ABSTRACT
Fusarium solani NAN103 lipase was successfully overexpressed in Pichia pastoris using inducible expression system and constitutive expression system under the control of alcohol oxidase 1 promoter (pAOX1) and glyceraldehyde-3-phosphate dehydrogenase promoter (pGAP), respectively. Lipase obtained using the constitutive promoter showed the highest activity of 18.8 U/mg in 3 days of cultivation time. Optimal lipase activity was observed at pH 7.0 and 35 °C using p-nitrophenyl laurate as the substrate. Lipase activity was enhanced by Mn2+, Ba2+, Li+, Ca2+, Ni2+, CHAPS and Triton X-100 but was inhibited by Hg2+, Ag+ and SDS. The addition of 10% v/v of octanol, p-xylene, hexane and isopropanol increased lipase activity. Cultivation of lipase-expressing P. pastoris under pGAP in synthetic wastewater containing 1% w/v palm oil resulted in degradation of 87% of the oil within 72 h. P. pastoris expressing F. solani lipase from constitutive expression system has the potential to be used as an alternative microorganism for lipid degradation.
Introduction
Lipases (triacylglycerol acylhydrolase, EC 3.1.1.3) can hydrolyse long-chain triglycerides (TAGs) to glycerol and free fatty acids (FFAs) at the lipid–water interface. Moreover, lipases can catalyse a wide range of reactions, including esterification, inter-esterification, alcoholysis, acidolysis and aminolysis. One of the interesting applications of lipases is wastewater treatment. Most municipal and some industrial wastewaters contain lipids as the major component of the organic matter. Pretreatment that hydrolyses these lipids may improve the biological degradation of lipid-containing wastewaters.[Citation1] Some reports have described the use of lipases during wastewater pretreatment.[Citation2,Citation3] Lipase-producing bacteria have also been used as a bacterial consortium during the pretreatment of lipid-rich wastewater.[Citation4] In addition, Yarrowia lipolytica has been used to degrade oil wastewaters.[Citation5,Citation6]
Recombinant DNA technology represents a very attractive method to overcome the cost limitation of the application of lipases. The methylotrophic yeast Pichia pastoris has become an interesting host for the heterologous expression of proteins. The advantages of P. pastoris include a rapid growth rate in minimal medium, low levels of endogenous protein secretion, ease of genetic manipulation, the ability to efficiently secrete heterologous proteins and the ability to perform eukaryotic post-translational modifications.[Citation7,Citation8] Most P. pastoris expression systems take advantage of the methanol-induced alcohol oxidase 1 (AOX1) system. The AOX1 promoter (pAOX1), a strong inducible promoter, is used for the construction of recombinant proteins upon induction with methanol. Lipases from several microorganisms have been successfully expressed and secreted using the pAOX1 system, including those from Candida thermophila,[Citation9] Y. lipolytica,[Citation10] Rhizopus chinensis,[Citation11] Candida rugosa [Citation6] and Candida antarctica.[Citation12,Citation13] Glyceraldehyde-3-phosphate dehydrogenase (GAP) is a key enzyme in glycolysis. Its promoter (pGAP), a strong constitutive promoter, has also been used for the constitutive expression of heterologous proteins. The advantage of using this promoter is that methanol is not required for induction, and it is not necessary to switch cultures from a different carbon source to methanol.[Citation14] Lipase genes have been expressed under the control of the pGAP, including those from C. rugosa [Citation15,Citation16] and Y. lipolytica.[Citation17]
The lipase-producing fungus Fusarium solani NAN103 isolated from a soil sample in the deciduous dipterocarp forest in Nan province, Thailand, exhibited the highest lipase activity.[Citation18] In this study, we were interested in the cloning and overexpression of F. solani lipase in a P. pastoris expression system to increase lipase production. In addition, the lipid degradation capability in synthetic wastewater of recombinant P. pastoris expressing F. solani lipase was investigated. The crude recombinant lipase was applied for wastewater pretreatment; therefore, the biochemical properties of the crude recombinant lipase obtained from P. pastoris were also studied.
Materials and methods
Strains, plasmids and culture conditions
The mature lipase gene from Fusarium solani NAN103 (FSL) (Genbank accession number KT003282) inserted in the plasmid vector pPICZαA was obtained from the Biofuels by Biocatalysts Research Unit, Chulalongkorn University. Escherichia coli DH5α (Gibco, USA) and P. pastoris X-33 (Invitrogen, USA) were used as the host cells for plasmid propagation and expression of the target gene, respectively. pPICZαA and pGAPZαA vectors (Invitrogen, USA) were used for the expression of the lipase gene in P. pastoris. E. coli DH5α was grown in Luria–Bertani broth at 37 °C. P. pastoris was grown in yeast extract peptone dextrose (YPD) medium (10 g/L of yeast extract, 20 g/L of peptone and 20 g/L of glucose) at 30 °C.
Cloning of lipase gene from F. solani
The FSL gene lacking its signal sequence was amplified by PCR with gene-specific primers containing recognition sites for the restriction enzymes KpnI and NotI. The forward primer was 5′-CAGGTACCGGCCCAGTGCCCTCTGTTGATGAAA-3′, and the reverse primer was 5′-TCGCGGCCGCAGTCATCTGCTTAACAAATTCCTGATCCAG-3′ (the restriction enzyme recognition sites are underlined). The PCR reaction mixtures contained 1× Phusion high-fidelity (HF) buffer, 200 µmol/L of each dNTP, 0.5 µmol/L of each primer, 10 ng of template DNA and 1 U of Phusion DNA polymerase (New England Biolabs Inc., USA) in a total volume of 50 µL. The reaction was performed at 98 °C for 30 s, followed by 30 cycles of 98 °C for 10 s, 62 °C for 30 s and 72 °C for 30 s and then finally at 72 °C for 10 min. The amplified fragment was digested with the appropriate restriction enzymes and ligated into similarly digested expression vectors pPICZαA (pAOX1) and pGAPZαA (pGAP). The ligation products were used to transform E. coli DH5α. To verify the nucleotide sequence of the lipase genes, plasmid DNAs extracted from selected clones were sequenced by Pacific Science (Bangkok, Thailand), using the α-factor (5′-TACTATTGCCAGCATTGCTGC-3′) and 3′ AOX (5′-GCAAATGGCATTCTGACATCC-3′) sequencing primers.
P. pastoris transformation
The recombinant plasmids were linearized using PmeI and AvrII for pPICZαA and pGAPZαA, respectively, and then used to transform competent P. pastoris X-33 using electroporation performed with the Gene Pulser Xcell™ (Bio-Rad, USA). Transformants were selected on YPDS-zeocin agar (10 g/L of yeast extract, 20 g/L of peptone, 20 g/L of glucose and 1 mol/L of d-sorbitol) containing zeocin (Invitrogen, USA) at 100, 500, 1000 and 2000 μg/mL. The plates were incubated at 30 °C for 3–4 days.
Lipase expression in P. pastoris
P. pastoris cells were precultured in 5 mL of YPD, shaken at 250 rpm for 24 h at 30 °C. For the pAOX1 expression system, P. pastoris/pPICZαA-FSL was cultured in 25 mL of buffered glycerol-complex medium (BMGY; 10 g/L of yeast extract, 20 g/L of peptone, 13.4 g/L of YNB, 4 × 10−4 g/L of biotin, 10 mL/L of glycerol and 0.1 mol/L of potassium phosphate buffer, pH 6.0) shaken at 250 rpm for 18 h at 30 °C. The cell pellets were harvested and resuspended in 50 mL of buffered methanol-complex medium (BMMY; BMGY medium containing 5 mL/L of methanol instead of glycerol). Different methanol concentrations (0.5, 1, 2 and 3% v/v) were added to the BMMY medium every 24 h for 7 days to induce expression. For the pGAP expression system, P. pastoris/pGAPZαA-FSL was cultured in 25 mL of YPD medium shaken at 250 rpm for 3 days at 30 °C. Aliquots of these supernatants were collected every day to measure lipase activity.
Lipase activity and protein assay
Lipase activity in the culture supernatant was determined by the hydrolysis of p-nitrophenyl laurate (p-NPL) into lauric acid and p-nitrophenol. The assay was modified from the method of Leelaruji et al.[Citation19] Absolute ethanol was used to solubilize p-NPL. Reactions contained 250 μL of lipase, 710 μL of 50 mmol/L phosphate buffer, pH 7.0, and 40 μL of 25 mmol/L p-NPL. This hydrolytic reaction was incubated at 35 °C for 10 min and then centrifuged at 14,000 g for 5 min. The absorbance of the supernatant was measured at 405 nm using an Eon™ microplate spectrophotometer (BioTek Instruments, Inc., USA). One unit of lipase activity was defined as the amount of enzyme releasing 1 μmol of p-nitrophenol per min under the assay conditions. The total protein content was determined using Bradford's method with bovine serum albumin used as the standard.[Citation20]
Preparation of the crude recombinant lipase
To prepare crude recombinant lipase for characterization, the culture broth was centrifuged at 10,000 g for 15 min at 4 °C. The collected supernatant was concentrated approximately 10 times by ultrafiltration through a Macrosep® Advance Centrifugal Device with a 10 kDa cut-off membrane (PALL, USA).
Effect of pH on lipase activity and stability
The optimal pH of lipase activity was determined under lipase assay conditions using buffers of different pH, p-NPL as the substrate and a reaction time of 10 min at 35 °C. The following buffer systems (50 mmol/L each) were used: citric acid–sodium citrate buffer (pH 5.0–6.0), Na2HPO4–NaH2PO4 buffer (pH 7.0–8.0) and glycine–NaOH buffer (pH 9.0–10.0). The pH stability of lipase was determined by incubating lipase in buffers of different pH for 24 h at 4 °C and then analysing the residual activity under lipase assay conditions with pH 7.0 as a reference.
Effect of temperature on lipase activity and stability
The effect of temperature on lipase activity was studied between 20 and 100 °C using the p-NPL assay in phosphate buffer (pH 7.0). The thermostability of lipase was determined by preincubating the lipase at 20–80 °C for 30 min in phosphate buffer (pH 7.0). The residual activity was measured using p-NPL as the substrate at 35 °C for 10 min. Lipase incubated at 4 °C was used as a reference.
Substrate specificity of lipase
The substrate specificity of lipase towards various p-nitrophenyl esters was examined using the substrates p-nitrophenyl butyrate (C4), p-nitrophenyl caprylate (C8), p-nitrophenyl decanoate (C10), p-NPL (C12), p-nitrophenyl myristate (C14) and p-nitrophenyl palmitate (p-NPP) (C16) at a final concentration of 1 mmol/L. The lipase activity was measured in phosphate buffer (pH 7.0) at 35 °C for 10 min.
Effect of metal ions on lipase activity
The effect of metal ions on lipase activity was determined by measuring the residual activity after preincubating lipase in phosphate buffer (pH 7.0) with different metal ions at a final concentration of 1 or 5 mmol/L at 4 °C for 1 h. The metal salts used were LiCl, BaCl2, CaCl2, MgCl2, MnCl2, CuSO4, HgCl2, AgNO3, ZnSO4, CoCl2, NiCl2, FeSO4 and FeCl3. The residual activity was measured using p-NPL as substrate at 35 °C for 10 min. A sample without the addition of metal ions was used as the control.
Effect of detergents and inhibitors on lipase activity
The effect of detergents (SDS, CTAB, EDTA, CHAPS, Triton X-100 and Tween-20) and inhibitors (DTT and PMSF) on lipase activity was determined after preincubating lipase with various detergents in phosphate buffer (pH 7.0) at 4 °C for 1 h. Detergents and inhibitors were present at a final concentration of 1 or 5 mmol/L, except that Triton X-100 and Tween-20 were used at 0.1% and 1% v/v. The residual activity was measured using p-NPL as substrate at 35 °C for 10 min. A sample incubated without addition of detergent or inhibitor was taken as the control.
Effect of organic solvents on lipase activity
The effect of various organic solvents on lipase activity at 10% v/v (50 µL of organic solvents plus 450 µL of crude enzyme (30 U/mL)) was investigated using various water-immiscible organic solvents (chloroform, hexane, 1-octanol and p-xylene) and water-soluble organic solvents (methanol, ethanol, propanol, isopropanol, butanol, acetone, acetonitrile and dimethyl sulphoxide (DMSO)). The mixture was incubated at 35 °C, 200 rpm for 30 min and then incubated further at 4 °C for 24 h. The residual activity was measured using p-NPL as substrate at 35 °C for 10 min. The sample without addition of organic solvents was taken as control.
Application of recombinant P. pastoris in lipid degradation
P. pastoris/pGAPZαA-FSL was precultured in 10 mL YPD at 30 °C, 250 rpm for 24 h. Subsequently, the inoculum (4% v/v) was added to 25 mL of synthetic wastewater medium in a 250-mL flask containing (in g/L) palm oil, 10; peptone, 0.6; beef extract, 0.4; urea, 0.1; Na2HPO4, 0.1; NaCl, 0.03; CaCl2, 0.014; KCl, 0.014 and MgSO4, 0.01 (the composition of the synthetic wastewater medium was adapted from Matsumiya et al. [Citation21]). The flask was incubated at 30 °C for 72 h, with shaking at 150 rpm. Samples of the culture were taken and treated with 5 mL of chloroform to extract the lipids. The chloroform layer was used for the determination of TAG and FFA content by high performance liquid chromatography (HPLC). The HPLC analysis was performed using a Shimadzu LC-20A (Shimadzu, Japan) equipped with an Apollo Silica column (25 cm × 4.6 mm; Alltech, USA) connected to an evaporative light scattering detector equipped with nitrogen gas. The mobile phase was composed of a mixture of solution A (hexane/isopropanol/ethyl acetate/formic acid, 85:10:10:0.1 by volume) and solution B (hexane/formic acid, 100:0.2 v/v). A flow rate of 1.5 mL/min and 40 °C column temperature were used.
Results and discussion
Cloning of lipase gene from F. solani and lipase expression in P. pastoris
The lipase gene of F. solani NAN103 has an open reading frame of 999 bp. It encodes 333 amino acid residues of a protein with a putative signal peptide of 16 amino acids. FSL gene lacking signal peptide sequence was amplified and transformed into P. pastoris. The sequence encoding the α-factor secretion signal from Saccharomyces cerevisiae inserted into the vectors was used to secrete proteins. Therefore, this expression system can be used to produce extracellular recombinant lipase. To compare inducible and constitutive expression in P. pastoris, the vectors pPICZαA and pGAPZαA were used for cloning, respectively. The results, presented in , showed that P. pastoris/pPICZαA-FSL induced by 2% v/v methanol had the highest specific lipase activity of 16.47 ± 1.23 U/mg in 5 days of cultivation time ((a)), while P. pastoris/pGAPZαA-FSL produced the highest specific lipase activity, 18.81 ± 1.98 U/mg, in 3 days of cultivation time ((b)). The constitutive expression of FSL was higher than that obtained from inducible expression by 1.1-fold. The expression of F. solani lipase in P. pastoris was higher than that observed in wild-type F. solani NAN103 having specific lipase activity of 0.087 U/mg.[Citation18] The FSL gene has been produced via other heterologous expression systems, including the S. cerevisiae expression system. Nectria haematococca (anamorph F. solani f. sp. pisi) was cloned into three different strains of S. cerevisiae. They produced lipase activities of 1.247–1.501 U/mL when p-NPP was used in the lipase assay.[Citation22] The activity of the recombinant lipase from P. pastoris/pGAPZαA-FSL seems to be close to that of the recombinant lipase from S. cerevisiae. Although both pAOX1 and pGAP are strong promoters that can drive the expression of a target gene, resulting in the production of large amounts of target protein, constitutive expression systems are more suitable for a large-scale production because the use of methanol causes environmental pollution.[Citation14] The yield of F. solani lipase from the constitutive expression system was higher than that from the induced expression system, and it required a shorter incubation time. Therefore, P. pastoris/pGAPZαA-FSL and the enzyme it produced were selected for further characterization and application studies.
Table 1. Lipase activity of recombinant P. pastoris.
Figure 1. Specific lipase activity of (a) P. pastoris/pPICZαA-FSL cultured in 50 mL of BMMY at 30 °C and 250 rpm. Initially, 0.5% v/v methanol was added. Thereafter, methanol at 0.5% v/v (•), 1% v/v (○), 2% v/v (▾) and 3% v/v (▵) was added every day. (b) P. pastoris/pGAPZαA-FSL was cultured in 50 mL of YPD at 30 °C, 250 rpm. Data are means of three independent experiments and error bars indicate standard deviation.
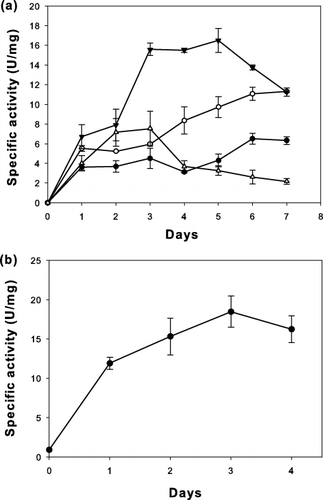
Effect of pH and temperature on lipase activity and stability
As seen in (a), the crude lipase from P. pastoris/pGAPZαA-FSL exhibited optimal lipase activity at pH 7.0. The enzyme was stable within a pH range of 6.0–7.0 and retained at least 80% activity after 24 h of incubation. At pH 8.0–9.0, the enzyme showed approximately 50% activity. In contrast, the purified lipase from F. solani characterized by Jallouli et al. [Citation23] was almost equally stable at pH 8.5–9.
Figure 2. Effect of (a) pH and (b) temperature on lipase activity (solid line) and stability (dashed line) of the recombinant lipase from P. pastoris/pGAPZαA-FSL. Data are means of three independent experiments and error bars indicate standard deviation.
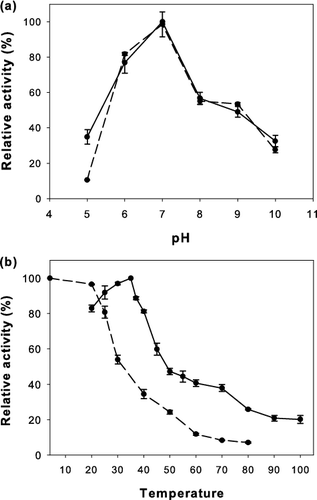
The optimum temperature of the enzyme was 35 °C. The lipase activity decreased rapidly above 40 °C. In fact, most fungal lipases are not stable above 40 °C.[Citation24] Above 40 °C, the recombinant lipase was less active, which was similar to the result obtained with purified lipase from F. solani.[Citation23] With respect to the enzyme's thermostability, the residual lipase activities after incubation at 20 and 25 °C for 30 min in phosphate buffer (pH 7.0) were 96% and 81%, respectively. Significant losses of lipase activity were observed when the enzyme was incubated for 30 min at temperatures above 25 °C, as shown in (b). This result indicated that the thermostability of this recombinant lipase was poor.
Substrate specificity of lipase
The substrate specificity of the crude lipase towards various p-nitrophenyl esters was investigated (). The relative activity was above 70% with carbon chain lengths from C4 to C14 but declined to 50% with p-NPP (C16). The highest lipase activity was obtained with p-NPL (C12). This suggests that the recombinant lipase from P. pastoris/pGAPZαA-FSL has wide substrate specificity. Similar substrate preference property was found for lipase from Aspergillus carneus, which showed maximum hydrolysis activity to p-NPL.[Citation24] Unlike the recombinant lipase (Lip5-DM) from Candida albicans expressed in P. pastoris, the lipase exhibited a preference for the short- and medium chain length p-nitrophenyl esters (C4 and C8) rather than the long chain length p-nitrophenyl esters (C12, C16 and C18). The highest lipase activity was obtained with C8,[Citation25] whereas the recombinant lipase from C. thermophila SRY-09 expressed in P. pastoris showed high activity towards p-nitrophenyl caproate (C6).[Citation9]
Effect of metal ions on lipase activity
Various metal ions had different effect on lipase activity, as shown in . Mn2+, Ba2+, Li+, Ca2+ and Ni2+ (1 mmol/L) stimulated lipase activity, whereas Ag+, Zn2+, Fe2+, Co2+, Cu2+ and Fe3+ ions decreased lipase activity. The addition of Ni2+ increased the relative activity by 90%. The presence of Hg2+ and Ag+ strongly inhibited lipase activity. The lipase activity was greatly inhibited by higher concentrations (5 mmol/L) of most of the metal ions, except that Li+ and Ni2+ were found to enhance lipase activity. The activity of recombinant lipase decreased in the presence of Hg2+, as was observed with the lipases from Penicillium aurantiogriseum,[Citation26] Mucor sp. [Citation27] and Aureobasidium pullulans.[Citation28] Hg2+ may affect enzyme conformation because the thiol group in sulphur-containing amino acid residues may have a high affinity to Hg2+.[Citation28] The increased lipase activity in the presence of Ca2+ (1 mmol/L) is somewhat similar to that of purified F. solani lipase, which requires Ca2+ to stimulate its lipase activity.[Citation23] Many lipases possess a calcium-binding motif around the catalytic site. Therefore, calcium ions might remove the fatty acids as insoluble salts and stabilize the enzyme.[Citation29] In addition, Li+ and Ni2+ at 1 mmol/L resulted in enhancing lipase activity, similar to a lipase from C. antarctica ZJB09193.[Citation13]
Table 2. Effect of various metal ions on the lipase activity of recombinant lipase from P. pastoris/pGAPZαA-FSL.
Effect of detergents and inhibitors on lipase activity
Lipase activity was strongly inhibited by SDS. At low concentration, the addition of CHAPS (1 mmol/L) and Triton X-100 (0.1% v/v) activated lipase activity, resulting in relative increases of 87% and 31%, respectively. DTT, Tween 20 and Triton X-100 at high concentration enhanced lipase activity, whereas PMSF, CHAPS, CTAB and EDTA reduced activity by 42%, 40%, 23% and 11%, respectively (). The recombinant lipase from P. pastoris/pGAPZαA-FSL appears to be the most active in the buffer containing 1 mmol/L of CHAPS or 1% v/v of Triton X-100. Therefore, the recombinant lipase could be potentially applied using CHAPS and Triton X-100 as surfactants. These results suggest that lipase from P. pastoris/pGAPZαA-FSL is activated by non-ionic detergents (Tween 20 and Triton X-100) whereas ionic detergents (SDS and CTAB) have an inhibitory effect on lipase activity. Similar effect was reported by Prazeres et al. [Citation30] in the case of the lipase from F. oxysporum. This enzyme was reported to be stimulated by Triton X-100 and Triton X-114 and strongly inhibited by SDS.
Table 3. Effect of various detergents and inhibitors on the lipase activity of recombinant lipase from P. pastoris/pGAPZαA-FSL.
Effect of organic solvents on lipase activity
P. pastoris/pGAPZαA-FSL lipase was stable in both water-miscible (−2.5 < log P < 1) and water-immiscible organic solvents (1 < log P < 4), as shown in . Lipase activity increased after incubation for 24 h in 10% v/v of octanol, p-xylene, hexane and isopropanol with a residual activity of 129.3%, 119.5%, 115.5% and 108.7%, respectively. Lipase activity was not strongly inhibited by any organic solvent except that butanol decreased lipase activity by 50%. The differences between lipase activities in different solvents may be due to direct interactions between the solvent molecules and enzyme molecules. Some solvents can induce the opening of the lid of lipase which facilitates the access of the substrate to the active site of lipase. Some solvents can act as competitive inhibitors in the reaction catalysed.[Citation31,Citation32] According to these results, butanol seems to be the competitive inhibitor for lipase from P. pastoris/pGAPZαA-FSL. Unlike the result of crude lipase from F. solani FS1, lipase activity increased by incubation in n-hexane and toluene but decreased by incubation in water-miscible organic solvents.[Citation33]
Table 4. Effect of various organic solvents on lipase activity of recombinant lipase from P. pastoris/pGAPZαA-FSL.
Application of recombinant P. pastoris in lipid degradation
The ability of P. pastoris/pGAPZαA-FSL to degrade palm oil in synthetic wastewater was tested. There was almost no difference between using 106 and 107 colony-forming units/mL (CFU/mL) of yeast cells in the reduction of TAG content ((a)). The TAG content decreased rapidly within 24 h of yeast cell addition and subsequently decreased gradually after 24 h. The higher cell concentration resulted in a greater decrease of FFA content. It might be due to more capability of FFA consumption. When 107 CFU/mL of P. pastoris/pGAPZαA-FSL was added to synthetic wastewater medium, the palm oil decreased from 10 to 1.3 g/L within 72 h, which was equal to 87% degradation ((b)).
Figure 4. Analysis of oil degradation by P. pastoris/pGAPZαA-FSL cultured in synthetic wastewater containing 10 g/L of palm oil shaken at 150 rpm for 3 days at 30 °C. (a) TAG (dashed line) and FFA (solid line) content (mmol/L) and (b) amount degraded (%). The different concentrations of yeast cells were 106 CFU/mL (close circle) and 107 CFU/mL (open circle). Data are means of three independent experiments and error bars indicate standard deviation.
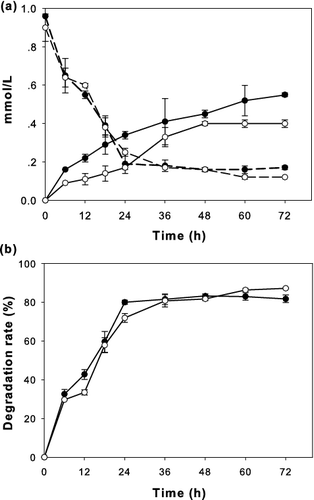
The use of lipase alone in lipid hydrolysis cannot remove FFA. Therefore, lipase-producing microorganisms should be used for lipid degradation because they can consume the FFA via β-oxidation. P. pastoris/pGAPZαA-FSL showed the ability to degrade lipids and reduce the concentration of FFA. Similarly, Matsumiya et al. [Citation21] reported that Burkholderia sp. DW2-1 grew well in synthetic wastewater medium and efficiently degraded lipids at high oil concentrations (10 g/L), reducing the lipid content by more than 90% after 48 h of cultivation. The recombinant Pichia grew in the synthetic wastewater medium containing palm oil and produced lipase to hydrolyse lipids to FFAs and glycerol. Although the synthetic wastewater medium has a carbon source such as glucose, it is possible that P. pastoris/pGAPZαA-FSL can use glycerol and oleic acid obtained from palm oil hydrolysis as carbon sources for the growth and expression of recombinant lipase under the control of pGAP. Glucose, glycerol, oleic acid and methanol have been used as the carbon source for the pGAP expression system in P. pastoris.[Citation14] It is possible to develop this organism into an aerobic wastewater treatment system. In order to reduce costs of wastewater treatment, it is not necessary to separate lipase from yeast cell before using. P. pastoris/pGAPZαA-FSL can be used as a whole-cell biocatalyst in the system. The recombinant P. pastoris can grow and produce lipase simultaneously in wastewater. It is better than the use of lipase alone in the pretreatment.
Conclusions
F. solani lipase gene was successfully overexpressed in P. pastoris using two different promoters. The recombinant lipase from the constitutive expression system (pGAP) exhibited higher lipase production than that from the induced expression system (pAOX1). The lipase-expressing P. pastoris under pGAP showed the ability to degrade palm oil in synthetic wastewater. It could be a good candidate for application in lipid-containing wastewater pretreatment.
Acknowledgments
Jinaporn Wongwatanapaiboon was supported by graduate scholarship under the Development and Promotion of Science and Technology Talents Project (DPST), the Institute for the Promotion of Teaching Science and Technology (IPST). Waraporn Malilas was supported by the Ratchadapiseksomphot Fund for Post-doctoral Fellowships, Chulalongkorn University. The authors would like to thank Paweenuch Lekhapan and Thanakorn Chanhom for their helpful assistance; PTT Research and Technology Institute for providing research collaboration; Enago (www.enago.com) for the English language review.
Disclosure statement
No potential conflict of interest was reported by the authors.
References
- Cammarota MC, Freire DMG. A review on hydrolytic enzymes in the treatment of wastewater with high oil and grease content. Bioresour Technol. 2006;97:2195–2210.
- Rigo E, Rigoni RE, Lodea P, et al. Application of different lipases as pretreatment in anaerobic treatment of wastewater. Environ Eng Sci. 2008;25:1243–1248.
- Rigo E, Rigoni RE, Lodea P, et al. Comparison of two lipases in the hydrolysis of oil and grease in wastewater of the swine meat industry. Ind Eng Chem Res. 2008;47:1760–1765.
- Prasad MP, Manjunath K. Comparative study on biodegradation of lipid-rich wastewater using lipase producing bacterial species. Indian J Biotechnol. 2011;10:121–124.
- Lan WU, Gang GE, Jinbao WAN. Biodegradation of oil wastewater by free and immobilized Yarrowia lipolytica W29. J Environ Sci. 2009;21:237–242.
- Domínguez A, Deive FJ, Angeles Sanromán M, et al. Biodegradation and utilization of waste cooking oil by Yarrowia lipolytica CECT 1240. Eur J Lipid Sci Technol. 2010;112:1200–1208.
- Li P, Anumanthan A, Gao XG, et al. Expression of recombinant proteins in Pichia pastoris. Appl Biochem Biotechnol. 2007;142:105–124.
- Ferrer P, Alarcón M, Ramón R, et al. Recombinant Candida rugosa LIP2 expression in Pichia pastoris under the control of the AOX1 promoter. Biochem Eng J. 2009;46:271–277.
- Thongekkaew J, Boonchird C. Molecular cloning and functional expression of a novel extracellular lipase from the thermotolerant yeast Candida thermophila. FEMS Yeast Res. 2007;7:232–243.
- Yu M, Lange S, Richter S, et al. High-level expression of extracellular lipase Lip2 from Yarrowia lipolytica in Pichia pastoris and its purification and characterization. Protein Expr Purif. 2007;53:255–263.
- Yu XW, Wang LL, Xu Y. Rhizopus chinensis lipase: gene cloning, expression in Pichia pastoris and properties. J Mol Catal B Enzym. 2009;57:304–311.
- Pfeffer J, Richter S, Nieveler J, et al. High yield expression of Lipase A from Candida antarctica in the methylotrophic yeast Pichia pastoris and its purification and characterisation. Appl Microbiol Biotechnol. 2006;72:931–938.
- Liu ZQ, Zheng XB, Zhang SP, et al. Cloning, expression and characterization of a lipase gene from the Candida antarctica ZJB09193 and its application in biosynthesis of vitamin A esters. Microbiol Res. 2012;167:452–460.
- Zhang AL, Luo JX, Zhang TY, et al. Recent advances on the GAP promoter derived expression system of Pichia pastoris. Mol Biol Rep. 2009;36:1611–1619.
- Chang SW, Lee GC, Shaw JF. Efficient production of active recombinant Candida rugosa LIP3 lipase in Pichia pastoris and biochemical characterization of the purified enzyme. J Agric Food Chem. 2006;54:5831–5838.
- Zhao W, Wang J, Deng R, et al. Scale-up fermentation of recombinant Candida rugosa lipase expressed in Pichia pastoris using the GAP promoter. J Ind Microbiol Biotechnol. 2008;35:189–195.
- Wang X, Sun Y, Ke F, et al. Constitutive expression of Yarrowia lipolytica lipase LIP2 in Pichia pastoris using GAP as promoter. Appl Biochem Biotechnol. 2012;166:1355–1367.
- Malilas W. Screening and induced mutation of lipolytic fungi to enhance hydrolytic activity [dissertation]. Bangkok: Chulalongkorn University; 2012.
- Leelaruji W, Piamtongkam R, Chulalaksananukul S, et al. Biodiesel production from Jatropha curcas oil catalyzed by whole cells of Aureobasidium pullulans var. melanogenum SRY 14-3. Afr J Biotechnol. 2013;12:4380–4386.
- Bradford MM. A rapid and sensitive method for the quantitation of microgram quantities of protein utilizing the principle of protein-dye binding. Anal Biochem. 1976;72:248–254.
- Matsumiya Y, Wakita D, Kimura A, et al. Isolation and characterization of a lipid-degrading bacterium and its application to lipid-containing wastewater treatment. J Biosci Bioeng. 2007;103:325–330.
- Nasser Eddine A, Hannemann F, Schafer W. Cloning and expression analysis of NhL1, a gene encoding an extracellular lipase from the fungal pea pathogen Nectria haematococca MP VI (Fusarium solani f. sp. pisi) that is expressed in planta. Mol Genet Genomics. 2001;265:215–224.
- Jallouli R, Khrouf F, Fendri A, et al. Purification and biochemical characterization of a novel alkaline (phospho) lipase from a newly isolated Fusarium solani strain. Appl Biochem Biotechnol. 2012;168:2330–2343.
- Saxena RK, Davidson WS, Sheoran A, et al. Purification and characterization of an alkaline thermostable lipase from Aspergillus carneus. Process Biochem. 2003;39:239–247.
- Lan DM, Yang N, Wang WK, et al. A novel cold-active lipase from Candida albicans: cloning, expression and characterization of the recombinant enzyme. Int J Mol Sci. 2011;12:3950–3965.
- Lima VMG, Krieger N, Mitchell DA, et al. Activity and stability of a crude lipase from Penicillium aurantiogriseum in aqueous media and organic solvents. Biochem Eng J. 2004;18:65–71.
- Abbas H, Hiol A, Deyris V, et al. Isolation and characterization of an extracellular lipase from Mucor sp strain isolated from palm fruit. Enzym Microb Technol. 2002;31:968–975.
- Liu Z, Chi Z, Wang L, et al. Production, purification and characterization of an extracellular lipase from Aureobasidium pullulans HN2.3 with potential application for the hydrolysis of edible oils. Biochem Eng J. 2008;40:445–451.
- Fickers P, Marty A, Nicaud JM. The lipases from Yarrowia lipolytica: Genetics, production, regulation, biochemical characterization and biotechnological applications. Biotechnol Adv. 2011;29:632–644.
- Prazeres JNd, Cruz JAB, Pastore GM. Characterization of alkaline lipase from Fusarium oxysporum and the effect of different surfactants and detergents on the enzyme activity. Braz J Microbiol. 2006;37:505–509.
- Adlercreutz P. Immobilisation and application of lipases in organic media. Chem Soc Rev. 2013;42:6406–6436.
- Su J, Zhang F, Sun W, et al. A new alkaline lipase obtained from the metagenome of marine sponge Ircinia sp. World J Microbiol Biotechnol. 2015;31:1093–1102.
- Maia MMD, Heasley A, Camargo de Morais MM, et al. Effect of culture conditions on lipase production by Fusarium solani in batch fermentation. Bioresour Technol. 2001;76:23–27.