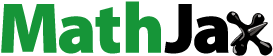
ABSTRACT
In South Africa, the information about the distribution and activity of anaerobic ammonium oxidizing (anammox) bacteria is very limited and anammox systems have not yet been tested and implemented. In this study, the existence and diversity of the anammox bacteria from various South African habitats are investigated. Batch systems were used to enrich anammox biomass from sludge collected from three Pretoria municipal wastewater treatment works. Anammox activity was tested and detected in two of the three wastewater treatment works after 90 days of primary enrichment. The activity was confirmed by consumption of both NH4 + and NO2 − in the system. The presence of anammox bacteria was also confirmed by polymerase chain reaction (PCR) amplification of the 16s rRNA of the anammox using anammox-specific primers. All clones retrieved were closely related to Brocadia species and were most abundant in all habitats tested. The maximum growth rate of anammox was also estimated on both experiments using a relatively new model.
Introduction
One of the legacies of the current dominance of the human kind on earth is the evident impact on nitrogen, phosphorous and sulphur cycles. Latest reports indicate that human activities such as increased combustion of fossil fuels and increased demand for nitrogen in agriculture and industry continue to alter the global cycle of nitrogen [Citation1,Citation2]. Because of the increased nitrogen mobility, excess nitrogen from human activities has serious and ongoing environmental consequences for aquatic and terrestrial ecosystems [Citation2].
It is extremely important to remove nitrogen to meet minimum disposal requirements during wastewater treatment because of its contribution to the eutrophication of receiving waters, and the toxicity and direct threat it poses to aquatic life [Citation3]. The nitrogen in wastewater is presented mainly as ammonium (NH4 +) [Citation4], which can be removed by physico-chemical or biological processes [Citation5]. Currently, there are several methods that are used for removal of nitrogen from wastewater. For example, physical membrane processes or ion exchange can be used to remove ammonium using a cation exchange resin [Citation6–9]. The disadvantage of the physical processes is that, in both cases, a highly concentrated brine or a highly acidified effluent stream is produced which requires further neutralization before disposal. On the other hand, biological processes including nitrosification--nitration--denitrification can be employed as a cleaner process [Citation10–13]. Biological nitrogen removal is considered less expensive and more effective than physico-chemical treatments and thus has been used more often to achieve nitrogen removal from domestic wastewaters [Citation14].
The conventional biological nitrogen removal processes are generally used for treating wastewaters with quite low nitrogen concentrations (concentration less than 100 mg N/l) [Citation4]. Some wastewater streams consist of high concentrations of nitrogen, mostly in the form of ammonium. If these streams are returned back to the inlet of the municipal WWTP, they increase the ammonium loading in the mainstream. Conventional biological nitrogen-removal process (denitrification--nitrification) is uneconomical and complicated when treating high nitrogen-containing wastewaters with low C/N ratio. During the last decade, several new sustainable and cost-effective alternatives have been discovered and studied, and their implementation can be a valid option to treat strong nitrogenous wastewaters characterized by high ammonium concentrations and low biodegradable organic matter content. Currently, anammox process is considered to have potential for treating wastewaters with high ammonium concentrations [Citation15,Citation16].
The anammox (anaerobic ammonium oxidation) process is novel and promising alternative nitrogen-removing technique in which ammonium is directly oxidized to dinitrogen gas using nitrite as the electron acceptor under anoxic conditions [Citation17]. For up until the 1990s, ammonium oxidation was thought to only take place under aerobic conditions [Citation18,Citation19]. Over two decades ago, Broda [Citation20] predicted the existence of chemolitoautotrophic bacteria capable of oxidizing ammonium using nitrite as electron acceptor on the basis of thermodynamic calculations. The predictions were experimentally corroborated by Mulder et al. [Citation21] two decades later in a denitrifying fluidized-bed reactor treating effluent from a yeast plant. That reactor removed an ammonium loading rate of 0.4 g NH4+−N·L−1·d−1 by this process. By nitrogen balances, they hypothesized the following stoichiometry and called the process Anammox:(1)
(1) Strous.et al. [Citation22] optimized the process conditions and found the following global equation of the process, based on mass balance over anammox-enriched cultures:
(2)
(2)
Ever since the anammox process was discovered in Delft and the first anammox organism, Candidatus Brocadia Anammoxidans, was identified, many more studies have reported the presence of anammox in natural environments such as marine sediments [Citation23–25], marine sponges [Citation26], estuarine/tidal river sediments [Citation27–30], deep-sea hydrothermal vents [Citation31], hot springs [Citation32] and some freshwater ecosystems [Citation33–36]. Consequently, new species other than Candidatus Brocadia Anammoxidans were discovered and identified and their 16srRNA were determined. These were Candidatus Kuenenia stuttgartiensis, Candidatus Scalindua sorokinii, Candidatus Scalindua brodae, Candidatus Scalindua wagneri, Candidatus Brocadia fulgida and Candidatus Anammoxoglobuspropionicus [Citation24,Citation37–39]. Over the past years, the main focus has been on shortening the start-up period and enhancing the activity of anammox bacteria. In an effort to do this, Li et al. [Citation40,Citation41] used a novel osmotic anammox system, where anammox was linked to forward osmosis to remove nitrogen. On the other hand, Yin et al. [Citation42] added reduced graphene oxides into an anammox reactor in order to accelerate anammox growth and enhance the activity and simultaneously improve the start-up period.
At the inception of this study, very little information was available regarding the distribution, diversity and abundance of anammox bacteria in the South African wastewater treatment plants. Therefore, the principal aim of this study was to investigate the extent of existence, culture community analysis and location of anammox activity in selected wastewater treatment plants.
Materials and methods
Sample collection
Wastewater samples were collected from three wastewater treatment plants in Pretoria, namely Daspoort, Baviaanspoort and Zeekoegat wastewater treatment plants. From Zeekoegat and Baviaanspoort, the sludge samples were collected from both anoxic and anaerobic zones of the second stage of the activated sludge system. This was done by lowering a sampling jug with an extended handle of about 1--1.5 m in length into the reactor. The sludge samples were then transferred to 1-L plastic containers. From Daspoort, the sludge samples were taken from an anaerobic digester from the middle (anoxic) and the bottom (anaerobic) part of the digester. This was done by opening the valve in the middle and bottom parts of the digester and collecting the samples into plastic containers. At Daspoort, the samples were also taken from the trickling filter by taking stones covered with biofilm and were placed in plastic sampling containers. The length from which the stones were taken was not measured but they were only sampled based on the attached growth. All the samples were stored at room temperature and cultured within 24 h of collection. The remaining samples were stored at 4 °C for future reference.
Experimental set-up
A series of batch reactors were set up and used for cultivation and enrichment of anammox bacteria. Each reactor was a 500-mL serum bottle containing 300 mL of simulated wastewater with the composition adapted from Jetten et al. [Citation43] ( and ). Each reactor was inoculated with 20% of different activated sludge samples from different wastewater treatment plants. To get rid of oxygen from the reactors and create anaerobic conditions, the reactors were purged with argon gas for 15--20 min before sealing with rubber septa and aluminium crimp seals. The reactors (Sigma Aldrich, Johannesburg, South Africa) were then shaken on a rotary shaker (Lasec, Johannesburg, South Africa) or stirred on a magnetic stirrer (Lasec) at a speed of 80–100 rpm at a temperature of 31 ± 2 °C in the dark controlled room. After 60 d of incubation, the synthetic medium was replaced with the freshly prepared one to replace the depleted nutrients. After 90 d of incubation, experiments to test anammox activity were conducted in small 500-mL reactors using the biomass from the primary enrichment reactors as the inoculum.
Table 1. Composition of anammox mineral medium.
Table 2. Composition of trace elements solution.
Analytical methods
Ammonium, nitrite and nitrate were analysed calorimetrically according to the following methods:
Nitrate analysis – 10 µL saturated sulfumic acid and 40 µL reactor effluent were added together. To the mixture, a total of 0.2 mL reagent was added containing 5% salicylic acid in 98% sulphuric acid and 2 mL 4 M NaOH (4 °C). This solution was analysed in a spectrophotometer at 420 nm after a 30-min reaction.
Ammonium analysis – 760 µL of a solution was added containing 0.54% ortho-pthalaldehyde, 0.05% β-mercaptoethanol and 10% ethanol in 400 mmol L−1 potassium phosphate buffer (pH 7.3) to a 40 µL reactor effluent sample. This solution is analysed in a spectrophotometer at 420 nm after a 30-min reaction.
Nitrite analysis – 950 µL of a reagent was added containing 1% sulfanilic acid and 0.05% N-naphthylethylenediamine in 1 mol L−1 H3PO4 to 50 µL of reactor effluent. This is followed by a spectrophotometric analysis at 540 nm after 5-min reaction.
The production of N2 was determined by pressure transducer device as the increment of pressure in the headspace of each reactor.
Detection of anammox by 16s rRNA gene sequencing
To detect the presence of anammox in the reactors, polymerase chain reaction (PCR) amplification targeting the 16s rRNA of the anammox was performed. From the reactors, samples were withdrawn and centrifuged at 6000 rpm for 10 min. Pellets were resuspended in phosphate-buffered saline (pH 7.4) and centrifuged again. DNA was directly extracted from these samples using a ZR Fungal/Bacterial DNA MiniPrep™ (Zymo Research Irvine, CA, USA) according to the manufacturer’s instructions. The PCR primers used in this study are primer sets AMX 368F-AMX 820R and AMX 368F-BS 820R ().
Table 3. PCR primers for anammox-bacteria-related 16s rRNA genes.
The PCR reaction mixture (50 µL) contained 25 µL of DreamTaq™ Green PCR Master Mix (2X) (Fermentas Life Sciences, Canada), 0.1 µmol L−1 of each reverse and forward primer, 1 µg of DNA template and nuclease free water. DreamTaq™ Green PCR Master Mix is a ready-to use solution containing DreamTaq™ DNA polymerase, optimized DreamTaq™ Green buffer, MgCl2 and dNTPs. The thermal cycle program used was an initial denaturation at 95 °C for 5 min, followed by 45 cycles of denaturation at 95 °C for 30 sec, annealing at 50 °C for 30 sec, extension at 72 °C for 2 min, final extension at 72 °C for 10 min and hold at 4 °C. The PCR products were recovered and purified using ExoSAP amplicon purification kit (Inqaba Biotech, Pretoria, South Africa) according to manufacturer's instructions. Purified DNA fragment were ligated to pJET1.2/blunt cloning vector (Thermo Scientific Waltham, MA, USA) and 16s rRNA gene clone libraries were constructed by transforming Premade Z-Competent TM E. coli Cells (Zymo Research) with a vector.
Sequencing was carried out with the BigDye Terminator v3.1 Cycle Sequencing Kit (Applied Biosciences, Carlsbad, CA, USA). Cleaned sequencing products were analysed on the ABI 3500XL genetic analyser (Applied Biosciences) using a 50-cm array and POP7. For phylogenetic analysis, 16S rRNA sequences, they were compared with available database sequences via NCBI-BLAST search, and the related taxa were obtained from GenBank. The multiple alignments were performed with the CLUSTAL X program [Citation44]. Sequences of 16S rRNA gene were edited with the BioEdit program [Citation45]. The phylogenetic trees were constructed via the neighbour-joining and maximum-parsimony algorithms with the MEGA 3 program [Citation46]. The similarity of the rRNA gene sequences of the clones was compared with that of other known anammox species.
Model development
The model was initially developed for determining the maximum specific growth rate for autotrophic bacteria in nitrification [Citation47]. The model was confirmed by Melcer [Citation48] and termed it high F/M model. In the present study, the model was adopted and modified to best describe the anammox process. The model has the assumption that nitrite consumption is the best signal for anammox growth. A mathematical expression was then derived to correlate nitrite consumption to biomass (anammox) growth rate.
Biological reactions
In the calculations of the maximum growth rate of anammox (μAn), it was assumed that the consumption of nitrite was related to anammox growth. The rate expression to correlate nitrite consumption to biomass production was as follows:(3)
(3)
In the case where S
NH is high enough not to be a rate limiting (i.e. in high F/M ratio), EquationEquation (3)(3)
(3) may be simplified as
(4)
(4)
For this condition, the growth of anammox biomass can be expressed as(5)
(5)
In batch reactors, the concentration of biomass can be calculated by integration of EquationEquation (5)(5)
(5) to obtain the following expression:
(6)
(6)
Substituting EquationEquation (6)(6)
(6) into (Equation4
(4)
(4) ) gives
(7)
(7)
Integration of EquationEquation (7)(7)
(7) gives the following expression:
(8)
(8)
Model simulation
The simulation was performed using a computer program for identification and simulation of aquatic systems (AQUASIM 2.0) [Citation49]. Batch experiments of cultures obtained from two wastewater treatment works (Daspoort and Zeekoegat) were conducted to evaluate the rate equations of kinetic constants for processes taking place in the batch reactors. The performance of the mathematical model and the utility of different parameters were then evaluated by simulating the biotransformation of nitrogen compounds by anammox bacteria.
Results and discussions
Enrichment of anammox biomass
Various attempts for anammox enrichment were performed from different sludge samples using batch reactors. The sludge samples that were used for starting anammox enrichment were originating from three municipal wastewater treatment plants. A period of 90 days was used for enriching anammox biomass due to slow growth of anammox bacteria. This was done to allow for enough time for anammox growth to large numbers if it was initially present from the sludge samples. The results for anammox activity obtained by calorimetric analysis of ammonia, nitrite and nitrate are presented in –.
Figure 1. Middle reactor represents the results for the reactor inoculated with Daspoort sample collected from the middle part of an anaerobic digester.
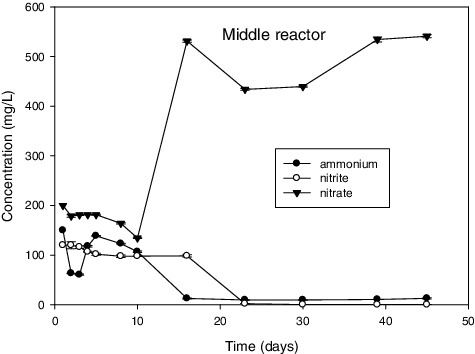
Figure 2. Zeekoegaat anoxic represents results from reactor inoculated with sludge samples obtained from the anoxic zone of the secondary stage of Zeekoegaat treatment plant.
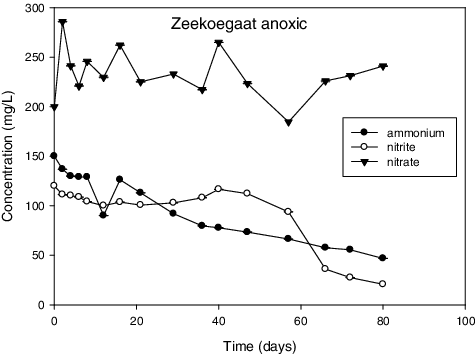
Figure 3. Control reactor represents the results for the reactor that was not inoculated with sludge.
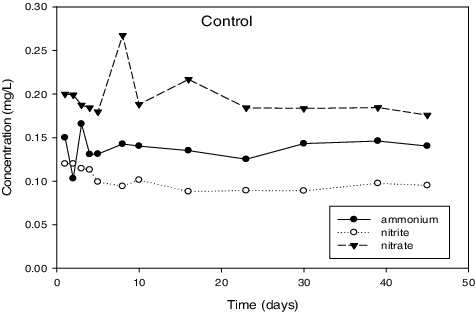
and illustrate the results with successful enrichment obtained after enrichment. First, simultaneous ammonium and nitrite removal was observed in these reactors. Additionally, an increased production of nitrate was also observed at the same time. There was no consumption of ammonia and nitrite observed in the rest of tested samples for the duration of experiments (results not shown). This observation suggested the absence of anammox activity in these reactors. There was also no significant change in the control reactor () where there were no cells added. Out of the seven enrichment attempts that were performed, only three were successful.
Notably, because the cultures were pre-enriched before testing the anammox activity, it took only 22 d for complete consumption of the substrates in Daspoort. On the contrary, when Dapena‐Mora [Citation50] was enriching anammox using municipal sludge, it took two months of reactor operation just to observe noticeable consumption of NH4 + and NO2 −. Recently, it took 40 d for Chen et al. [Citation51] to start up a reactor using a pre-enriched mixed denitrifying-anammox sludge. However, it took just above 80 d for the Zeekoegaat culture for complete consumption. This was attributed to low anammox biomass concentration and confirmed by PCR results which showed a very faint positive band on a gel (results not shown). Furthermore, Qin et al. [Citation52] reported that high concentration of anammox bacteria translate into higher rate of nitrogen conversion.
Anammox was also detected in a trickling filter sample and was only confirmed by PCR. These results are in accordance with those reported by Schmid et al. [Citation37], who also detected anammox bacteria with high anaerobic ammonium oxidation activity in a trickling filter. It was noteworthy that enrichment was observed mostly from samples that were collected from anoxic conditions. This observation could be a characteristic associated with the favourable conditions of anammox bacteria. A summary of all enrichment experiments is shown in .
Table 4. Summary of enrichment experiments.
PCR identification and phylogenetic analysis
After a successful enrichment was obtained, PCR was performed using anammox-specific primer sets Amx368F-Amx820R and Amx368F-BS820R. The PCR for the middle, trickling filter and Zeekoegaat anoxic reactors’ DNA extracts resulted in positive amplicons for both primer sets (results not shown). These results were the second confirmation of the presence of anammox from the two reactors including the trickling filter. On the other hand, no positive results were observed for all other reactors.
Sequencing of the cloned 16s rRNA fragments resulted in the detection of sequences which were highly similar to known anammox bacteria. and are showing the phylogenetic trees obtained from the sequencing of the retrieved clones. These results showed that the anammox bacteria from an anaerobic digester (middle reactor), trickling filter and Zeekoegaat WWTP were all closely related to Brocadia species (fulgida, anmmoxidans and caroliniensis) ( and ). Previously, Hu et al. [Citation53] enriched Brocadia fulgida from a peat soil. Correspondingly, Sánchez Guillén et al. [Citation54] found that B. fulgida was the dominant anammox strain throughout the long-term operation of a sequencing batch reactor seeded with granular sludge for sewage treatment. Dapena‐Mora [Citation50] likewise performed anammox enrichment from municipal activated sludge and found Kuenenia stuttgartiensis as major anammox species in the enrichment culture. On the contrary, Schmid et al. [Citation37] isolated K. stuttgartiensis from the trickling filter biofilm. This indicates that each anammox species can occupy diverse ecological niches. There is still no well-defined niche definition for the anammox species. However, there are reports that Brocadia and Kuenenia species are commonly found in wastewater treatment plants and bioreactors [Citation55]. Based on the phylogenetic tree analysis, it was clear that the South African wastewater treatment plants (Pretoria region) were dominated by Brocadia species.
Model calibration
During model calibration, certain parameter values of the model are adjusted in order to generate the results that are in agreement with the experimental data. In the present study, the calibration approach was to fit the model simulation data on the nitrite experimental data obtained during batch experiments. The maximum growth rate of anammox was determined by performing a nonlinear regression analysis on EquationEquation (8)(8)
(8) using AQUASIM program. By using EquationEquation (8)
(8)
(8) and the experimental data, three other biokinetic coefficients (b
An, X
An,0 and Y
An) were also predicted.
Estimation of maximum growth rate
The maximum growth rate values predicted by the model are presented in . The growth rate for Daspoort and Zeekoegaat cultures were estimates as 0.101 and 0.023 h−1, respectively. These values are considerably higher than those reported in. [Citation22,Citation56]. Although the maximum growth rates for the present study were higher than those in literature, the culture from Zeekoegaat had lower growth rate than the culture from Daspoort. Strous et al. [Citation22] attributed the low anammox growth rate to low substrate consumption rate. This was confirmed in the present study as it took more than 80 d for nitrite to be completely consumed with the Zeekoegaat culture while it took only 45 d for nitrite consumption with the Daspoort culture. This means that the low growth rate for Zeekoegaat was due to low nitrite consumption rate.
Table 5. Estimation of maximum growth rate using high F/M growth model.
The yield coefficients for anammox biomass were calculated to be 9.9 mg biomass mg−1 N and 9.5 mg biomass mg−1 N whereas the decay rate coefficients were 0.0049 and 0.0003 d−1 for Daspoort and Zeekoegaat, respectively. Based on these values, the anammox concentration was calculated to be 8 mg L−1 on both experiments ().*
In assessing the validity of the model, the model predictions were compared to measured experimental data. According to and , the model predicted values fit to measured experimental data quit well for Daspoort and Zeekoegaat experiments, respectively. However, there was a bit of deflection in the Zeekoegaat experiment () due to instability of the reactor at the beginning of the experiment. The good agreement between the model and measured data is evidently demonstrating the validity of the model developed in this study. Therefore, this model is suitable for estimating maximum growth rate for anammox in batch experiments.
Conclusions
The study provided evidence for the existence of anammox bacteria in some of Gauteng wastewater treatment plants. The study also provided the information of the possibility of wide distribution of anammox with little diversity as it was found out that the habitats are dominated by only Brocadia species. The maximum growth rate of anammox bacteria was determined to be higher than previously reported in literature.
Disclosure statement
No potential conflict of interest was reported by the authors.
Additional information
Funding
References
- Galloway JN , Townsend AR , Erisman JW , et al. Transformation of the nitrogen cycle: recent trends, questions, and potential solutions. Science. 2008;320(5878):889–892.
- Vitousek PM , Aber JD , Howarth RW , et al. Human alteration of the global nitrogen cycle: sources and consequences. Ecol Appl. 1997;7(3):737–750.
- Daims H , Taylor MW , Wagner M . Wastewater treatment: a model system for microbial ecology. Trends Biotechnol. 2006;24(11):483–489.
- Van Hulle SWH , Vandeweyer HJP , Meesschaert BD , et al. Engineering aspects and practical application of autotrophic nitrogen removal from nitrogen rich streams. Chem Eng J. 2010;162(1):1–20.
- Zhang L , Zheng P , Tang CJ , et al. Anaerobic ammonium oxidation for treatment of ammonium-rich wastewaters. J Zhejiang Uni Sci B. 2008;9(5):416–426.
- Bódalo A , Gómez J , Gómez E , et al. Ammonium removal from aqueous solutions by reverse osmosis using cellulose acetate membranes. Desalination. 2005;184(1–3):149–155.
- Jorgensen TC , Weatherley LR . Ammonia removal from wastewater by ion exchange in the presence of organic contaminants. Water Res. 2003;37(8):1723–1728.
- Lahav O , Green M . Ammonium removal using ion exchange and biological regeneration. Water Res. 1998;32(7):2019–2028.
- Sarioglu M . Removal of ammonium from municipal wastewater using natural Turkish (Dogantepe) zeolite. Sep Purif Technol. 2005;41(1):1–11.
- Peng Y , Zhu G . Biological nitrogen removal with nitrification and denitrification via nitrite pathway. Appl Microbiol Biotechnol. 2006;73(1):15–26.
- Park JY , Yoo YJ . Biological nitrate removal in industrial wastewater treatment: which electron donor we can choose. Appl Microbiol Biotechnol. 2009;82(3):415–429.
- Bertanza G . Simultaneous nitrification–denitrification process in extended aeration plants: pilot and real scale experiences. Water Sci Technol. 1997;35(6):53–61.
- Mahne I , Prinčič A , Megušar F. Nitrification/denitrification in nitrogen high-strength liquid wastes. Water Res. 1996;30(9):2107–2111.
- Khin T , Annachhatre AP . Novel microbial nitrogen removal processes. Biotechnol Adv. 2004;22(7):519–532.
- He S , Zhang Y , Yang M , et al. Repeated use of MAP decomposition residues for the removal of high ammonium concentration from landfill leachate. Chemosphere. 2007;66(11):2233–2238.
- Ma B , Zhang S , Zhang L , et al. The feasibility of using a two-stage autotrophic nitrogen removal process to treat sewage. Bioresour Technol. 2011;102(17):8331–8334.
- Strous M , Van Gerven E , Zheng P , et al. Ammonium removal from concentrated waste streams with the anaerobic ammonium oxidation (anammox) process in different reactor configurations. Water Res. 1997;31(8):1955–1962.
- Fux C , Boehler M , Huber P , et al. Biological treatment of ammonium-rich wastewater by partial nitritation and subsequent anaerobic ammonium oxidation (anammox) in a pilot plant. J Biotechnol. 2002;99(3):295–306.
- Jetten MSM , Wagner M , Fuerst J , et al. Microbiology and application of the anaerobic ammonium oxidation (‘anammox’) process. Curr Opin Biotechnol. 2001;12(3):283–288.
- Broda E . Two kinds of lithotrophs missing in nature. Z Allg Mikrobiol. 1977;17(6):491–493.
- Mulder A , van de Graaf AA , Robertson LA , et al. Anaerobic ammonium oxidation discovered in a denitrifying fluidized bed reactor. FEMS Microbiol Ecol. 1995;16(3):177–183.
- Strous M , Heijnen J , Kuenen J , et al. The sequencing batch reactor as a powerful tool for the study of slowly growing anaerobic ammonium-oxidizing microorganisms. Appl Microbiol Biotechnol. 1998;50(5):589–596.
- Dalsgaard T , Canfield DE , Petersen J , et al. N2 production by the anammox reaction in the anoxic water column of Golfo Dulce, Costa Rica. Nature. 2003;422(6932):606–608.
- Kuypers MMM , Sliekers AO , Lavik G , et al. Anaerobic ammonium oxidation by anammox bacteria in the Black Sea. Nature. 2003;422(6932):608–611.
- Thamdrup B , Dalsgaard T . Production of N2 through anaerobic ammonium oxidation coupled to nitrate reduction in marine sediments. Appl Environ Microbiol. 2002;68(3):1312–1318.
- Mohamed NM , Saito K , Tal Y , et al. Diversity of aerobic and anaerobic ammonia-oxidizing bacteria in marine sponges. ISME J. 2009;4(1):38–48.
- Trimmer M , Nicholls JC , Deflandre B . Anaerobic ammonium oxidation measured in sediments along the Thames estuary, United Kingdom. Appl Environ Microbiol. 2003;69(11):6447–6454.
- Rysgaard S , Glud RN , Risgaard-Petersen N , et al. Denitrification and anammox activity in Arctic marine sediments. Limnol Oceanogr. 2004;49(5):1493–1502.
- Meyer RL , Risgaard-Petersen N , Allen DE . Correlation between anammox activity and microscale distribution of nitrite in a subtropical mangrove sediment. Appl Environ Microbiol. 2005;71(10):6142–6149.
- Dale OR , Tobias CR , Song B . Biogeographical distribution of diverse anaerobic ammonium oxidizing (anammox) bacteria in Cape Fear River Estuary. Environ Microbiol. 2009;11(5):1194–1207.
- Byrne N , Strous M , Crépeau V , et al. Presence and activity of anaerobic ammonium-oxidizing bacteria at deep-sea hydrothermal vents. ISME J. 2008;3(1):117–123.
- Jaeschke A , Op den Camp HJM , Harhangi H , et al. 16S rRNA gene and lipid biomarker evidence for anaerobic ammonium‐oxidizing bacteria (anammox) in California and Nevada hot springs. FEMS Microbiol Ecol. 2009;67(3):343–350.
- Schubert CJ , Durisch‐Kaiser E , Wehrli B , et al. Anaerobic ammonium oxidation in a tropical freshwater system (Lake Tanganyika). Environ Microbiol. 2006;8(10):1857–1863.
- Robert Hamersley M , Woebken D , Boehrer B , et al. Water column anammox and denitrification in a temperate permanently stratified lake (Lake Rassnitzer, Germany). Syst Appl Microbiol. 2009;32(8):571–582.
- Penton CR , Devol AH , Tiedje JM . Molecular evidence for the broad distribution of anaerobic ammonium-oxidizing bacteria in freshwater and marine sediments. Appl Environ Microbiol. 2006;72(10):6829–6832.
- Zhang Y , Ruan XH , Op den Camp HJM , et al. Diversity and abundance of aerobic and anaerobic ammonium‐oxidizing bacteria in freshwater sediments of the Xinyi River (China). Environ Microbiol. 2007;9(9):2375–2382.
- Schmid M , Twachtmann U , Klein M , et al. Molecular evidence for genus level diversity of bacteria capable of catalyzing anaerobic ammonium oxidation. Syst Appl Microbiol. 2000;23(1):93–106.
- Kartal B , Rattray J , van Niftrik LA , et al. Candidatus ‘Anammoxoglobus propionicus’ a new propionate oxidizing species of anaerobic ammonium oxidizing bacteria. Syst Appl Microbiol. 2007;30(1):39–49.
- Kartal B , Van Niftrik L , Rattray J , et al. Candidatus ‘Brocadia fulgida’: an autofluorescent anaerobic ammonium oxidizing bacterium. FEMS Microbiol Ecol. 2007;63(1):46–55.
- Li X , Sun S , Badgley BD , et al. Long-term performance and microbial community characterization of an osmotic anammox system for removing reverse-fluxed ammonium. Bioresour Technol. 2016;211:628–635.
- Li X , Lu Y , He Z . Removal of reverse-fluxed ammonium by anammox in a forward osmosis system using ammonium bicarbonate as a draw solute. J Membr Sci. 2015;495:424–30.
- Yin X , Qiao S , Zhou J , et al. Fast start-up of the anammox process with addition of reduced graphene oxides. Chem Eng J. 2016;283:160–166.
- Jetten M , Schmid M , van de Pas‐Schoonen K , et al. Anammox organisms: enrichment, cultivation, and environmental analysis. Meth Enzymol. 2005;397:34–57.
- Thompson JD , Gibson TJ , Plewniak F , et al. The CLUSTAL_X windows interface: flexible strategies for multiple sequence alignment aided by quality analysis tools. Nucleic Acids Res. 1997;25(24):4876–4882.
- Hall TA. BioEdit: a user-friendly biological sequence alignment editor and analysis program for Windows 95/98/NT. Nucleic Acids Symp Ser. 1999;41:95–98.
- Kumar S , Tamura K , Nei M . MEGA3: integrated software for Molecular Evolutionary Genetics Analysis and sequence alignment. Brief Bioinform. 2004;5(2):150–163.
- Sözen S , Orhon D , San H . A new approach for the evaluation of the maximum specific growth rate in nitrification. Water Res. 1996;30(7):1661–1669.
- Melcer H . Methods for wastewater characterization in activated sludge modeling. London: IWA Publishing; 2003.
- Reichert P , Ruchti J , Simon W . AQUASIM 2.0. Duebendorf: Swiss Federal Institute for Environmental Science and Technology (EAWAG); 1998.
- Dapena‐Mora A , Van Hulle SWH , Luis Campos J , et al. Enrichment of anammox biomass from municipal activated sludge: experimental and modelling results. J Chem Technol Biotechnol. 2004;79(12):1421–1428.
- Chen H , Hu H , Chen Q , et al. Successful start-up of the anammox process: influence of the seeding strategy on performance and granule properties. Bioresour Technol. 2016;211:594–602.
- Qin Y , Zhou S . Enrichment and molecular diversity of anammox bacteria in UASB reactor. Environ Prot Eng. 2009;35(3):17–26.
- Hu BL , Rush D , van der Biezen E , et al. New anaerobic, ammonium-oxidizing community enriched from peat soil. Appl Environ Microbiol. 2011;77(3):966–971.
- Sánchez Guillén JA , Lopez Vazquez CM , de Oliveira Cruz LM , et al. Long-term performance of the anammox process under low nitrogen sludge loading rate and moderate to low temperature. Biochem Eng J. 2016;110:95–106.
- Schmid MC , Maas B , Dapena A , et al. Biomarkers for in situ detection of anaerobic ammonium-oxidizing (anammox) bacteria. Appl Environ Microbiol. 2005;71(4):1677–1684.
- Van de Graaf AA , de Bruijn P , Robertson LA , et al. Autotrophic growth of anaerobic ammonium-oxidizing micro-organisms in a fluidized bed reactor. Microbiology. 1996;142(8):2187–2196.