ABSTRACT
Aspergillus chevalieri var. intermedius is a dominant filamentous fungal species in Fuzhuan tea and is associated with the quality and health benefits of this tea. The sexual or asexual reproduction of this fungus depends on the osmotic pressure of the tea. Efforts to enhance the beneficial effects of A. chevalieri var. intermedius are hampered by difficulties in disrupting its genes. To address this issue, we identified the A. chevalieri var. intermedius homolog (Acku70) of human Ku70 and generated an Acku70 disruption strain (ΔAcku70), aiming to improve the gene replacement efficiency. ΔAcku70 grew at a slightly lower rate in vitro than the wild-type strain; however, the two strains exhibited similar sensitivity to temperature, osmotic pressure and the effects of ethyl methane sulphonate and H2O2. The replacement efficiency of veA and flbA dramatically increased in ΔAcku70 compared to that in the wild type. The efficiency of flbA replacement increased from 2.6% to 80%, whereas the frequency of veA disruption increased from 15.2% to 83.9% and from 30.8% to 86.8%. Thus, ΔAcku70 is suitable for use as a type strain for large-scale functional genomic analysis of A. chevalieri var. intermedius.
Introduction
Fuzhuan tea, a microbial fermentation product, is traditionally produced mainly in the Hunan Province of China and is a popular beverage of the inhabitants of the border regions of southern and western China [Citation1]. Aspergillus chevalieri var. intermedius is the dominant filamentous species in Fuzhuan tea and is associated with the quality and health benefits of the tea, including lowering lipid levels, improving digestion and antitumour activities [Citation2,Citation3]. A. chevalieri var. intermedius is a homothallic species with distinctive modes of sexual and asexual reproduction that are regulated by osmotic pressure [Citation4]. Liu et al. [Citation4] discussed the impact of sexual spores and asexual spores of A. chevalieri var. intermedius in dealing with different concentrations of sucrose and NaCl, different temperature and different culture media. The conidia formation increases as the sucrose concentration increases, whereas the amount of cleistothecia gradually decreases with the increase in sucrose concentration. The number of conidia of A. chevalieri var. intermedius also increases, the higher the NaCl concentration is [Citation4]. This indicates that A. chevalieri var. intermedius is a good model to study the process of conidia and ascospore development, since it can form different kinds of spores under different osmotic pressure. Thus, molecular genetic characterization of A. chevalieri var. intermedius would not only benefit the Fuzhuan tea industry, but would also shed light on the mechanism of sexual or asexual reproduction of filamentous fungi.
Our laboratory has been working on A. chevalieri var. intermedius genome and transcriptome sequencing, and large-scale gene knockout research work is to be undertaken. Agrobacterium tumefaciens-mediated transformation (ATMT) was achieved and a suppression subtractive hybridization library of A. chevalieri var. intermedius was successfully constructed [Citation5,Citation6]; several genes were cloned and analysed using gene disruption [Citation5]. However, the gene replacement efficiency in A. chevalieri var. intermedius is low.
Exogenous DNA fragments and gene replacement cassettes contain double-strand breaks (DSBs); the DSB repair mechanism integrates them into cellular genomes. The two main pathways involved in classical DSB repair mechanism are homologous recombination (HR) and non-homologous end joining (NHEJ) [Citation7]. In the HR pathway, DSBs’ integration into chromosomes occurs at regions with sequence homology [Citation8], whereas in the NHEJ pathway, DSBs randomly integrate into chromosomes independent of sequence homology. HR and NHEJ are independent and competitive [Citation9]. For example, Saccharomyces cerevisiae preferentially uses HR with highly efficient gene replacement for repairing DSBs [Citation10,Citation11]. Conversely, mammals, plants and filamentous fungi preferentially use NHEJ and, thus, have low gene replacement efficiency [Citation12].
The principal proteins involved in the mammalian NHEJ pathway are Ku, DNA-PKcs (DNA-dependent protein kinase catalytic subunit), DNA ligase IV, XRCC4 and Artemis [Citation9]. Ku, a heterodimer comprising Ku70 and Ku80, combines DSB ends and then recruits DNA-PKcs and Artemis. The DNA-PKcs–Artemis complex results in the DNA ligase IV–XRCC4 complex recruitment to the DNA ends, which ligates DSBs [Citation13]. Compared with Rad52 paralogs in the HR pathway, Ku serves as a ‘gatekeeper’ of recombination and can suppress DSB repair through other pathways [Citation9,Citation12]. Deleting ku in fungi increases the gene replacement efficiency as first shown in yeast studies and subsequently in other fungi, including Neurospora crassa [Citation14], Aspergillus fumigatus [Citation15], Aspergillus nidulans [Citation16], Aspergillus niger [Citation17], Magnaporthe grisea [Citation18], Penicillium chrysogenum [Citation19] and Penicillium decumbens [Citation20]. The growth of ku-deficient mutants in vitro is not significantly different compared with that of wild-type strains. Therefore, ku-deficient strains can be used for genome-wide gene-targeting studies.
Here we hypothesized that homologous deletion of ku70 from A. chevalieri var. intermedius will increase the gene replacement efficiency. Therefore, we isolated A. chevalieri var. intermedius Acku70 and investigated the effects of its deletion mutant ΔAcku70 on the gene replacement efficiency. We demonstrate that ΔAcku70 markedly enhanced the gene replacement efficiency with no apparent defects in the growth in vitro, except for a slightly lower growth rate. Therefore, ΔAcku70 will serve as a useful tool for the genetic analysis of A. chevalieri var. intermedius.
Materials and methods
Fungal strains, medium and growth conditions
We isolated A. chevalieri var. intermedius GZAAS20.1004 from Fuzhuan tea and cultured it in malt yeast extract agar (MYA) or MY broth (MYB) medium at 28 °C [Citation21]. MYA contains 20 g·L−1 malt extract, 5 g·L−1 yeast extract and 30 g·L−1 sucrose. MYB contains 50 g·L−1 sodium chloride, 20 g·L−1 malt extract, 5 g·L−1 yeast extract and 30 g·L−1 sucrose. For conidia formation, fungal mycelia were cultured on solid MYA plates containing 17% (m/v) sodium chloride and incubated for at least seven days at 37 °C. Minimal medium (MM) contained 2.05 g·L−1 K2HPO4, 41.45 g·L−1 KH2PO4, 0.15 g·L−1 NaCl, 0.50 g·L−1 MgSO4.7H2O, 0.1 g·L−1 CaCl2.2H2O, 0.0025 g·L−1 FeSO4.7H2O, 0.5 g·L−1 (NH4)2SO4 and 2.0 g·L−1 sucrose with pH 7.0–8.0. Induction medium (IM) contained 40 mmol L−1 2-(N-morpholino)ethanesulfonic acid, 0.5% (v/v) glycerol and 200 μmol L−1 acetosyringone in MM with pH 5.3. These latter two media were used for genetic transformation.
Cloning of fungal ku70 homolog
The oligonucleotide primers used in this study are listed in . A partial sequence of Acku70 cDNA was amplified using polymerase chain reaction (PCR) from cDNA that was prepared using total fungal RNA as the template with two degenerate primers designated as P1 and P2. The 5′ end of Acku70 cDNA was amplified using a SMARTER cDNA synthesis kit (BD Clontech, Mountain View, CA, USA) with the specific primer P3 and nested primer P4. The 3′ end of Acku70 cDNA was amplified using a SMARTER cDNA synthesis kit with the specific primer P5. A genomic DNA fragment containing the open reading frame (ORF) of Acku70 was amplified using PCR with the specific primers P6 and P7. An additional 0.9-kb sequence flanking the 3′ end of Acku70 was amplified using the specific primer P8, nested primer P9 and degenerate walking primer P26 [Citation22]. The composition of the PCR reaction mixture for genome walking was as follows: total volume of 25 μL, 12.5 μL of 2× PCR Mix Master (Tiangen Biotech, Beijing, China), 1 μL DNA template, 1.5 μL double-distilled H2O and 5 μL of 10 μmol L−1 of each primer (specific primer or nested primer and degenerate walking primer).
Table 1. Oligonucleotide primers.
The software used for primer design was CODEHOP and Primer premier 5.0. Gene sequences were analysed using online databases, such as that of the National Center for Biotechnology Information (NCBI), ORFfinder, softberry and so on. ExPASy, TMPRED, NCBI and SignalP 4.1 Server were used for analysis of genes encoding protein amino-acid sequences. The Ku70 phylogenetic relationships were expressed by constructing a neighbour-joining phylogenetic tree with the software MEGA5.05.
Construction of vectors for ku70 gene replacement
To create the ku70 replacement cassette, 1287-bp 3′- and 1207-bp 5′-fragments flanking the Acku70 sequence were amplified using the primer pairs P12/P13 and P10/P11, respectively. P12 and P13 contained XbaI and SacI restriction sites. P10 and P11 contained HindIII and EcoRI restriction sites. The 3′-fragment digested by XbaI/SacI was ligated to XbaI/SacI-digested pDHt/sknt, containing a G418-resistance cassette [Citation23], to generate the plasmid pDHt/sknt-D. The Acku70 disruption plasmid pDHt/sknt-D-U was generated by inserting the 5′-fragment into the HindII/EcoRI sites of the plasmid pDHt/sknt-D.
Fungal genetic transformation
A. tumefaciens strain LBA4404 (maintained at our laboratory) that was transformed with an appropriate binary vector was cultured in liquid MM at 28 °C for 48 h with 50 µg·mL−1 kanamycin. The A. tumefaciens suspension was diluted to an optical density at 600 nm (OD600) of 0.15 with IM and was cultured at 28 °C until the culture reached an OD600 = 0.4–0.5. The cell suspension (100 μL) was mixed with an equal volume of a conidial suspension (1 × 106 mL−1) from strain GZAAS20.1004 or ΔAcku70. The mixture (200 μL/plate) was plated on a co-cultivation medium (IM) and was incubated at 28 °C for 48 h. MYA medium containing ampicillin (300 μg·mL−1) and hygromicin B (60 µg·mL−1) or G418 (8 µg·mL−1) was plated on the co-culture plate at 28 °C for several days. Transformants were cultured on MYB plates containing hygromycin B (60 µg·mL−1) or G418 (8 µg·mL−1) and incubated at 28 °C.
Isolation of ku70 disruption strain
An Acku70 disruption cassette was constructed using the G418-resistance gene nptII as a selectable marker. The disruption of Acku70 was performed using ATMT. Putative Acku70 disruptants were identified among the G418-resistant transformants using PCR with primer pairs P14/P15 that were selected for nuclear homogeneity using a single spore and further verified by Southern blotting. The probe used for Southern blotting was amplified using the primers nptF (5′-ATCGACTCGAGATGATTGAACAAGATGGATTG-3′) and nptRV (5′-GACGATATCTCAGAAGAACTCGTCAAGAAGGC-3′) that were derived from the plasmid pUR5750, which were labelled with digoxigenin (DIG) using a DIG DNA Kit II (Labkit, Shenzhen, China). The confirmed transformants were designated as ΔAcku70 strains.
Phenotypic analysis
The sensitivity of the mutants to DNA-damaging agents were tested as follows: small conidia (1 × 104 conidia) from each A. chevalieri var. intermedius strain were inoculated onto solid MYB medium containing ethyl methane sulfonate (EMS; 0.25% v/v) (Sigma-Aldrich, Beijing, China) or H2O2 (2 mmol·L−1) and incubated at 28 °C for four days. To determine the sensitivity of each A. chevalieri var. intermedius strain to temperature, small conidia (1 × 104 conidia) were inoculated onto solid MYB medium and incubated at 28 35 °C for four days. For osmotic stress tests, small conidia (1 × 104 conidia) from each A. chevalieri var. intermedius strain were inoculated onto solid MYA medium supplemented with various NaCl concentrations (5%, 10%, 15% m/m) and incubated at 28 °C for four or seven days. The experiments were repeated three times from independently grown cultures.
Analysis of gene replacement efficiency
The putative veA and flbA genes of A. chevalieri var. intermedius were selected for analysis of gene replacements efficiency. Binary vector pDHt/sk-hyg was used for constructing gene replacement cassettes. The vector pDHt/sk-VF containing a 1-kb flanking region of veA was generated as follows: amplification of the 500-bp 5′- and 500-bp 3′-flanking sequences of veA was achieved using the primer pairs P18/P19 and P20/P21, respectively. P18 and P19 contained XhoI and BamHI restriction sites. P20 and P21 contained SpeI and XbaI restriction sites. The two fragments were sequentially inserted into the XhoI/BamHI and SpeI/XbaI sites of plasmid pDHt/sk-hyg to generate the vector pDHt/sk-VF. The vector pDHt/sk-VT contained 1000-bp 5′- and 1000-bp 3′-flanking regions, which were amplified using veA primer pairs P22/P23 and P24/P25, respectively. The vector pDHt/sk-VT was constructed in the same manner as the vector pDHt/sk-VF. The vector pDHt/sk-FA, which was constructed in our laboratory, contains an flbA gene-replacement cassette with 927-bp homology to the upstream region of flbA and 1274-bp homology to the downstream region of flbA.
Results and discussion
Identification and analysis of putative Acku70 genes
A PCR strategy was chosen to determine the sequence of the Acku70 gene. A 1.3-kb sequence with similarity to ku70 cDNA was amplified from A. chevalieri var. intermedius DNA using the degenerate primers P1 and P2 designed according to the highly conserved amino-acid sequences among known fungal Ku70 proteins. Subsequently, 3′ and 5′ rapid amplification of cDNA ends was performed to extend the known sequence in both directions; an extra 3′ flanking DNA sequence was obtained using genome walking PCR. Finally, the nucleotide sequence of a genomic DNA fragment (approximately 3.14 kb) containing a 2234-bp ORF was determined and designated as Acku70 (GI:1007442966).
The Acku70 ORF is interrupted by five introns, which were identified by comparing the DNA sequence with that of the genomic DNA of Acku70. The splice site sequences follow the GT–AG splicing rule. The predicted Ku70 protein of A. chevalieri var. intermedius comprises 645 amino acid residues and shares approximately 74% amino acid sequence identity with A. fumigatus Ku70. The phylogenetic relationships among the fungal Ku70 orthologs are shown in
Isolation of the ΔAcku70 strain
To establish a highly efficient gene replacement system and to determine whether Acku70 is involved in the NHEJ pathway in A. chevalieri var. intermedius, an Acku70 disruption cassette was constructed using the G418-resistance gene nptII as a selectable marker (). The plasmid pDHt/sknt-D-U containing the Acku70 disruption cassette was used for transformation of A. tumefaciens strain LBA4404, and G418R transformants were obtained using the fungal genetic transformation strategy described above. All transformants were preliminarily identified using the primer pair P14/P15 (data not shown); a candidate Acku70-disruption strain designated as Acku70-A29 was isolated and selected for nuclear homogeneity using a single spore. PCR analysis using primer pairs P14/P15 ((A)) and Southern blotting analysis of genomic DNA digested with EcoRI or SacI ((B)), respectively, were used for verification of the identity of the purified Acku70-A29 strain. PCR analysis revealed that a 2611-bp coding sequence of Acku70 was replaced by the G418R cassette. Southern blotting analysis confirmed that a single copy of the G418R cassette integrated into the genome of strain Acku70-A29.
Figure 3. PCR and Southern blotting analysis of the ku70 gene disruption vector. PCR analysis (A) using primer pairs P14 and P15: lane 1, molecular size marker (Sangon Biotech, Shanghai, China); lane 2, wild-type strain; lane 3, ΔAcku70 strain. Southern blotting analysis (B) of the genomic DNA of the ΔAcku70 strain: lane 1, digestion using EcoRI; lane 2, digestion using SacI.
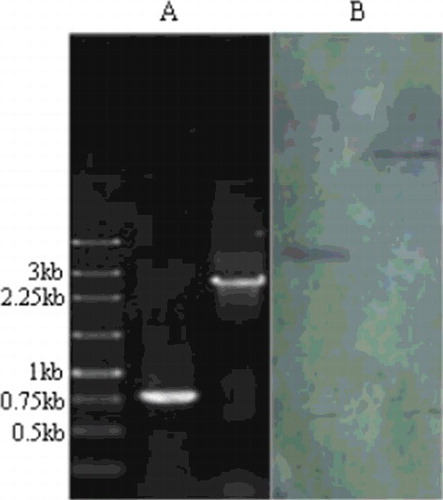
Phenotypic characterization of ΔAcku70
The sexual and asexual modes of reproduction of A. chevalieri var. intermedius are regulated by osmotic pressure and temperature, and the growth rate is influenced by temperature as well [Citation4]. Because Ku70 homologues are involved in NHEJ-mediated repair of DSBs in eukaryotes [Citation9,Citation24,Citation25], we studied the effects of temperature, osmotic stress and DNA-damaging agents on the growth rate. Plate assays demonstrated that the growth rate of ΔAcku70 was slightly lower compared with that of the wild-type strain on solid MYB medium. No morphological irregularities of the mycelia were observed. Compared with the wild type, the mutant identically responded to temperature and osmotic pressure, and there were no differences in sensitivity to various EMS and H2O2 concentrations when the strains were cultured on MYB plates ().
Disrupting ku70 or ku80 decreases the growth of Arthroderma benhamiae and A. fumigatus through an unknown mechanism. The growth of A. chevalieri var. intermedius was also decreased; however, deleting other genes from the ku70 or ku80 disruption strains does not cause an undesirable phenotype [Citation15,Citation26]. Moreover, there were no obvious differences in colony morphology and temperature sensitivity between Acku70 and the wild-type strain. The disruption of Acku70 did not change the sensitivity against genotoxic mutagens, for example, EMS and H2O2. This suggests that a Ku-independent pathway must be involved in the repair of DNA DSBs. Because the sexual and asexual reproduction of A. chevalieri var. intermedius are regulated by osmotic pressure and the growth of the ΔPcku70 strain in P. chrysogenum is completely inhibited at elevated NaCl concentrations [Citation4,Citation19]. we observed the colony growth of ΔAcku70 and wild-type strains in the presence of different NaCl concentrations. The obtained results that ΔAcku70 identically responded to osmotic pressure compared with the wild type indicate that the deletion of ku70 did not influence the osmotic stress-mediated sporogenesis and mycelial growth.
Highly efficient gene replacement using ΔAcku70
The effect of the disruption of Acku70 on homologous gene replacement was evaluated by deleting two putative genes (veA and flbA) from the wild-type and ΔAcku70 strains. The veA gene product plays a critical role in activating sexual development, and the gene inhibits the asexual development of A. nidulans [Citation27]. It is part of the velvet family that is highly conserved in ascomycetes and basidiomycetes. A ΔveA strain of A. nidulans does not produce sexual fruiting bodies (i.e. cleistothecia), but produces conidia both in the light and in the dark [Citation27,Citation28]. FlbA is a key regulatory protein which connects the cascade of FlbB-E proteins and BrlA to the G-protein coupled receptor signalling that is required to arrest vegetative growth during conidiation. FlbA activates the GTPase activity of FadA, thus causing the return of the protein to an inactive state, arresting vegetative growth and allowing asexual sporulation [Citation29,Citation30].
The plasmid pDHt/sk-VF, carrying Hyg flanked by 0.5-kb upstream and 0.5-kb downstream genomic sequences of veA, and the plasmid pDHt/sk-VT, carrying Hyg flanked by 1-kb upstream and 1-kb downstream genomic sequences of veA, were used to replace veA. The hygromycin-resistant transformants were confirmed using PCR with the primer pair P16/P17. The amplicon differed by 715 bp from the recipient strain (2153 bp (0.5 kb/0.5 kb) or 2287 bp (1 kb/1 kb)), indicating that veA was disrupted. The plasmid pDHt/sk-FA, carrying Hyg flanked by 927-bp upstream and 1274-bp downstream genomic sequences of flbA, was used as the target for gene disruption. Transformants were analysed using primer pairs P27/P28. Replacement of flbA was confirmed by a shift from an 897-bp amplicon that was generated from the recipient strain to a 2236-bp amplicon.
As shown in , because of varying lengths of the homologous arms, the frequencies of gene replacement at the veA locus in the two cases were 15.2% and 30.8%, respectively, in the wild-type strain. However, the targeted gene disruption frequency varies among chromosomal loci, and the gene replacement efficiency at the flbA locus was as low as 2.6% in the wild-type strain. These variations may be closely related to locus-specific properties, including differences in chromatin structure. The gene replacement efficiency dramatically increased in ΔAcku70, and the gene disruption frequency increased to 80% at the flbA locus. Although the gene replacement efficiency reached 86.8% at the veA locus, there was ectopic integration of the disruption construct. Therefore, Ku-independent NHEJ pathways, which are present in N. crassa and M. grisea [Citation31,Citation32], may exist in A. chevalieri var. intermedius.
Table 2. Efficiency of gene replacement at the veA and flbA loci in wild-type and ΔAcku70 strains.
Overall, we have successfully improved the gene replacement frequency of A. chevalieri var. intermedius by using the ΔAcku70 strain. The homologous integration frequency of ΔAcku70 increases by blocking the NHEJ pathway. Therefore, the ΔAcku70 strain is expected to speed up the process of study of functional genes in A. chevalieri var. intermedius.
Conclusions
In this study, we first characterized the Ku70 ortholog of A. chevalieri var. intermedius and used gene disruption methods to produce deletion mutants designated as ΔAcku70. ΔAcku70 grew slightly slower than the wild type but showed no obvious differences in colony morphology and temperature sensitivity. The sensitivity of ΔAcku70 to EMS and H2O2 was almost the same as that of the wild type. Further results revealed that the gene replacement efficiencies of flbA, veA (500/500) and veA (1000/1000) were 80%, 83.9% and 86.8% in ΔAcku70, respectively, while they were only 2.6%, 15.2% and 30.8% in the wild-type strain. Thus, we demonstrate here that the Acku70 mutant strain ΔAcku70 is a useful recipient for targeted gene mutagenesis in A. chevalieri var. intermedius.
Acknowledgments
The authors thank the staff of the Guizhou Key Laboratory of Agricultural Biotechnology for contributing to this research.
Disclosure statement
No potential conflict of interest was reported by the authors.
Additional information
Funding
References
- Xu A, Wang Y, Wen J, et al. Fungal community associated with fermentation and storage of Fuzhuan brick-tea. Int J Food Microbiol. 2011;146(1):14–22.
- Deng FM, Gong SL, Yang WL. Study on bioactivity of extracellular polysaccharide of Eurotium criastatum by high throughput screening. Food Machinery. 2007;23(6):48–51.
- Huang Q, Chen LL, Ke YPC. Study on the effect of dark tea fermentation liquid with Eurotium cristatum on the activity of digestive enzyme. Microbiology. 2007;34(5):917–920.
- Liu ZY, Qin J, Li NL. [Study of conditions of sporogenesis of Aspergillus chevalieri var. intermedius in Fuzhuan Tea]. Southwest China J Agricultural Sci. 1991;4(1):73–77. Chinese.
- Ma Q, Liu YX, Liu ZY. Cloning of veA cDNA fragment related to sexual spore development in Eurotium cristatum. Guizhou Agricultural Sci. 2011;39(3):25–27.
- Tan YM, Wang H, Liu YX, et al. Identification of sporulation-related genes in Aspergillus chevalieri var. intermedius by suppression subtractive hybridization. Mycosystema. 2013;32(1):56–63.
- Qiu C, Cheng B, Zhang YS, et al. Efficient knockout of transplanted green fluorescent protein gene in Medaka using TALENs. Mar Biotechnol. 2014;16:674–683.
- Lin SC, Chang YY, Chan CC. Strategies for gene disruption in Drosophila. Cell Biosci. [Internet]. 2014 [cited 2016 Apr 18];4:63. Available from: http://cellandbioscience.biomedcentral.com/articles/10.1186/2045-3701-4-63.
- Kass EM, Jasin M. Collaboration and competition between DNA double-strand break repair pathways. FEBS Lett. 2010;584(17):3703–3708.
- Frank-Vaillant M, Marcand S. Transient stability of DNA ends allows nonhomologous end joining to precede homologous recombination. Mol. Cell. 2002;10:1189–1199.
- Beaton BP, Kwon D-N, Choi Y-J, et al. Inclusion of homologous DNA in nucleasemediated gene targeting facilitates a higher incidence of bi-allelically modified cells. Xenotransplantation. 2015;22:379–390.
- Krappmann S. Gene targeting in filamentous fungi: the benefits of impaired repair. Fungal Biol Rev. 2007;21:25–29.
- Dudasova Z, Dudas A, Chovanec M. Non-homologous end-joining factors of Saccharomyces cerevisiae. FEMS Microbiol Rev. 2004;28:581–601.
- Ninomiya Y, Suzuki K, Ishii C, et al. Highly efficient gene replacements in neurospora strains deficient for nonhomologous end-joining. Proc Natl Acad Sci USA. 2004;101(33):12248–12253.
- da Silva Ferreira ME, Kress MR, Savoldi M, et al. The akuB(KU80) mutant deficient for nonhomologous end joining is a powerful tool for analyzing pathogenicity in Aspergillus fumigatus. Eukaryot Cell. 2006;5(1):207–211.
- Nayak T, Szewczyk E, Oakley CE, et al. A versatile and efficient gene-targeting system for Aspergillus nidulans. Genetics. 2006;172:1557–1566.
- Honda Y, Kobayashi K, Kirimura K. Increases in gene-targeting frequencies due to disruption of kuea as a ku80 homolog in citric acid-producing Aspergillus niger. Biosci Biotechnol Biochem. 2011;75(8):1594–1596.
- Villalba F, Collemare J, Landraud P, et al. Improved gene targeting in Magnaporthe grisea by inactivation of MgKU80 required for non-homologous end joining. Fungal Genet Biol. 2008;45:68–75.
- Hoff B, Kamerewerd J, Sigl C, et al. Homologous recombination in the antibiotic producer Penicillium chrysogenum: strain DeltaPcku70 shows up-regulation of genes from the HOG pathway. Appl Microbiol Biotechnol. 2010;85(4):1081–1094.
- Li ZH, Du CM, Zhong YH, et al. Development of a highly efficient gene targeting system allowing rapid genetic manipulations in Penicillium decumbens. Appl Microbiol Biotechnol. 2010;87:1065–1076.
- Liu ZY, Qing J, Wang NL. The separation and identification of “Golden Flower Fungus” in Fuzhuan tea. J Gac. 1990;9(1):69–74.
- Deng J, Wei M, Yu B, et al. Efficient amplification of genes involved in microbial secondary metabolism by an improved genome walking method. Appl Microbiol Biotechnol. 2010;87(2):757–764.
- Cao Y, Liu YX, Tan YM, et al. Construction of G418 resistance gene expression cassette in binary vector and Agrobacterium tumefaciens mediated transformation of Aspergillus chevalieri var. intermedius. Southwest China J. Agricultural Sci. 2013;26(6):2509–2513.
- Qi XL, Su XF, Guo HM,et al. A ku70 null mutant improves gene targeting frequency in the fungal pathogen Verticillium dahliae. World J Microbiol Biotechnol. 2015;31:1889–1897.
- Zhang TT, Zhang XB, Shi WD, et al. The DNA damage repair protein Ku70 regulates tumor cell and hepatic carcinogenesis by interacting with FOXO4. Pathology Res Practice. 2016;212:153–161.
- Grumbt M, Defaweux V, Mignon B, et al. Targeted gene deletion and in vivo analysis of putative virulence gene function in the pathogenic dermatophyte Arthroderma benhamiae. Eukaryot Cell. 2011;10(6):842–853.
- Kim H, Han K, Kim K, et al. The veA gene activates sexual development in Aspergillus nidulans. Fungal Genet Biol. 2002;37(1):72–80.
- Wang FF, Dijksterhuis J, Wyatt T, et al. VeA of Aspergillus niger increases spore dispersing capacity by impacting conidiophore architecture. Antonie van Leeuwenhoek. 2015;107:187–199.
- van Munster JM, Nitsche BM, Akeroyd M, et al. Systems approaches to predict the functions of glycoside hydrolases during the life cycle of Aspergillus niger using developmental mutants ΔbrlA and ΔflbA. PloS One. [Internet]. 2015 [cited 2016 18 Apr];10(1):e0116269. Available from: http://journals.plos.org/plosone/article?id=10.1371/journal.pone.0116269.
- Han KH, Han KY, Yu JH, et al. The nsdD gene encodes a putative GATA-type transcription factor necessary for sexual development of Aspergillus nidulans. Mol Microbiol. 2001;41(2):299–309.
- Ishibashi K, Suzuki K, Ando Y, et al. Nonhomologous chromosomal integration of foreign DNA is completely dependent on MUS-53 (human Lig4 homolog) in Neurospora. Proc Natl Acad Sci USA. 2006;103(40):14871–14876.
- Kito H, Fujikawa T, Moriwaki A, et al. MgLig4, a homolog of Neurospora crassa Mus-53 (DNA ligase IV), is involved in, but not essential for, non-homologous end-joining events in Magnaporthe grisea. Fungal Genet Biol. 2008;45(12):1543–1551.