ABSTRACT
Because of tumour dependence on angiogenesis, anti-angiogenic therapy has become the most attractive area of basic and clinical study in the field of cancer research. In order to create a synergistic effect on angiogenesis and immune regulation, we designed and constructed a new type of DNA vaccine that can express VEGFR2 (vascular endothelial growth factor receptor 2) and the prostate cancer antigen IL-12 (interleukin 12) in the same reading frame. The aim of this study was to investigate the anti-tumour activity of a eukaryotic expression plasmid carrying a fusion gene of human VEGFR2 and IL-12. According to the gene sequences in GenBank, we synthesized the human VEGFR2 and IL-12 genes. VEGFR2 and IL-12 were joined by a sequence encoding a Furin recognition site and a 2A cleavage site, and the resulting fusion gene was cloned into the eukaryotic expression vector pVAX1 to construct the expression plasmid pVAX1-VEGFR2-F2A-IL-12. The expression of VEGFR2 and IL-12 could be detected in 293T cells transfected with pVAX1-VEGFR2-F2A-IL-12 by enzyme-linked immunosorbent assay. Each of these proteins, and in particular co-expression of both proteins, can result in humoral and cellular immune responses in C57BL/6 mice. After injection into the tumour-bearing mouse model, the plasmid showed stronger inhibition of tumour growth than a plasmid expressing VEGFR2 alone. Our results demonstrate that a DNA vaccine carrying a fusion gene of human VEGFR2 and IL-12 could represent a promising approach for tumour immunotherapy.
KEYWORDS:
Introduction
Over the past two decades, DNA vaccines developed rapidly and were considered an innovative and promising approach for cancer immunotherapy because of their significant advantages compared to traditional vaccines.[Citation1,Citation2] However, the effects of DNA vaccines were not satisfactory in large animal models or human trials. A number of approaches were tested for enhancing the immunogenicity and efficacy of DNA vaccines, including improved plasmid design; immunomodulatory and immune-enhancing molecules; prime-boost administration; and strategies to break the immunosuppressive networks.[Citation2–4] Because different strategies focused on different aspects of DNA vaccination, their combination was necessary for rational DNA vaccine design. The activation of both humoral and cellular immune responses has been shown to be essential for efficient cancer immunotherapy.[Citation5] Fusion genes linking tumour antigens with one of a wide variety of immunostimulatory molecules would allow for single DNA vaccines capable of multiple functions, and for manipulation of different immune activation pathways.[Citation5–7] With the rapidly growing number of tumour-associated antigens (TAAs), immunostimulatory molecules and different strategies, it is also important to optimize the design of DNA vaccines to enhance their efficacy.
Tumour formation, development and metastasis are all closely related to the formation of new blood vessels (angiogenesis). Accordingly, angiogenesis has become an important subject in the field of anti-tumour research in recent years.[Citation8] VEGFR2 (vascular endothelial growth factor receptor 2) is a major member of the VEGF receptor family, which is also known as KDR, Flk-1 (mice) or Quek1 (quail). VEGFR2 is characterized by its expression in tumour endothelial cells, and by its involvement in the proliferation and migration of endothelial cells.[Citation9,Citation10] Based on the fact that VEGFR2 is expressed in the newborn endothelial cells of blood vessels, this molecule is usually considered a marker of tumour cell invasion and metastasis, and it is an ideal target for a DNA vaccine. VEGFR2 has seven immunoglobulin-like domains in its extracellular region, of which the second, third and fourth ones are critical for ligand binding. These three domains are usually selected as target regions for DNA vaccine constructs. The use of VEGFR2 as a target for anti-tumour therapy has led to significant results, including effective inhibition of tumour angiogenesis, and consequent inhibition of tumour growth and metastasis.[Citation11,Citation12]
Interleukin 12 (IL-12) is a cytokine secreted by dendritic cells and macrophages that can activate natural killer (NK) cells, macrophages and T-cells. IL-12 enhances the cytotoxic activity of NK cells and promotes the destruction of infectious pathogens and tumour cells.[Citation11] IL-12 also has anti-angiogenic activity.[Citation13] By increasing the production of interferon gamma (IFN-γ), IL-12 can induce the expression of chemotaxis factor IP-10, which can inhibit tumour angiogenesis.[Citation14] In the present study, the VEGFR2 and IL-12 genes were joined by a Furin recognition site and a 2A linker and cloned into an expression plasmid to produce the DNA vaccine pVAX1-VEGFR2-F2A-IL-12. The possible immune activation, anti-angiogenesis and anti-tumour effects of this DNA vaccine were investigated.
Material and methods
Plasmid DNA constructs and preparation
Using the protein-encoding region of human VEGFR2 (GenBank: EU826563.1) and human IL-12 (GenBank: AF101062.1), we designed a VEGFR2–IL-12 fusion gene. The VEGFR2 gene was connected by a Furin recognition site and a 2A cleavage peptide linker to the IL-12 gene. The synthesis of the fusion gene was carried out by GENEWIZ Inc. (Suzhou, China). The fusion gene was then cloned into the eukaryotic expression vector pVAX1, using KpnI and XhoI restriction sites to make the eukaryotic expression plasmid pVAX1-VEGFR2-F2A-IL-12. Cloning and insertion were verified by double restriction enzyme digestion. The pVAX1 and pVAX1-VEGFR2-F2A-IL-12 plasmids were transiently transfected into 293T cells (China Infrastructure of Cell Line Resources, Beijing, China), and flow cytometry or enzyme-linked immunosorbent assays (ELISAs) were performed to detect the expression of the VEGFR2 and IL-12 proteins.
Detection of VEGFR2 and IL-12 expression by flow cytometry and ELISA
Forty-eight hours after transfection with the recombinant plasmid pVAX1-VEGFR2-F2A-IL-12, the cells and the culture supernatant from six-well plates were collected separately. Because VEGFR2 is localized within cell membranes, the goat anti-VEGFR2 antibody (Santa Cruz, sc-19530, CA, USA) and the fluorescein isothiocyanate (FITC)-labelled rabbit anti-goat IgG (Santa Cruz, sc-2777, CA, USA) were incubated with these cells at room temperature for 60 min, sequentially. Subsequently, the cells were washed and analyzed using a flow cytometer (FACSCalibur, BD Biosciences, San Jose, CA, USA) to determine the percentage of VEGFR2+ cells.
The collected supernatant was added to the wells of a 96-well plate at various dilutions and incubated at 4 °C overnight. The next day, the ELISA plate was washed three times with phosphate-buffered saline (PBS), then mouse anti-human IL-12 antibody (Santa Cruz, sc-65354, CA, USA) and horseradish peroxidase (HRP)-labelled goat anti-mouse IgG (Santa Cruz, sc-2055, CA, USA) were sequentially incubated with the coated supernatant for 1 h each at 37 °C. After washing three times with PBS, the colour reaction was performed with 3,3′,5,5′-tetramethylbenzidine (TMB). After the reaction was stopped by addition of 2 mol/L of H2SO4, the optical density (OD) at 450 nm was detected using a microplate spectrophotometer (Nanodrop 2000, Thermo Fisher Scientific, Waltham, MA, USA).
Mice and cell lines
Female C57BL/6 mice (4–6 weeks old) were purchased from Beijing Weitong Lihua Experimental Animal Technology Co. Ltd. (Beijing, China), and were maintained in accordance with the Guide for the Care and Use of Laboratory Animals (NIH Publication No. 85-23, Revised 1996). The mouse melanoma tumour cell line B16-F10 was purchased from the Shanghai Cell Institute (Shanghai, China). To generate a cell line stably expressing human VEGFR2, B16-F10 cells were transfected with a plasmid carrying VEGFR2 (pIRES-neo-VEGFR2) and then subjected to selection by treatment with G418. This cell line is hereafter referred to as B16-F10-VEGFR2.
DNA vaccination
Female C57BL/6 mice were randomly divided into four groups. The four groups of mice (n = 5) were vaccinated three times at 10-day intervals with 50 µg plasmid by injection into the quadriceps muscle followed by electroporation. The mice were sacrificed at the 14th day after the final immunization, and the related immunological tests were performed. Experimental procedures were in strict agreement with international guidelines for the care and use of laboratory animals and were approved by the Animal Ethics Committee of Chongqing Medical University.
Assay of antibodies in serum
To evaluate the humoral responses in the mouse model, anti-VEGFR2 antibodies in the sera of immunized mice were identified by ELISA. The 96-well microplates were coated with 10 μg/mL recombinant VEGFR2 antigen in bicarbonate buffer, pH 7.2, and incubated at 4 °C overnight. After blocking with 5% powdered milk in PBS containing Tween-20, sera taken from the mice on day 14 after the last immunization were serially diluted in PBS and incubated on the plates for 2 h at room temperature. After washing with PBS, the plates were incubated with a 1:1000 dilution of HRP-conjugated goat anti-mouse IgG antibody (Zhong Shan Jin Qiao Biological Technology, Beijing, China) at room temperature for 1 h. The reaction was stopped with 2 mol/L H2SO4, and the absorbance at 450 nm (OD450 nm) was measured using an ELISA plate reader (680 Microplate Reader, BIO-RAD, Hercules, CA, USA).
ELISPOT assay
Two weeks after the last vaccination, splenocytes from control and vaccinated groups of mice were isolated. The IFN-γ enzyme-linked immunospot (ELISPOT) assay was performed according to the protocol supplied by the manufacturer (Dakewe Biotech Ltd., Shenzhen, China). In brief, the 96-well plates were coated with anti-mouse interferon (IFN-γ) monoclonal antibody (mAb) at 4 °C overnight, and blocked for 1 h at 37 °C. The fresh isolated splenocytes (5 × 105 cells/well) from each vaccinated mice group were added to the wells and incubated with 10 μg/mL of recombinant VEGFR2 protein for 48 h. Each assay was performed in triplicate. The spots were counted and analyzed with an ELISPOT Reader (AID, Ebinger, Germany).
In vivo tumour treatment experiments
Seven days before the challenge with the tumour cells, mice were vaccinated with 50 μg of empty vector pVAX1, pVAX1-VEGFR2 or pVAX1-VEGFR2-F2A-IL-12. Subsequently, 4–6-week-old female C57BL/6 mice were injected in the right side of the abdomen with 1.0 × 105 B16-F10-VEGFR2 cells, and the mice were vaccinated two more times, with seven days between vaccinations. Tumour development was monitored in individual mice every two days. The following parameters were evaluated on the 50th day after vaccination: tumour growth inhibition rate (%) [(Wc − Wt) × 100/Wc, where Wc is the average tumour weight in the control group and Wt is the average tumour weight in the treatment group], tumour volume (mm3) (V = 0.5 × dl × ds2, where dl is the long diameter and ds is the short diameters) and tumour weight (XPE105 analytical balance, METTLER TOLEDO, Ockerweg, Germany).
Data analysis
Differences between groups were analyzed by one-way analysis of variance (ANOVA) using the SPSS 17.0 software. Differences were considered to be statistically significant at p < 0.05.
Results and discussion
Expression of VEGFR2 and IL-12 from the DNA vaccine plasmid in 293T cells
The expression of proteins from the DNA vaccine plasmid in 293T cells was verified. The expression of VEGFR2 was analyzed by flow cytometry and the results showed that the fusion gene can be expressed in cells at a high level, and positive expression was detected in 18.37% of the cells ((a)). Because IL-12 is expressed then secreted, ELISA analysis was performed on the culture supernatant to detect the expression of IL-12. The ELISA results showed that the average expression of IL-12 in the pVAX1-VEGFR2-F2A-IL-12 transfected cultures was significantly higher than that in the untransfected control group or the empty vector group, and that the DNA vaccine plasmid can drive IL-12 expression in eukaryotic cells ((b)).
Antibody assays
Anti-VEGFR2 antibodies were detected in mice vaccinated with pVAX1-VEGFR2-F2A-IL-12 and pVAX1-VEGFR2 plasmids at two weeks after their last immunization. As shown in (a), vaccination with either pVAX1-VEGFR2-F2A-IL-12 or pVAX1-VEGFR2 induced production of anti-VEGFR2 antibodies. The data showed that the levels of antibody production induced by pVAX1-VEGFR2-F2A-IL-12 were similar to those induced by pVAX1-VEGFR2 ((b)). The antibody titres of mice vaccinated with pVAX1-VEGFR2-F2A-IL-12 were not significantly higher than those of mice vaccinated with pVAX1-VEGFR2 (p < 0.05).
ELISPOT assays
Two weeks after their last immunization, the IFN-γ ELISPOT assay of mouse spleen lymphocytes was performed to detect the antigen-specific cellular immune response. As shown in (a), VEGFR2-specific IFN-γ secretion was induced in mice immunized with pVAX1-VEGFR2 and mice immunized with pVAX1-VEGFR2-F2A-IL-12. The number of spots detected in the assay using cells from the vaccinated groups was significantly higher than the number of spots detected using cells from the pVAX1 vector-only controls (p < 0.05). Further, the number of spots in the assay using cells from mice immunized with pVAX1-VEGFR2-F2A-IL-12 was higher than the number of spots in the assay using cells from mice immunized with pVAX1-VEGFR2 (p < 0.05) ((b)). These results showed that the release of IFN-γ from the spleen cells of mice was significantly enhanced by co-expression of IL-12 with VEGFR2. Overall, these results indicated that pVAX1-VEGFR2-F2A-IL-12 significantly enhanced the release of IFN-γ in the spleen cells of mice.
Anti-tumour efficacy of the pVAX1-VEGFR2-F2A-IL-12 DNA vaccine
In recent years, immune therapy technology has developed rapidly, and has played an important role in the treatment of many diseases. This is especially true in the treatment of cancer, where immune therapy has shown great potential and therapeutic effect.[Citation15] Anti-tumour immunotherapy using vaccines has become a prominent research topic, and much progress has been made using this approach. In 2012, the world's first anti-tumour therapeutic vaccine, PROVENGE®, was approved by the United States Food and Drug Administration (FDA) for prostate cancer treatment.[Citation16] However, many studies have indicated that a vaccine containing only an antigen is not effective enough to induce an anti-tumour immune response, inhibit tumour growth, inhibit recurrence or inhibit metastasis.[Citation17,Citation18] At present, the synergistic combination of antigens with different immunostimulatory molecules to produce significantly improved anti-tumour vaccines is a key avenue of investigation.
In this study, tumour growth in mice treated with pVAX1-VEGFR2 or pVAX1-VEGFR2-F2A-IL-12 was significantly inhibited compared with tumour growth in mice treated with pVAX1 vector (p < 0.05) ((a)). These results are in line with the important role that angiogenesis plays in the process of tumour development, as it is necessary for the tumour to meet its nutritional needs. Because of tumour dependence on angiogenesis, anti-angiogenic therapy has become the most attractive area of basic and clinical study in the field of cancer research.[Citation19] Vascular endothelial growth factor (VEGF) and its receptor (VEGFR2) have become popular subjects for study because of their role in angiogenesis. A variety of research on anti-tumour therapy with VEGFR2 as a target has demonstrated significant effects, including inhibition of tumour angiogenesis, tumour growth and metastasis.[Citation20–22]
Figure 4. Anti-tumour efficacy of DNA vaccines in a tumour-bearing mouse model after immunization. Growth curve of a B16-F10-VEGFR2 tumour (a); mean tumour weight (b); and tumour growth inhibition rate (c) on day 50 after vaccination.
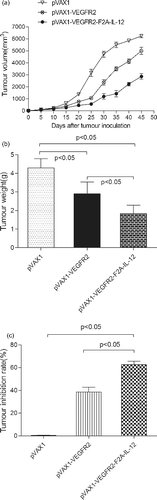
In addition, the pVAX1-VEGFR2-F2A-IL-12 vaccine inhibited the tumour growth more effectively than pVAX1-VEGFR2 vaccine (p < 0.05). IL-12 is a cytokine secreted by dendritic cells and macrophages that has the ability to promote the proliferation of NK cells, macrophages and T-cells. IL-12 also enhances the cytotoxic activity of NK cells, promoting the killing and removal of infectious pathogens and tumour cells. It has been demonstrated that many cytokines, including IL-12, have anti-tumour effects and can be used as adjuvants.[Citation23,Citation24] IL-12 also has anti-angiogenic activity. IL-12 increases the production of IFN-γ, and can induce higher expression of the chemotaxis factor IP-10, which, in turn, inhibits tumour angiogenesis.
We also measured the tumour weights ((b)), and calculated the tumour growth inhibition rates in mice ((c)) after treatment with the different vaccine plasmids. The tumour weights in the mice immunized with pVAX1-VEGFR2-F2A-IL-12 were significantly lower compared to the tumour weights in mice immunized with pVAX1-VEGFR2 (p < 0.05) or pVAX1 vector (p < 0.05) ((b)). The tumour growth inhibition rate in the mice immunized with pVAX1-VEGFR2-F2A-IL-12 was also significantly higher than in mice immunized with pVAX1-VEGFR2 (p < 0.05) or pVAX1 vector (p < 0.05) ((c)).
Taken together, our results demonstrate that the anti-tumour effect of VEGFR2 combined with IL-12 is greater than the anti-tumour effect of VEGFR2 alone. Therefore, we suggest that co-expressing VEGFR2 and IL-12 has great potential as a gene therapy technique to inhibit tumour angiogenesis. To date, DNA vaccines designed in this way have been rare, and only a few innovative examples exist. This method could be used with other tumour vaccine antigens to create a dual effect combining the specific immune response with the inhibition of tumour angiogenesis. Overall, our study demonstrates that a plasmid co-expressing VEGFR2 and IL-12 has strong potential as a DNA vaccine for use in anti-tumour therapy.
Conclusions
In order to create a synergistic effect with IL-12 and VEGFR2 on angiogenesis and immune regulation, we designed and constructed a new type of DNA vaccine that can express VEGFR2 and the prostate cancer antigen IL-12 in the same reading frame. The results showed that our DNA vaccine could induce both a humoral immune response and a cellular immune response in mice. Importantly, the immune response induced by our fusion gene was significantly higher than the response induced by VEGFR2 alone. The tumour growth inhibition rate in tumour-bearing mice treated with the pVAX1-VEGFR2-F2A-IL-12 DNA vaccine was significantly higher than that in pVAX1-VEGFR2 treated mice. Consistent with this, the tumour weights of the mice in the pVAX1-VEGFR2-F2A-IL-12 treatment group were significantly less than those of the mice in the pVAX1-VEGFR2 treatment group. Based on these results, we conclude that the anti-tumour effect of VEGFR2 combined with IL-12 is greater than that of VEGFR2 alone. Overall, our study demonstrates that a plasmid co-expressing VEGFR2 and IL-12 has strong potential as a DNA vaccine for use in anti-tumour therapy.
Disclosure statement
The authors declare no conflict of interest.
Additional information
Funding
References
- Yang B, Jeang J, Yang A, et al. DNA vaccine for cancer immunotherapy. Hum Vaccin Immunother. 2014;10(11):3153–3164.
- Pol J, Bloy N, Obrist F, et al. Trial Watch: DNA vaccines for cancer therapy. Oncoimmunology [ Internet]. 2014 [ cited 2016 Jan 3];3(1):e28185. Available from: http://dx.doi.org/10.4161/onci.23803.
- Williams JA. Improving DNA vaccine performance through vector design. Curr Gene Ther. 2014;14(3):170–189.
- Saade F, Petrovsky N. Technologies for enhanced efficacy of DNA vaccines. Expert Rev Vaccines. 2012;11(2):189–209.
- Walther W, Schlag PM. Current status of gene therapy for cancer. Curr Opin Oncol. 2013;25(6):659–664.
- Chiarella P, Fazio VM, Signori E. Electroporation in DNA vaccination protocols against cancer. Curr Drug Metab. 2013;14(3):291–299.
- Khan KH. DNA vaccines: roles against diseases. Germs. 2013;3(1):26–35.
- Russo G, Mischi M, Scheepens W, et al. Angiogenesis in prostate cancer: onset, progression and imaging. BJU Int. 2012;110(11 Pt C):E794–E808.
- Nakagami H, Morishita R. Development of DNA vaccines as an anti-hypertensive therapy or for anti-angiogenesis. Expert Opin Biol Ther. 2015;15(3):431–436.
- Hu Q, Wu M, Fang C, et al. Engineering nanoparticle-coated bacteria as oral DNA vaccines for cancer immunotherapy. Nano Lett. 2015;15(4):2732–2739.
- Wang Z, Dabrosin C, Yin X, et al. Broad targeting of angiogenesis for cancer prevention and therapy. Seminars Cancer Biol. 2015;35(suppl):S224–S243.
- Vasudev NS, Reynolds AR. Anti-angiogenic therapy for cancer: current progress, unresolved questions and future directions. Angiogenesis. 2014;17(3):471–494.
- Lucas ML, Heller L, Coppola D, et al. IL-12 plasmid delivery by in vivo electroporation for the successful treatment of established subcutaneous B16.F10 melanoma. Mol Ther. 2002;5(6):668–675.
- Dabrowska A, Giermasz A, Golab J, et al. Potentiated antitumor effects of interleukin 12 and interferon alpha against B16F10 melanoma in mice. Neoplasma. 2001;48(5):358–361.
- Greten TF, Wang XW, Korangy F. Current concepts of immune based treatments for patients with HCC: from basic science to novel treatment approaches. Gut. 2015;64(5):842–848.
- Cheever MA, Higano CS. PROVENGE (Sipuleucel-T) in prostate cancer: the first FDA-approved therapeutic cancer vaccine. Clin Cancer Res. 2011;17:3520–3526.
- Wang Y, Zhang J, Wu Y, et al. Mannan-modified adenovirus targeting TERT and VEGFR-2: a universal tumour vaccine. Sci Rep [Internet]. 2015 [ cited 2016 Jun 23];5:11275. Available from: http://www.ncbi.nlm.nih.gov/pmc/articles/PMC4471666/.
- Tagliamonte M, Petrizzo A, Tornesello ML, et al. Antigen-specific vaccines for cancer treatment. Hum Vaccin Immunother. 2014;10(11):3332–3346.
- Gardlik R, Behuliak M, Palffy R, et al. Gene therapy for cancer: bacteria-mediated anti-angiogenesis therapy. Gene Ther. 2011;18:425–431.
- Niethammer AG, Lubenau H, Mikus G, et al. Double-blind, placebo-controlled first in human study to investigate an oral vaccine aimed to elicit an immune reaction against the VEGF-Receptor 2 in patients with stage IV and locally advanced pancreatic cancer. BMC Cancer [ Internet]. 2012 [ cited 2015 Dec 20];12:361. Available from: http://dx.doi.org/10.1186/1471-2407-12-361.
- Xu WW, Li B, Lam AK, et al. Targeting VEGFR1- and VEGFR2-expressing non-tumor cells is essential for esophageal cancer therapy. Oncotarget. 2015;6(3):1790–1805.
- Zhao X, Bose A, Komita H, et al. Vaccines targeting tumor blood vessel antigens promote CD8+ T cell-dependent tumor eradication or dormancy in HLA-A2 transgenic mice. J Immunol. 2012;188(4):1782–1788.
- Maranda E, Robak T. Biological properties and clinical significance of interlenkins12(IL-2). Postepy Hig Med Dosw. 1998;52:489–506.
- Li S, Zhang X, Xia X. Regression of tumor growth and induction of long-term anti-tumor memory by interleukin-l2 electro-gene therapy. J Natl Cancer Inst. 2002;94(10):762–768.