ABSTRACT
Bacterial diseases of Cymbidium orchids continue to threaten the floriculture industry. Bacterial isolates were isolated and cultured from Cymbidium plants exhibiting disease symptoms: black lesions with or without water-soaking on leaves. To identify the bacterial isolates, we performed polymerase chain reaction (PCR) amplification using species-specific primers. The pathogen was tentatively identified as Burkholderia gladioli, which was first described as a phytopathogen, and later associated with human infections, especially with patients with cystic fibrosis. PCR-restriction fragment length polymorphism analysis of 16S ribosomal DNA (rDNA) products digested with HinfI and sequencing of the 16S rDNA confirmed the isolate as B. gladioli. Burkholderia gladioli strains infecting plants are divided into two pathovars: alliicola and gladioli. Pathogenicity tests showed that the isolate proved to be pathogenic to Cymbidium orchid but not to onion plants, a common host for B. gladioli pv. alliicola. To the best of our knowledge, this is the first report of B. gladioli in Cymbidium orchids in Korea.
Introduction
Burkholderia spp. is a genus of aerobic, Gram-negative, rod-shaped and motile proteobacteria found in diverse habitats. Some of them are reported to have plant growth-promoting, endophytic and antifungal strains with potential biocontrol activity [Citation1]. However, many of them are mainly known as plant or human pathogens. One of them, Burkholderia gladioli, was initially identified as a pathogen of gladiolus [Citation2]. It was subsequently reported that the bacteria are associated with diseases in other plants and three pathovars were identified according to their specialization to the host plant. B. gladioli pv. alliicola (formerly known as Pseudomonas alliicola) causes onion bulb rot, B. gladioli pv. gladioli (formerly Pseudomonas marginata) causes a leaf and corn disease in gladioli and irises and B. gladioli pv. agaricicola causes soft rot in the mushroom Agaricus bitorquis [Citation3]. A fourth pathovar, B. gladioli pv. cocovenenans (formerly Pseudomonas cocovenenans), is a lethal toxin-producing strain and provides differential characteristics of the food-poisoning pathovar from phytopathogenic pathovars [Citation4].
Orchidaceae (the orchid family) is a diverse and widespread family of flowering plants that often have fragrance and ornamental qualities. Orchidaceae is affected by a range of diseases, including root diseases, stem and pseudobulb decays, leaf spots and flower blights [Citation5]. The most common and important bacterial pathogens found in orchid farms include Pectobacterium carotovora, Dickeya spp., P. cypripedii, Acidovorax cattleya and B. gladioli [Citation5,Citation6]. Particularly, B. gladioli can cause serious losses in orchid production including Dendrobium, Oncidium, Miltonia and Cymbidium. For example, B. gladioli is one of the primary pathogens of orchids in Thailand, and the losses of Dendrobium orchids due to the bacterial disease have been estimated at 50% or more [Citation6,Citation7]. It has also been reported as a major pathogen of Dendrobium orchids in Hawaii as well [Citation5].
Members of the genus Cymbidium belong to the orchid family and are popularly grown as potted flower plants in Korea. Many cultivars of Cymbidium with highly decorative flower spikes developed by breeding systems have been released on the domestic and international market. At present, Cymbidium occupies the largest cultivation area of any orchid in Korea, accounting for 47% (ca. 101 ha) of the total cultivated land for orchid production [Citation8]. So far, the pathogens reported in Korea that cause Cymbidium disease include Fusarium subglutinans, Sclerotium rolfsii, Acidovorax avenae, Cymbidium mosaic virus and Odontoglossum ring spot virus [Citation8–14].
Here, we describe the isolation and identification of the bacterial pathogen B. gladioli from symptomatic Cymbidium leaves based on microbiological characteristics, pathogenicity and the molecular approach. To the best of our knowledge, the finding of B. gladioli on the Cymbidium orchid is the first record in Korea, and we characterized the phenotypic and pathological properties of the isolate.
Materials and methods
Field survey and sampling
Samples were harvested from Cymbidium showing disease symptoms at nine farms located in various regions of South Korea (). Samples were collected during farm visits and were kept at 4 ℃ until analysis.
Isolation and identification of the pathogen
For the isolation of casual agents, marginal sides of symptomatic areas from leaves were cut to approximately 1 cm2 in size and sterilized with 70% ethanol for 1 min. The leaf tissues were placed on tryptic soy agar (TSA) plates, and then were incubated at 28 ℃ for two days. Bacterial colonies were streaked separately onto TSA plates for single colony isolation and were used for this study. The isolated colonies were maintained at 4 ℃ for short-term use or at −78 ℃ in a deep fridge for long-term storage in a glycerol stock. The reference strains B. gladioli pv. gladioli (KCTC 2967) and B. gladioli pv. alliicola (KCTC 12374) [Citation15], used as controls for comparison in the experiments, were obtained from the Korean Collection for Type Cultures (Jeongeup, South Korea).
Molecular characterization of bacteria
Polymerase chain reaction (PCR) amplification was carried out with species-specific primers in a C1000™ thermal cycler (BioRad, Foster City, CA, USA): CMG16-1 (5'-AGAGTTTGATCMTGGCTCAG-3') and G16-2 (5'-CGAAGGATATTAGCCCTC-3') [Citation16–18], GLA-f (5'-CGAGCTAATACCGCGAAA-3') and GLA-r (5'-AGACTCGAGTCAACTGA-3') [Citation19]. PCR reaction was carried out in a 20 μL final volume containing 1 × PCR reaction buffer, 2.5 mmol/L of MgCl2, 50 pmol of each primer, 0.1 mmol/L dNTP (deoxynucleoside triphosphate) mix, 0.5 U Taq DNA polymerase (Geneslabs, Seongnam, South Korea) and approximately 50 ng of bacterial genomic DNA extracted with ExiPrep™ Bacteria Genomic DNA Kit (Bioneer, Daejeon, South Korea). Amplification was performed with an initial denaturation step at 94 ℃ for 2 min; followed by 35 cycles of denaturation at 94 ℃ for 1 min, annealing at 50 ℃ for 1 min and extension at 72 ℃ for 1 min; and a final extension step at 72 ℃ for 7 min (T100™ Thermal Cycler, BioRad, Foster City, CA, USA). The amplified products were separated in 0.8% (w/v) agarose gel in 1 × TBE (Tris-borate-ethylenediaminetetraacetic acid) buffer, stained with RedSafe™ dye (INtRON Biotech., Seongnam, Korea) and visualized under ultraviolet light. PCR products were cloned into the pGEM T-Easy Vector (Promega, Madison, WI, USA) according to the manufacturer's recommendations. Plasmid DNA for sequencing was prepared with the PrimePrep plasmid DNA isolation Kit (GeNetBio, Nonsan, South Korea) according to the recommendations of the manufacturer. Nucleotide sequences from DNA sequencing were aligned using the ClustalW method of MegAlign software (Lagergene, Madison, WI, USA) and homology was determined using the National Center for Biotechnology Information (NCBI) Blast Network Server [Citation20].
Restriction fragment length polymorphism (RFLP) analysis was performed with the restriction endonuclease HinfI (New England Biolab) on nearly full-length 16S ribosomal DNA (rDNA) (approximately 1500 bp) amplified with primers fD1 (5'-CCGAATTCGTCGACAACAGAGTTTGATCCTGGCTCAG-3') and rD1 (5'-CCCGGGATCCAAGCTTAAGGAGGTGATCCAGCC-3') [Citation21–23]. B. gladioli pv. gladioli (Bgg, KCTC 2967) and B. gladioli pv. alliicola (Bga, KCTC 12374) were used as reference controls. Nucleotide sequences of PCR products obtained from isolates 9 and 14 from Cymbidium farm No. 2, Bgg and Bga were aligned using the ClustalW method of MegAlign software (Lagergene) and homology was determined using the NCBI Blast Network Server (https://blast.ncbi.nlm.nih.gov/Blast.cgi) [Citation20].
Koch's postulates
Bacteria grown on TSA plates for two days were used for the inoculation tests. To fulfil Koch's postulates, leaves from six-month-old Cymbidium were inoculated by wounding with a sterile toothpick smeared with a reference control Bga and the isolate 2-9 obtained from Cymbidium orchids with disease symptoms. Sterile water was used for negative control inoculation. The inoculated Cymbidium plants were maintained in a growth chamber at 24 °C and 70% relative humidity for two weeks. Disease development was observed beginning at two to three days after inoculation. Bacteria were then reisolated from the Cymbidium leaves displaying symptoms and were grown on TSA plates at 24 ℃ for two days.
Pathogenicity tests
The ability of the isolate 2-9 to cause rot on a possible alternative host, onion, was determined. Overnight cultures of bacterial strains were scraped off TSA plates, suspended in sterile water at about 5.0 × 108 cfu/mL, and 10 µL of the bacterial suspension was used as inoculum. Onion blubs and slices of onion approximately 1.25 cm thick were placed in Petri dishes containing moistened 3M filter paper (Whatman, Maidstone, UK). Onion bulbs and scales were stabbed with a sterilized needle, and a 5 µL droplet of the bacterial suspension was placed on the wounded site. Sterile water was used as a control inoculation. The inoculated bulbs and scales were kept in a growth chamber at 28 ℃ for 10 days. A necrotic zone or soft rot lesion at least 2 mm beyond the inoculation site was considered as evidence of infection for all pathogenicity tests. Bacteria were reisolated from marginal sides of the infected areas to confirm the identity of the pathogen. For onion leaf inoculation, at least five leaves of each onion plant were inoculated by the scissors-clipping method [Citation24–26]. All inoculation experiments were conducted at least three times.
Results and discussion
Symptoms and isolation of the bacterium
Bacteria responsible for disease symptoms were recovered from Cymbidium farms located at various regions of South Korea (). The isolates were identified by PCR amplification using species-specific primers. Previously, one of the species-specific primer set, CMG16-1 and G16-2, was developed to detect the presence of the specific gene regions in the area coding 16S rDNA of B. gladioli [Citation16–18,Citation27]. We used the primer set and successfully amplified an approximately 470-bp fragment of 16S rDNA from B. gladioli pv. alliicola (Bga) and B. gladioli pv. gladioli (Bgg) ((A)). The amplicon was not observed from Erwinia cypripedii, Dickeya chrisanthemi, Agrobacterium tumefaciens or Escherichia coli, indicating that the primer set, CMG16-1 and G16-2, is species-specific. Two bacterial isolates (strain 2-9 and 2-14) from 18 of the diseased Cymbidium tissues from greenhouse No. 2 in the Kyounggi-do region gave amplification typical for B. gladioli ((B)). Bacterial isolates from other farms did not produce specific amplification for B. gladioli (data not shown). Bga was used as a positive control (P). In PCR with another set of species-specific primers for B. gladioli, GLA-f and GLS-r [Citation19], an approximately 300 bp fragment was amplified in strain 2-9 as well as in the positive controls Bga and Bgg ((C)). These results indicate that Cymbidium displaying disease symptoms on leaves was infected by B. gladioli.
Figure 2. Detection of B. gladioli from Cymbidium samples harvested from Cymbidium farm No.2. (A) Amplification using species-specific oligonucleotide primers, CMG16-1 and G16-2, to detect B. gladioli. L: size marker (Geneslabs, Seongnam, South Korea).
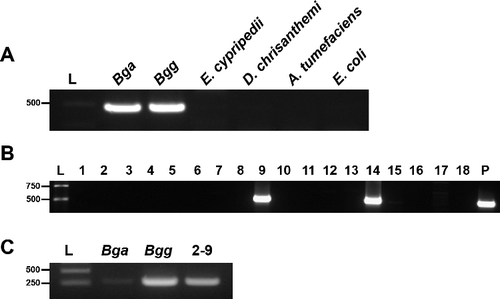
The recognized symptoms from infected leaves included dark-brown to black lesions often surrounded by water-soaking ((A)). Bacterial isolates from this type of diseased Cymbidium leaf tissue revealed the presence of a dominant form of bacterial colonies on TSA. The isolated colonies produced round-shaped, beige-yellowish colonies, approximately 2–2.5 mm in diameter for two-day cultivation and with the majority of the colonies secreting a diffusible yellow or tan pigment into the media ((B)).
Identification of Burkholderia gladioli
To examine the species-specific amplicons obtained by PCR using primer CMG16-1 and G16-2, we sequenced the 470-bp PCR products of isolate 2-9, 2-14, Bga and Bgg. The sequence from isolate 2-9 was identical (100%, without gaps) with the one from 2-14 (data not shown). Their nucleotide sequences were compared with gene sequences of known strains in the GenBank sequence database (https://blast.ncbi.nlm.nih.gov/Blast.cgi). A BLAST search supported the species-specific PCR results that the isolates were B. gladioli (data not shown) [Citation28,Citation29]. A sequence alignment of the gene products was constructed using MegAlign software (ClustalW method) (). The alignment displayed only three mismatched nucleotides between Bga and Bgg. The nucleotide sequence from the isolate 2-9 displayed 100% and 99.4% identity with Bga and Bgg, respectively, demonstrating that the bacterial pathogen from diseased Cymbidium tissues is B. gladioli.
Figure 4. Sequence alignment of 470-bp fragment of the 16S rDNA of the isolate 2-9, B. gladioli pv. galioli (Bga), B. gladioli pv. galioli (Bgg).
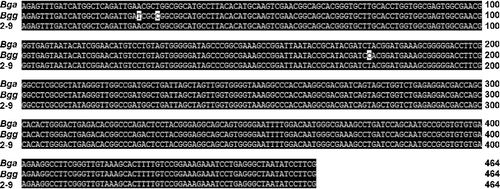
Based on the sequence analysis of PCR products (), the bacterial isolates obtained from diseased Cymbidium samples could be ascribed to B. gladioli. Additionally, we carried out PCR-RFLP analysis of the isolates 2-9 and 2-14 with reference controls, Bgg and Bga. Nearly full-length 16S rDNA was amplified with primers fD1 and rD1. The RFLP patterns of bacterial isolates 2-9 and 2-14 obtained using HinfI restriction endonuclease were the same as that of Bgg but not the Bga reference strain used in this study (). Both strands of the PCR products were sequenced using primers fD1 and rD1 and then aligned using MegAlign software (Clustal W method) (Figure S1 in the Online Supplementary Appendix). Bacterial isolate 2-9 showed very high levels of similarity with both reference controls, Bgg and Bga (99.7%–99.8%).
Reisolation of Burkholderia gladioli from inoculated Cymbidium orchids
Six-month-old Cymbidium plants were artificially inoculated with isolate 2-9 and B. gladioli, and lesion development was monitored and compared for 12 days after inoculation (). Negative control with a toothpick displayed a small wounding site without further development for 12 days. However, inoculation with isolate 2-9 and B. gladioli resulted in development of lesions around the inoculation sites with black necrosis. The water-soaked lesions appeared three to eight days after inoculation, rapidly enlarged and turned to dark-brown to black lesions within 8 to 12 days after inoculation. Eventually, the symptoms became similar to those observed in the greenhouse as shown in (A). Reisolations from the artificially inoculated Cymbidium plants produced round-shaped beige-yellowish colonies of approximately 2–2.5 mm in diameter for two-day cultivation similar to the colonies in (B) (data not shown). Identification of the bacterium was confirmed by PCR using B. gladioli-specific primers, CMG16-1 and G16-2, followed by sequencing analysis, thus fulfilling Koch's postulates (Figure S2 in the Online Supplementary Appendix).
Pathogenicity tests
Plant strains of B. gladioli are divided into pathovars, alliicoli and gladioli, according to their specialization to the host plant [Citation30,Citation31]. According to the early work by Ballard et al. [Citation32], pv. alliicola and pv. gladioli are indistinguishable using physiological testing. Studies on the distinction between the pathovars of B. gladioli are nearly non-existent [Citation17,Citation18]. In the present study, the isolate 2-9 was identified as B. gladioli by PCR analysis using species-specific primers and the sequence analysis of the 16S rDNA gene. Bga and Bgg are very closely related and indistinguishable phylogenetically and phenotypically [Citation30–32]. It was difficult to determine the pathovar of the isolate 2-9 by molecular analysis including PCR-RFLP and 16S rDNA sequencing because of high similarity between pathovar alliicola and pathovar gladioli. Therefore, we decided to perform a pathogenicity test using a possible alternative host.
Bgg has very wide host ranges including gladioli, freesia, irises and Dendrobium [Citation1]. On the contrary, Bga, which infects tulip and onion [Citation1], is well known to cause ‘slippery skin’ on onion bulbs and dry necrotic spots on onion leaves [Citation15,Citation17,Citation18]. The first report in Korea of soft rot of onion by Bga was in the summer of 1999 [Citation15], and it is now becoming a common disease during storage or transit. Previously, 25 isolates of B. gladioli were characterized and 10 of the isolates were identified as B. gladioli pv. gladioli in Italy, since none of them was pathogenic on onion plants [Citation21]. To obtain more precise identification of the 2-9 isolate, we carried out a pathogenicity test using onions. For the pathogenicity assay, onion bulbs were inoculated with isolate 2-9, Bgg and Bga. After inoculation and incubation at 28 ℃ for 10 days, photo images were obtained from the cross section and the scale of inoculated onion bulbs. The tissue around the point inoculated with Bga became bright yellow and water-soaked 10 days after inoculation (). On the contrary, onion bulbs inoculated with Bgg or isolate 2-9 displayed dried wound in the absence of typical symptoms of B. gladioli, water-soaked and macerated internal rot. Detached onion bulb scales inoculated with isolate 2-9, Bgg or Bga also displayed similar results (data not shown). When 20-fold concentrated bacterial inoculums were applied, only onion bulbs inoculated with B. gladioli pv. alliicola were macerated at 10 days after inoculation (Figure S3 in the Online Supplementary Appendix).
Figure 7. Symptoms of cross-inoculation studies of isolate 2-9 on a possible alternative host, onion, 10 days after inoculation. Onion bulbs (A) and scales (B).
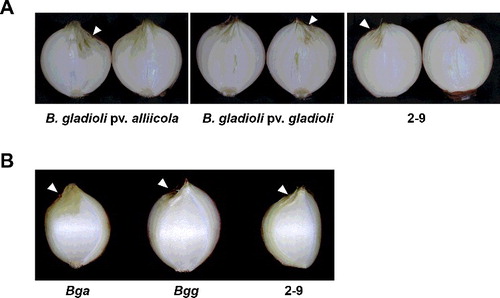
To quantify the pathogenicity of B. gladioli and isolate 2-9 on onion plants, the leaf tips of onion plants were inoculated with Bga, Bgg and isolate 2-9 using the scissors-clipping method [Citation24,Citation25]. The disease progress of Bga was rapid from the tip of the leaf, resulting in the wilting and eventual drying of the infected leaves ((A)). At two days after inoculation, significant increases in the lesion lengths were detected in onions inoculated with Bga compared with those infected by Bgg and the isolate 2-9 ((B)). At 14 days after inoculation, Bga displayed significantly larger lesion lengths of around 2.5 cm compared to Bgg and the isolate 2-9 displaying 0.5 cm lesion lengths similar with the negative control.
Figure 8. Symptom development on onion leaves inoculated with B. gladioli pv. alliicoli (Bga), B. gladioli pv. gladioli (Bgg) and isolate 2-9. Onion leaves (A) and lesion length measurements (B) of bacteria-inoculated plants over 14 days.
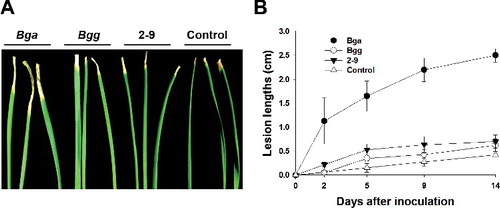
Taken together, our results indicate that the isolate 2-9 is pathogenic to Cymbidium but not to onion, suggesting that the isolate 2-9 could be Bgg. However, although onion plants are common host plants for Bga, there are a few reports that Bgg is isolated from diseased onions [Citation17–19]. Therefore, although isolate 2-9 is most likely Bgg, the interpretation of the results from the pathogenicity test should be viewed with caution. Because bacterial rot disease on Cymbidium caused by B. gladioli may have an extensive economic impact and quarantine on the Cymbidium industry, additional nation-wide monitoring should be carried out in Korea. In addition, copper and streptomycin resistance is widespread among strains of B. gladioli in nurseries [Citation5]. It has been suspected that frequent use of chemicals containing copper and streptomycin may have inadvertently resulted in the development of the resistant B. gladioli [Citation5]. Therefore, in addition to a monitoring system of B. gladioli, adequate attention to control the bacterial disease is required to minimize economic losses in the Cymbidium industry.
Conclusions
In this study, bacterial isolates were isolated from Cymbidium leaves exhibiting dark-brown to black lesions. The isolated colonies produced round-shaped, beige-yellowish colonies for two-day cultivation and secreted a diffusible yellow or tan pigment into the media. Sequence analysis of nearly full-length 16S rDNA displayed very high levels of identity with two pathovars of B. gladioli, Bgg and Bga. To achieve pathovars determination of the isolate, we performed a pathogenicity test using a possible alternative host, onion. Bulbs and leaves of onion plants inoculated with the isolate and a reference strain Bgg displayed dried wound in the absence of typical symptoms of Bga, water-soaked and macerated internal rot. Our results suggest that dark-brown to black lesions of Cymbidium plants are caused by Bgg. This is, to the best of our knowledge, the first description of occurrence of bacterial rot of Cymbidium plants caused by B. gladioli in Korea.
Supplementary_Data.pdf
Download PDF (1.1 MB)Acknowledgments
The authors are grateful to Min Jang for the critical reading of manuscript.
Disclosure statement
No potential conflict of interest was reported by the authors.
Additional information
Funding
References
- Compant S, Nowak J, Coenye T, et al. Diversity and occurrence of Burkholderia spp. in the natural environment. FEMS Microbiol Rev. 2008;32:607–626.
- McCulloch L. A bacterial disease of gladiolus. Science. 1921;54:115–116.
- Yabuuchi E, Kosako Y, Oyaizu H, et al. Proposal of Burkholderia gen. nov. and transfer of seven species of the genus Pseudomonas homology group II to the new genus, with the type species Burkholderia cepacia (Palleroni and Holmes 1981) comb. nov. Microbiol Immunol. 1992;36:1251–1275.
- Jiao Z, Kawamura Y, Mishima N, et al. Need to differentiate lethal toxin-producing strains of Burkholderia gladioli, which cause severe food poisoning: description of B. gladioli pathovar cocovenenans and an emended description of B. gladioli. Microbiol Immunol. 2003;47:915–925.
- Keith LM, Sewake KT, Zee FT. Isolation and characterization of Burkholderia gladioli from orchids in Hawaii. Plant Dis. 2005;89:1273–1278.
- Chan YL, Lin KH, Sanjayaet al., Gene stacking in Phalaenopsis orchid enhances dual tolerance to pathogen attack. Transgenic Res. 2005;14:279–288.
- Chuenchitt S, Dhirabhava W, Karnjanarat S, et al. A new bacterial disease on orchids Dendrobium sp. caused by Pseudomonas gladioli. Kasetsart J. 1983;17:26–36.
- Han KS, Park JH, Back CG, et al. First report of Fusarium subglutinans causing leaf spot disease on Cymbidium orchids in Korea. Mycobiology. 2015;43:343–346.
- Han KS, Lee SC, Lee JS, et al. First report of sclerotium rot on Cymbidium orchids caused by Sclerotium rolfsii in Korea. Mycobiology. 2012;40:263–264.
- Kim SM, Choi SH. Simultaneous detection of Cymbidium mosaic virus and Odontoglossum ringspot virus in orchids using multiplex RT-PCR. Virus Genes. 2015;51:417–422.
- Lee BD, Kim WG, Cho WD, et al. Occurrence of dry rot on Cymbidium orchids caused by Fusarium spp. in Korea. Plant Pathol J. 2002;18:156–160.
- Yoon JY, Chung BN, Choi GS, et al. Genetic variability in the coat protein genes of Cymbidium mosaic virus isolates from orchids. Virus Genes. 2012;44:323–328.
- Ajjikuttira PA, Lim-Ho CL, Woon MH, et al. Genetic variability in the coat protein genes of two orchid viruses: Cymbidium mosaic virus and Odontoglossum ringspot virus. Arch Virol. 2002;147:1943–1954.
- Ryu KH, Park WM. Rapid detection and identification of odontoglossum ringspot virus by polymerase chain reaction amplification. FEMS Microbiol Lett. 1995;133:265–269.
- Lee CJ, Lee JT, Kwon JH, et al. Occurrence of bacterial soft rot of onion plants caused by Burkholderia gladioli pv. alliicola in Korea. Australasian Plant Pathol. 2005;34:287–292.
- Bauernfeind A, Schneider I, Jungwirth R, et al. Discrimination of Burkholderia gladioli from other Burkholderia species detectable in cystic fibrosis patients by PCR. J Clin Microbiol. 1998;36:2748–2751.
- Stoyanova M, Hristova P, Petrov N. Method for differentiating Burkholderia gladioli pathovars. Science Technol. 2011;6:15–19.
- Stoyanova M, Kizheva Y, Chipeva V, et al. Phytopathogenic Burkholderia species in bulb plants in Bulgaria. Biotechnol Biotechnol Equip. 2011;25:2477–2483.
- Furuya N, Ura H, Iiyama K, et al. Specific oligonucleotide primers based on sequences of the 16S-23S rDNA spacer region for the detection of Burkholderia gladioli by PCR. J General Plant Pathol. 2002;68:220–224.
- Altschul SF, Gish W, Miller W, et al. Basic local alignment search tool. J Mol Biol. 1990;215:403–410.
- Fiori M, Ligios V, Schiaffino A. Identification and characterization of Burkholderia isolates obtained from bacterial rot of saffron (Crocus sativus L.) grown in Italy. Phytopathol Mediterr. 2011;50:450–461.
- Segonds C, Heulin T, Marty N, et al. Differentiation of Burkholderia species by PCR-restriction fragment length polymorphism analysis of the 16S rRNA gene and application to cystic fibrosis isolates. J Clin Microbiol. 1999;37:2201–2208.
- Weisburg WG, Barns SM, Pelletier DA, et al. 16S ribosomal DNA amplification for phylogenetic study. J Bacteriol. 1991;173:697–703.
- Chern M, Canlas PE, Fitzgerald HA, et al. Rice NRR, a negative regulator of disease resistance, interacts with Arabidopsis NPR1 and rice NH1. Plant J. 2005;43:623–635.
- Chern M, Fitzgerald HA, Canlas PE, et al. Overexpression of a rice NPR1 homolog leads to constitutive activation of defense response and hypersensitivity to light. Mol Plant Microbe Interact. 2005;18:511–520.
- Kauffman HE, Reddy APK, Hsieh SPV, et al. An improved technique for evaluation of resistance of rice varieties to Xanthomonas oryzae. Plant Dis Rep. 1973;57:537–541.
- Mujahid TY, Siddiqui K, Ahmed R, et al. Isolation and partial characterization of phosphate solubilizing bacteria isolated from soil and marine samples. Pak J Pharm Sci. 2014;27:1483–1490.
- Seo YS, Lim JY, Park J, et al. Comparative genome analysis of rice-pathogenic Burkholderia provides insight into capacity to adapt to different environments and hosts. BMC Genomics [Internet]. 2015 [cited 2016 Jun 16];16:349. Available from: http://bmcgenomics.biomedcentral.com/articles/10.1186/s12864-015-1558-5
- Seo YS, Lim J, Choi BS, et al. Complete genome sequence of Burkholderia gladioli BSR3. J Bacteriol. 2011;193:3149.
- Saddler GS. Burkholderia gladioli pv. alliicola. Mycipathologia. 1994;128:55–56.
- Saddler GS. Burkholderia gladioli pv. gladioli. Mycipathologia. 1994;128:57–58.
- Ballard RW, Palleroni NJ, Doudoroff M, et al. Taxonomy of the aerobic pseudomonads: Pseudomonas cepacia, P. marginata, P. alliicola and P. caryophylli. J Gen Microbiol. 1970;60:199–214.