ABSTRACT
Boron (B) deficiency inhibits and disturbs root growth and development by interacting with auxin (indole-3-acetic acid, IAA). However, the underlying mechanism of this interaction is still poorly understood. This study found that plants in a long-term boron deprivation treatment (∼0.25 µg L−1) had inhibited elongation of trifoliate orange roots, enlarged root tips and severe necrosis as well as a significant decrease in soluble boron and IAA content in seedling root tips. The results of a short-term boron deprivation treatment showed that a significant decrease in soluble boron and IAA content occurred after 3 hours of treatment (HOT) and 1 day of treatment (DOT), respectively. Moreover, the expression of IAA synthetic genes (TAA1, TAR2, YUC3 and YUC8) was strongly induced as early as 3 HOT and was then significantly reduced. The expression of rootward IAA transport genes (AUX1, PIN1 and PIN4) decreased significantly in the boron deprivation treatment, but the expression levels of shootward IAA transport genes (LAX1, ABCB1 and PIN3) were significantly increased. Taken together, the increase in IAA content before 1 DOT may be due to increased IAA synthesis caused by the induction of TAA1, TAR2, YUC3 and YUC8 expression; the subsequent decrease and the significantly lower final IAA content compared to the +B treatment (0.25 mg L−1 B) may be due to the reduced expression of IAA synthetic genes and rootward IAA transport genes and the increased expression of shootward IAA transport genes.
Abbreviations: DAT: days after treatment; DOT: day of treatment; FW: fresh weight; HOT: hour of treatment; isB: insoluble boron; LC: liquid chromatography; LSD: least-significant difference; MS: mass spectrometry; PAT: polar auxin transport; qRT-PCR: quantitative real-time polymerase chain reaction; sB: soluble boron
Introduction
Boron (B) is an essential microelement for plant growth and development because of its ability to cross-link the pectin rhamnogalacturonan II in primary cell walls.[Citation1] Boron deficiency alters the physical properties (extensibility and plasticity) of cell walls, and therefore impairs the cell wall structure and function.[Citation2–4] Boron deficiency has become a serious problem in the cultivation of many agricultural crops, such as wheat,[Citation5] maize [Citation6] and citrus.[Citation7] Boron deficiency has severe negative effects on plants, including induction of male sterility in wheat, rice and maize,[Citation5,Citation6] the reduction of root biomass and nodules in legumes [Citation8] and the deformation of new leaves and hyperplastic petioles in castor beans.[Citation9] In citrus, boron deficiency causes leaf senescence, decreases the tree vigour and reduces the fruit yield and quality.[Citation10,Citation11] It also causes corky split veins to occur in mature leaves.[Citation7,Citation12]
Roots are critical for absorption, the transport of water and mineral nutrients such as boron, the synthesis of metabolites such as auxin, etc. Previous studies show that boron deficiency severely inhibits root growth and development.[Citation2,Citation13–16] Because indole-3-acetic acid (IAA) plays an important role in root elongation, lateral-root initiation and emergence and root mitosis,[Citation17] the symptoms of boron deficiency may be related to an alteration in IAA metabolism.[Citation18,Citation19]
IAA is mainly synthesized in shoot and root tips, young leaves, developing seeds and other actively growing tissues.[Citation20] The pathway for IAA biosynthesis has two steps: the conversion of tryptophan to indole-3-pyruvate catalyzed by the TAA family of amino transferases, and the conversion of IPA to IAA catalyzed by the YUC family of flavin monooxygenases.[Citation21] The action of IAA depends on its distribution within plant tissues; except for local biosynthesis and release from inactive precursors, IAA distribution is mainly determined by IAA-directional transport between cells.[Citation22,Citation23] It is well established that polar auxin transport (PAT) within plant tissues is regulated by auxin influx and efflux carriers, such as AUXIN RESISTANT1/LIKE AUX1 (AUX1/LAX) uptake permeases, adenosine triphosphate (ATP)-binding cassette subfamily boron (ABCB) transporters and PIN-FORMED (PIN) carrier proteins.[Citation24,Citation25] In plant roots, there are two PAT pathways: the first one moves IAA toward the root tip through the central cylinder cells with the aid of AUX1, ABCB19 and PIN1, PIN4 and PIN7; the second one moves IAA from the root tip toward the shoot through the outer layers of root cells with the aid of AUX1, ABCB1, PIN2 and PIN3.[Citation25–27]
Trifoliate orange is the main rootstock in the citrus-producing region of China.[Citation10] However, its primary and lateral root elongation is more sensitive to boron deficiency than other citrus rootstocks.[Citation15,Citation16] Although a relationship between boron deficiency and IAA metabolism in citrus has been hypothesized [Citation7,Citation15,Citation16] and was discovered in squash,[Citation2] sunflower,[Citation28] pea [Citation29] and Arabidopsis thaliana,[Citation19] our knowledge about this relationship is still rudimentary. In this study, we comprehensively analyzed the transcript profiles of the genes involved in IAA synthesis and transport after exposure to boron-deficient conditions. We also assessed the response of root morphology and boron and IAA root content to boron deficiency. The aim of this work was to investigate the possible underlying mechanisms of root malformation resulting from boron deficiency.
Materials and methods
Plant material and cultivation
Trifoliate orange seeds [Poncirus trifoliata (L.) Raf.] were obtained from the National Citrus Breeding Center at Huazhong Agricultural University, Hubei Province, China. The culture method and the conditions for seed germination and seedling growth were as described by Zhou et al. [Citation16]. When the seedlings had—five to seven leaves, uniform and healthy seedlings were selected and were transferred to the treatment solutions: full strength Hoagland's nutrient solution containing either ∼0.25 µg L−1 B (−B), or 0.25 mg L−1 B (+B). Each treatment was replicated three times, with over 30 seedlings in each repetition. After eight weeks of cultivation, when boron deficiency symptoms were visible, roots and root tips were collected for measurement of boron and IAA content and for morphological and anatomical analysis.
A short-term boron treatment experiment investigating the response of IAA-related genes to boron deficiency was also conducted. Approximately 300 uniform and healthy seedlings were placed in −B and +B culturing solutions, respectively. For each repetition, over 10 seedlings were randomly harvested at 3, 6 and 12 h, and 1, 2 and 3 days after treatment (DAT). Approximately 1.5 cm of the root tips were quickly collected, homogenized, quickly dried with paper towel, frozen in liquid nitrogen and then stored at −80 °C for later analysis.
Morphological and anatomical analysis
Fifteen trifoliate orange seedlings which had been cultured for eight weeks in low or sufficient boron solution were randomly collected and rinsed with deionized water. For root morphology analysis, the root samples were scanned using an Epson digital scanner (Expression 10000XL 1.0, Epson Inc., Japan), and the image was analyzed using WinRhizo Pro (S) v. 2009c (Regent Instruments Inc., Canada) software. The total root length and root number per seedling were measured. The root density was defined as the ratio of the root number to the total root length. For the anatomical root analysis, approximately 1 cm of the root tip length was excised, immediately fixed in formalin–acetic acid–alcohol and stored at 4 °C until later sectioning. The preparation and bright-field microscopic observation of root tip longitudinal paraffin sections were performed according to the method described by Liu et al. [Citation30].
Determination of different forms of boron
The concentration of different forms of boron in the sampled root tips was measured according to the protocol described by Du et al. [Citation31], with slight modifications; 200 mg of 1.5-cm root tips were cut into small pieces (less than 1 mm in length) and were then transferred to a 10-mL centrifuge tube with 5 mL of 1 mol L−1 NaCl. (1 mol L−1 NaCl can completely destroy the cell membrane but cannot destroy the cell wall, allowing the boron in the cytoplasm and cell gap to be extracted by high concentration NaCl.) The samples were oscillated at 100 r min−1 for 24 h at 25 °C and were then filtered with 0.15 mm gauze and quantitative filter paper. This filtrate was defined as the soluble B solution. Then, the residues were washed with 5 mL of 1 mol L−1 HCl (1 mol L−1 HCl can destroy the cell wall, allowing for the extraction of the boron in the cell wall) into a new 10 mL centrifuge tube, oscillated at 100 r min−1 for 24 h at 25 °C and were then filtered with 0.15 mm gauze and quantitative filter paper. This filtrate was defined as the insoluble B solution. The boron concentration in the filtrates was determined using inductively coupled plasma atomic spectroscopy (ICP-AES; Thermo Inc., IRIS Advan, USA) according to the equipment manual.
Quantification of IAA in root tips
IAA was quantified according to the method described by Fu et al. [Citation32]: 200 mg of 1.5-cm root tips were homogenized in liquid nitrogen and extracted for 24 h in 2 mL of methanol containing 2H2-IAA (purchased from CDN Isotopes Inc.) as internal standard at –20 °C, and then centrifuged at 42,200 × g for 15 min at 4 °C. The supernatant was collected and purified with an Oasis Max solid phase extract cartridge (150 mg 6 cc−1, Waters). Then, the entire sample was injected into a liquid chromatography (LC)/mass spectrometry (MS)/MS system consisting of an Acquity Ultra Performance Liquid Chromatograph (Acquity UPLC; Waters) and a triple quadruple tandem mass spectrometer (Quattro Premier XE; Waters). There were three biological replicates for each treatment.
Quantitative real-time polymerase chain reaction (PCR) analysis
RNA isolation and first-strand cDNA synthesis were conducted according to the method described by An et al. [Citation33]. Gene expression levels were analyzed by quantitative real-time PCR (qRT-PCR) using SYBR® Green I (PE Applied Biosystems). Gene sequences related to IAA synthesis and transport were obtained from the Citrus clementina genome database (www.phytozome.net). Specific primers for qRT-PCR were designed using Primer 3.0.[Citation34] Transcript IDs and their corresponding primers are listed in . The qRT-PCR was performed in a 10-µL reaction volume using the SYBR® Green PCR Master Mix on an ABI 7500 Real-Time System, following the manufacturer's protocol. Each plant was analyzed with three biological replicates and each biological replicate included two technical replicates. Samples were initially incubated at 50 °C for 2 min, then at 95 °C for 10 min, and then subjected to 40 cycles of 95 °C for 15 s, 60 °C for 60 s. The Livak method [Citation4] was employed to calculate the relative gene expression level.
Table 1. Gene IDs and specific primers for quantitative real-time PCR analysis.
Statistical analysis
The data were evaluated by Duncan's multiple test or Student's t-test in the analysis of variance (ANOVA) programme in SAS (SAS Institute, Cary, USA). Differences were considered significant at P < 0.05.
Results and discussion
Effect of long-term boron deficiency on root morphology and anatomy
Boron deficiency severely inhibits root growth and development.[Citation2,Citation6,Citation13,Citation15,Citation16,Citation35] In this study, we compared the difference in root morphology and anatomy between −B and +B treatments by using eight-week hydroponic cultivation ( and ). The results showed that the primary and lateral root length was markedly reduced under boron-deficient conditions ((a) and (b)): the mean total root length per seedling was 50.03 cm ± 9.43 cm in the −B treatment, significantly lower than that in the +B treatment, where the mean total root length was 101.12 cm ± 8.99 cm. However, the mean root density was 0.74 ± 0.15 in the −B treatment, significantly higher than that in the +B treatment (0.44 ± 0.07), and there was no significant difference in the total root number between the two treatments (). The anatomical analysis of the longitudinal root section by light microscopy showed that, when compared to the +B treatment ((c)), the root tips of boron-deficient seedlings were dramatically swollen, had obvious necrosis and had larger cells ((d) and (e)). These results are in agreement with the results reported in Arabidopsis,[Citation19] suggesting that boron deficiency inhibits the root growth and development by repressing root elongation rather than root growth initiation.
Figure 1. Morphological and anatomical symptoms in trifoliate orange roots cultivated hydroponically under +B (0.25 mg L−1 boron) or −B (∼0.25 µg L−1 boron) conditions for eight weeks. Morphological symptoms of the whole roots (a) and a single root (b); longitudinal sections of root tips cultivated under +B conditions (c); different longitudinal sections of root tips cultivated under –B conditions (d, e).
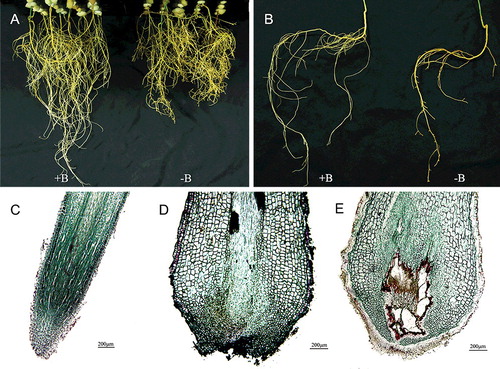
Table 2. Effects of boron deficiency on the root morphology of trifoliate orange seedlings after 8-week hydroponic cultivation.
Soluble and insoluble B and IAA content in boron-deficient root tips
Auxin plays an important role in root development, including root elongation, lateral-root initiation and emergence, and in the root apical meristem.[Citation17] In this study, we found that the insoluble B content in −B treated root tips in the long-term experiment was significantly higher than in +B treated root tips. Conversely, the soluble B content was significantly lower in the –B treated root tips compared to the +B treatment ((a)). Moreover, we found that approximately 80% of B found in –B treated root tips was insoluble B, whereas +B root tips contained a fairly equal amount of insoluble and soluble B. The IAA content was significantly lower in −B treated root tips (0.91 ng g−1) compared to the +B treated root tips (5.78 ng g−1) ((b)). Therefore, the decrease in the content of soluble B was accompanied by a decrease in the IAA content, similar to the findings in boron-deficient pea roots.[Citation29] Moreover, the profiles of B and IAA were also compared over time during the three-day experiment (). The results indicated that the insoluble B content was slightly higher in −B treated root tips than in +B treated root tips after 6 hours of treatment (HOT) and was significantly higher after 2 days of treatment (DOT) ((a)). In addition, the soluble B remained at a constant level in +B treated root tips but continuously decreased in −B treated root tips throughout the experiment. However, the soluble B content became significantly lower than that in the +B treated root tips after 12 HOT. At the end of this treatment (at 3 DOT), the mean soluble B level in −B treated root tips was 5.78 mg kg−1, approximately 62% of that in +B treated root tips (9.25 mg kg−1) ((b)). Interestingly, the mean IAA content was 5.8 ng g−1 FW in +B treated root tips whilst in the −B treated root tips, the IAA content increased gradually after 3 HOT, peaked at 1 DOT and then decreased dramatically by the end of this experiment ((c)). Such changes in the IAA content in the −B treated root tips have been widely reported in other studies.[Citation18,Citation35–39] Taken together, the results confirmed that boron deficiency affects not only the boron content, but also the IAA content in the root tips, and that the effects of boron deficiency on root development are likely related to the decrease in the IAA content.
Transcript analysis of auxin biosynthesis genes
Eaton [Citation39] first reported the similarity of symptoms in plants affected by boron deficiency and plants deficient in auxin, establishing a relationship between boron and auxin. Dyar and Webb [Citation35] reported that the growth of bean roots was significantly inhibited by a seven-day boron-deficiency treatment and that the inhibitory effect could be alleviated by adding exogenous auxin. To date, although an interaction between boron and auxin or IAA metabolism has been reported in many plants, the relationship remains unresolved.[Citation19,Citation40]
The IAA content of plant roots first depends on local IAA synthesis.[Citation17] It is well known that the TAA family of amino transferases and the YUC family of flavin monooxygenases, encoded by TAA genes and YUC genes, respectively, are the two key enzymes involved in IAA biosynthesis.[Citation21] In the present study, we analyzed the expression of two TAA genes (TAA1 and TAR2) and five YUC genes (YUC2, YUC3, YUC4, YUC6 and YUC8) from trifoliate orange root tips. It is interesting to note that the seven IAA biosynthesis genes kept a nearly constant transcript level in the +B treatment (); however, in the −B treatment, the transcript levels of TAA1 ((a)), TAR2 ((b)) and YUC3 ((d)) were significantly induced from 3 HOT to 12 HOT, whereas the transcript levels of YUC8 ((g)), YUC2 ((c)), YUC4 ((e)) and YUC6 ((f)) in the –B treatment were similar to or slightly lower than those in the +B treatment. These genes, including TAA1, TAR2, YUC3 and YUC8, whose transcript levels were significantly induced before 12 HOT by boron deficiency, are related to the two key enzymes of the TAA family of amino transferases and YUC family of flavin monooxygenases necessary for IAA.[Citation21] The induction of the transcription of these genes before 12 HOT () possibly contributed to the increase in the IAA content before one day of –B treatment ((c)).
Transcript analysis of auxin transport genes
The IAA content in plant roots depends on the IAA transport as well.[Citation17] The auxin transport is more complex in the roots and it has two distinct polarities: a rootward direction and a shootward direction, which require a variety of auxin transport proteins.[Citation25,Citation26] Here, we analyzed the transcripts of the key genes involved in IAA transport, including ABCB1, ABCB19, AUX1, LAX1, LAX2, LAX3, PIN1, PIN3 and PIN4 (). Similar to the transcript levels of the IAA biosynthesis genes, the transcript levels of these IAA transport genes were constant in the +B treatment and much more variable in the −B treatment. The expression levels of ABCB1 ((a)), LAX1 ((d)) and PIN3 ((h)) were significantly higher in the −B treatment from 3 HOT to 3 DOT. The expression level of the ABCB1 and PIN3 genes peaked at 1 DOT and was about twofold higher than that in +B treated root tips ((a) and (h)). Conversely, the expression levels of AUX1 ((c)), PIN1 ((g)) and PIN4 ((i)) were lower in the −B treatment from 3 HOT to 3 DOT. The ABCB19 transcript level was also markedly lower in −B treated root tips than in +B treated root tips at 6 and 12 HOT; the gene was then induced and its transcript level was significantly higher than that in the +B treated root tips after 1 DOT ((b)). The LAX2 ((e)) and LAX3 ((f)) gene expression levels showed no significant difference between treatments. Because AUX1, PIN1 and PIN4 belong to the rootward IAA transport genes and LAX1, ABCB1 and PIN3 belong to the shootward transport genes,[Citation25,Citation26,Citation27] the significant decrease in the expression levels of rootward IAA transport genes and the significant increase of the expression levels of shootward transport genes () implied that the influx of IAA to root tips decreased and the efflux of IAA from root tips increased. This proposed underlying mechanism could explain the root morphological changes and the IAA fluctuation in the root tips in conditions of boron deficiency. Further evidence is needed to confirm this suggested mechanism.
Conclusions
A long-term boron-deficiency treatment significantly inhibited the elongation of roots, enlarged root tips and caused severe necrosis, as well as a decrease in the soluble B and IAA content in seedling root tips. The decrease in soluble B and IAA content occurred at 3 HOT and 1 DOT, respectively. The present study supports the suggestion that boron deficiency results in decreased root length and disturbed root growth by affecting the IAA content through interference with IAA metabolism. This study further suggests that the increase in IAA content before 1 DOT may be due to the increase in IAA synthesis caused by the induction of TAA1, TAR2, YUC3 and YUC8 under boron-deficiency treatment; the significant decrease in IAA content at 3 DOT or an eight-week boron-deficiency treatment may be due to the decrease in the expression levels of IAA biosynthesis genes and rootward IAA transport genes, and the increased expression levels of IAA shootward transport genes. Taken together, these results provide a reasonable explanation for root morphological change and the IAA fluctuation in the root tips. Further research needs to focus on proving it.
Acknowledgments
The authors are grateful to Dr. Jinfang Chu, Dr. Weiping Li and Dr. Cunyu Yan (Institute of Genetics and Developmental Biology, Chinese Academy of Sciences) for their valuable technical assistance with the quantification of IAA in root tips.
Disclosure statement
No potential conflict of interest was reported by the authors.
Additional information
Funding
References
- Gupta UC, Solanki HA. Impact of boron deficiency on plant growth. Int J Bioassays. 2013;2:1048–8.
- Dell B, Huang L. Physiological response of plants to low boron. Plant Soil. 1997;193:103–8.
- Cakmak I, Römheld V. Boron deficiency-induced impairments of cellular functions in plants. Plant Soil. 1997;193:71–8.
- Hajiboland R, Farhanghi F, Aliasgharpour M. Morphological and anatomical modifications in leaf, stem and roots of four plant species under boron deficiency conditions. Anales de Biología. 2012;34:13–8.
- Rerkasem B, Jamjod S. Boron deficiency in wheat: a review. Field Crop Res. 2004;89:173–8.
- Lordkaew S, Dell B, Jamjod S, et al. Boron deficiency in maize. Plant Soil. 2011;342:207–8.
- Chen L, Han S, Qi Y, et al. Boron stresses and tolerance in citrus. Afr J Biotechnol. 2012;11:5961–8.
- Bolanos L, Brewin NJ, Bonilla I. Effects of boron on Rhizobium-legume cell-surface interactions and nodule development. Plant Physiol. 1996;110:1249–8.
- Silva DHD, Rossi ML, Boaretto AE, et al. Boron affects the growth and ultrastructure of castor bean plants. Sci Agr. 2008;65:659–8.
- Sheng O, Song S, Peng S, et al. The effects of low boron on growth, gas exchange, boron concentration and distribution of ‘Newhall’ navel orange (Citrus sinensis Osb.) plants grafted on two rootstocks. Sci Hortic. 2009;121:278–8.
- Xiao J, Yan X, Peng S, et al. Seasonal changes of mineral nutrients in fruit and leaves of ‘Newhall’ and ‘Skagg's Bonanza’ navel oranges. J Plant Nutr. 2007;30:671–8.
- Yang C-Q, Liu Y-Z, An J-C, et al. Digital gene expression analysis of corky split vein caused by boron deficiency in ‘Newhall'navel orange (Citrus sinensis Osbeck) for selecting differentially expressed genes related to vascular hypertrophy. PloS One [Internet]. 2013 [cited 2015 Nov 12];8:e65737. Available from: http://journals.plos.org/plosone/article?id=10.1371/journal.pone.0065737
- Kato Y, Miwa K, Takano J, et al. Highly boron deficiency-tolerant plants generated by enhanced expression of NIP5;1, a boric acid channel. Plant Cell Physiol. 2009;50:58–8.
- Kocábek T, Svoboda Z, Al-Zwi AM, et al. Boron-regulated hypocotyl elongation is affected in Arabidopsis mutants with defects in light signalling pathways. Environ Exp Bot. 2009;67:101–8.
- Mei L, Sheng O, Peng S, et al. Growth, root morphology and boron uptake by citrus rootstock seedlings differing in boron-deficiency responses. Sci Hortic. 2011;129:426–8.
- Zhou G, Peng S, Liu Y, et al. The physiological and nutritional responses of seven different citrus rootstock seedlings to boron deficiency. Trees. 2014;28:295–8.
- Ljung K, Hull AK, Celenza J, et al. Sites and regulation of auxin biosynthesis in Arabidopsis roots. Plant Cell. 2005;17:1090–8.
- Hirsch AM, Pengelly WL, Torrey JG. Endogenous IAA levels in boron-deficient and control root tips of sunflower. Bot Gaz. 1982;143:15–8.
- Martin-Rejano EM, Camacho-Cristobal JJ, Herrera-Rodriguez MB, et al. Auxin and ethylene are involved in the responses of root system architecture to low boron supply in Arabidopsis seedlings. Physiol Plant. 2011;142170–8.
- Pop TI, Pamfil D, Bellini C. Auxin control in the formation of adventitious roots. Not Bot Horti Agrobo. 2011;39:307–8.
- Zhao Y. Auxin biosynthesis: a simple two-step pathway converts tryptophan to indole-3-acetic acid in plants. Mol Plant. 2012;5:334–8.
- Muller D, Leyser O. Auxin, cytokinin and the control of shoot branching. Ann Bot. 2011;107:1203–8.
- Robert HS, Friml J. Auxin and other signals on the move in plants. Nat Chem Biol. 2009;5:325–8.
- Na X, Hu Y, Yue K, et al. Narciclasine modulates polar auxin transport in Arabidopsis roots. J Plant Physiol. 2011;168:1149–8.
- Peer WA, Blakeslee JJ, Yang H, et al. Seven things we think we know about auxin transport. Mol Plant. 2011;4:487–8.
- Petrášek J, Friml J. Auxin transport routes in plant development. Development. 2009;136:2675–8.
- Rahman A. Auxin: a regulator of cold stress response. Physiol Plant. 2012;147:28–8.
- Hirsch AM, Torrey JG. Ultrastructural changes in sunflower root cells in relation to boron deficiency and added auxin. Can J Bot. 1980;58:856–8.
- Li C, Pfeffer H, Dannel F, et al. Effects of boron starvation on boron compartmentation, and possibly hormone-mediated elongation growth and apical dominance of pea (Pisum sativum) plants. Physiol Plant. 2001;111:212–8.
- Liu Y, Li S, Yang C, et al. Effects of boron-deficiency on anatomical structures in the leaf main vein and fruit mesocarp of pummelo Citrus grandis (L.) Osbeck. J Hortic Sci Biotech. 2013;88:693–8.
- Du CW, Wang YH, Xu FS, et al. Study on the physiological mechanism of boron utilization efficiency in rape cultivars. J Plant Nutr. 2002;25:231–8.
- Fu J, Chu J, Sun X, et al. Simple, rapid, and simultaneous assay of multiple carboxyl containing phytohormones in wounded tomatoes by UPLC-MS/MS using single SPE purification and isotope dilution. Anal Sci. 2012;28:1081–8.
- An J, Liu Y, Yang C, et al. Isolation and expression analysis of CiNIP5, a citrus boron transport gene involved in tolerance to boron deficiency. Sci Hortic. 2012;142:149–8.
- Koressaar T, Remm M. Enhancements and modifications of primer design program Primer3. Bioinformatics. 2007;23:1289–8.
- Dyar JJ, Webb KL. A relationship between boron & auxin in C14 translocation in bean plants. Plant Physiol. 1961;36:672–8.
- Blilou I, Xu J, Wildwater M, et al. The PIN auxin efflux facilitator network controls growth and patterning in Arabidopsis roots. Nature. 2005;433:39–8.
- Mitchell RJ, Garrett HE, Cox GS, et al. Boron and ectomycorrhizal influences on indole-3-acetic acid levels and indole-3-acetic acid oxidase and peroxidase activities of Pinus echinata Mill. roots. Tree Physiol. 1986;1:1–8.
- Bohnsack CW, Albert LS. Early effects of boron deficiency on indoleacetic acid oxidase levels of squash root tips. Plant Physiol. 1977;59:1047–8.
- Eaton FM. Interrelations in the effects of boron and indoleacetic acid on plant growth. Bot Gaz. 1940;101:700–8.
- Blevins DG, Lukaszewski KM. Boron in plant structure and function. Annu Rev Plant Biol. 1998;49:481–8.