lu H, Zhou Y, et al. Enhanced biotransformation of nitrobenzene by the synergies of Shewanella species and mediator-functionalized polyurethane foam. J Hazard Mater. 2013;252–253:227–232.
- Cutter L, Sowers KR, May HD. Microbial dechlorination of 2,3,5,6-tetrachlorobiphenyl under anaerobic conditions in the absence of soil or sediment. Appl Environ Microbiol. 1998;64:2966–2969.
- O'Loughlin EJ, Burris DR, Delcomyn CA. Reductive dechlorination of trichloroethene mediated by humic-metal complexes. Environ Sci Technol. 1999;33(7):1145–1147.
- Kappler A, Haderlein SB. Natural organic matter as reductant for chlorinated aliphatic pollutants. Environ Sci Technol. 2003;37:2714–2719.
- Guo J, Liu H, Qu J, et al. The structure activity relationship of non-dissolved redox mediators during azo dye bio-decolorization processes. Bioresour Technol. 2012;112:350–354.
- Wang X, Liu G, Zhou J, et al. Quinone-mediated reduction of selenite and tellurite by Escherichia coli. Bioresour Technol. 2011;102(3):3268–3271.
- Fredrickson JK, Kostandarithes HM, Li SW, et al. Reduction of Fe(III), Cr(VI), U(VI), and Tc(VII) by Deinococcus radiodurans R1. Appl Environ Microbiol. 2000;66(5):2006–2011.
- Yuan S, Lu H, Wang J, et al. Enhanced bio-decolorization of azo dyes by quinone-functionalized ceramsites under saline conditions. Process Biochem. 2012;47(2):312–318.
- Lv H, Zhou J, Wang J, et al. Enhanced biodecolorization of azo dyes by anthraquinone-2-sulfonate immobilized covalently in polyurethane foam. Bioresour Technol. 2010;101(18):7185–7188.
- Alvarez LH, Perez-Cruz MA, Rangel-Mendez JR, et al. Immobilized redox mediator on metal-oxides nanoparticles and its catalytic effect in a reductive decolorization process. J Hazard Mater. 2010;184(1–3):268–272.
- Cervantes FJ, Gonzalez-Estrella J, Márquez A, et al. Immobilized humic substances on an anion exchange resin and their role on the redox biotransformation of contaminants. Bioresour Technol. 2011;102(2):2097–2100.
- Wang J, Li L, Zhou J, et al. Enhanced biodecolorization of azo dyes by electropolymerization-immobilized redox mediator. J Hazard Mater. 2009;168(2–3):1098–1104.
- Liu H, Guo J, Qu J, et al. Biological catalyzed denitrification by a functional electropolymerization biocarrier modified by redox mediator. Bioresour Technol. 2012;107:144–150.
- Li H, Guo J, Lian J, et al. Study the biocatalyzing effect and mechanism of cellulose acetate immobilized redox mediators technology (CE-RM) on nitrite denitrification. Biodegradation. 2014;25(3):395–404.
- Lian J, Li Z, Xu Z, et al. Isolation and Cr(VI) reduction characteristics of quinone respiration in Mangrovibacter plantisponsor strain CR1. Biotechnol Appl Biochem. Forthcoming 2015. doi:10.1002/bab.1395.
- APHA. Standard methods for the examination of water and wastewater. 21st ed. Washington (DC): American Public Health Association/American Water Works Association/Water Environment Federation; 2005.
- Llovera S, Bonet R, Simon-Pujol M, et al. Chromate reduction by resting cells of Agrobacterium radiobacter EPS-916. Appl Environ Microbiol. 1993;59:3516–3518.
- Liu G, Zhou J, Wang J, et al. Acceleration of azo dye decolorization by using quinone reductase activity of azoreductase and quinone redox mediator. Bioresour Technol. 2009;100:2791–2795.
- Deiana S, Premoli A, Senette C. Reduction of Cr(VI) by caffeic acid. Chemosphere. 2007;67(10):1919–1926.
- Bard AJ, Faulkner LR. Electrochemical methods: fundamentals and applications. 2nd ed. New York (NY): Wiley; 2001.
- Van der Zee FP, Cervantes Francisco J. Impact and application of electron shuttles on the redox (bio)transformation of contaminants: a review. Biotechnol Advances. 2009;27(3):256–277.