ABSTRACT
The domain of unknown function DUF946 family consists of plant proteins with an average length of around 239 residues that have no characterized function. The gene family needs deeper characterization and functional analysis of its members. In this study, we report five OsDUF946 (OsDUF946.1–OsDUF946.5) family members in rice Nipponbare with three distinct motifs. All the OsDUF946 proteins were classified into three major groups (I, II, III) by phylogenetic analysis. Real-time polymerase chain reaction showed that the expression patterns of the five OsDUF946 family members were different in 15 different rice tissues as well as under various stress conditions and abscisic acid treatment. The expression of OsDUF946.1 was significantly downregulated under salt, cold and heat stress, while the expression of OsDUF946.4 and OsDUF946.5 was significantly upregulated under drought and salt conditions. Overexpression of OsDUF946.4 in Escherichia coli significantly improved the resistance to salt and drought, while overexpression of OsDUF946.5 in E. coli did not have such an effect. The obtained results provide a starting point for further research into the function of the OsDUF946 family in rice.
Introduction
The domain of unknown function (DUF) families in the Pfam database (http://pfam.xfam.org/family) includes many highly conserved DUFs presumably with important biological function, as well as DUFs whose functions have not been proved to be essential and have not been unravelled yet [Citation1–3]. Some DUF-containing proteins have recently been reported to enhance the abiotic stress tolerance in plants [Citation4–8].
Abiotic stresses, e.g. drought, salinity and extreme temperatures, are the primary cause of crop loss worldwide, reducing the average yields for most major crop plants by more than 50% [Citation9]. In order to preclude the detrimental impacts from specific stress factors and ensure survival, plants have evolved intricate mechanisms to perceive external signals, allowing optimal response to environmental conditions [Citation10–15]. Drought and salinity generally account for osmotic stress, leading to the disruption of homeostasis and ion distribution in cells [Citation16–18]. It is well known that a range of environmental stress factors can activate similar signalling pathways and cellular responses, including the synthesis of stress proteins, upregulation of antioxidants and accumulation of compatible solutes [Citation11,Citation19–22]. Rice (Oryza sativa), as a dietary staple for almost two-thirds of the world's population, is one of the most important agricultural crops. Recently, some genes have been used successfully to improve the tolerance of rice to stresses [Citation23–28].
The DUF946 family consists of a number of plant proteins of unknown function with an average length of around 239 residues. The gene family needs deeper characterization and functional analysis of its members. In this study, we analysed the OsDUF946 family in rice and studied the expression profile of five OsDUF946 members and their expression pattern under various stresses and abscisic acid (ABA) treatment. To better understand the biological role of this family of proteins, two of the studied genes, OsDUF946.4 and OsDUF946.5, were overexpressed in transgenic Escherichia coli.
Materials and methods
Database searches
The sequences of putative OsDUF946 family proteins in rice were obtained by searching the rice genome annotation project (RGAP version 7) database (http://rice.plantbiology.msu.edu/) with domain number PF06101. All the corresponding sequences of the putative OsDUF946 family members were then downloaded from RGAP and confirmed with the SMART database (http://smart.embl-heidelberg.de/smart/batch.pl) [Citation29,Citation30]. If there were several gene models at one locus, only the complete gene model was selected for further sequence analysis.
Sequence analysis
Information about the chromosomal localization, amino acid (aa) sequence length and full-length cDNA accessions was obtained for each OsDUF946 gene from RGAP and the Knowledge-Based Oryza Molecular Biological Encyclopedia (KOME) (http://cdna01.dna.affrc.go.jp/cDNA). PSORT (http://psort.hgc.jp/form.html) was used to predict the protein localization of OsDUF946 members. MEME version 4.11.2 (http://meme.nbcr.net/meme/) was used to predict motifs [Citation31]. The alignment of the aa sequences of OsDUF946 family members was done using Clustal Omega [Citation32].
Plant growth and various stress and ABA treatment
Rice (Oryza sativa L. subsp. japonica cv. Nipponbare) seeds were germinated for three days, and then seedlings were grown hydroponically in a 30-L vessel containing nutrient solution [Citation33]. At the emergence of the fourth leaf, the seedlings were subjected to various stresses and ABA treatment. For high-salinity treatment, NaCl solution was added to achieve a final concentration of about 200 mmol/L. Drought stress was modelled by putting intact plants in the air without water supply. For cold stress, the vigorous seedlings were transferred to a growth chamber at 4 °C (12-h-light/12-h-dark cycle). For heat stress, the seedlings were subjected to 42 °C heat shock treatments. For ABA treatment, 100 μmol/L ABA was sprayed onto the leaves of seedlings.
RNA extraction and real-time polymerase chain reaction
Samples were collected and ground to fine powder in liquid nitrogen. Total RNA was extracted with Trizol reagent (GIBCO, Burlington, ON, USA) according to the manufacturer's instructions. Then the total RNA was treated with RNase-free DNase I (Invitrogen, Carlsbad, CA, USA) for 15 min to degrade any potential DNA contamination. Real-time polymerase chain reaction (PCR) was performed on an optical 96-well plate with a Bio-Rad CFX96 (Cambridge, MA, USA) real-time PCR system. Each reaction contained 5 µL of 2 × SYBR Green Master Mix Reagent (Roche, Indianapolis, IN, USA), 1 µL of cDNA samples and 200 nmol/L gene-specific primer in a final volume of 10 µL. The thermal cycle used was as follows: 95 °C for 10 min, followed by 39 cycles of 95 °C for 10 s, 60 °C for 30 s and 72 °C for 1 min. The rice OsUbiquitin gene (LOC102716385) was used as an internal control with primers 5′-AACCAGCTGAGGCCCAAGA-3′ and 5′-ACGATTGATTTAACCAGTCCATGA-3′. The primers used in the expression analysis of the OsDUF946 gene family members are listed in Table S1 in the Supplementary Appendix. The 2–△△Ct method was used for analysis of the relative expression data as described previously [Citation34].
Assay for salt stress and drought stress tolerance of E. coli transformants
In order to construct the expression vector pET32a-OsDUF946.4 and pET32a-OsDUF946.5, specific primers were designed. For OsDUF946.4, sense, 5′-GGATCCATGCGAAGGAGCAAGTGGTG-3′ (BamHI site underlined) and antisense, 5′-AAGCTTTTACTTGGCCTCCCTCTCGT-3′ (HindIII site underlined). For OsDUF946.5, sense, 5′-GGATCCATGCAAAATGATACAGATAC-3′ (BamHI site underlined) and antisense, 5′-AAGCTTTCAGGACCTCTCCAATGCTT-3′ (HindIII site underlined). The amplified products were cloned into a pET32a vector at the BamHI-HindIII site to express the fusion protein, which has a Trx•Tag™ thioredoxin at the N-terminus [Citation35]. The transformed E. coli Rosetta cells (Sanborn, MN, USA) were grown in Luria–Bertani (LB) liquid medium containing 100 µg/mL of ampicillin at 37 °C overnight, then they were inoculated into fresh LB medium (1:100 dilution) supplemented with ampicillin (100 µg/mL) to be incubated until the exponential growth phase (optical density at 600 nm (OD600) of 0.5–0.6). Isopropylthio-β-D-galactoside was added into the cultures to induce the expression of the transformed gene.
For the salt and drought (mimic) tolerance assay, the growth curve of E. coli transformants was plotted. The OD values of pET-32a, pET32a-OsDUF946.4 and pET32a-OsDUF946.5 cells were measured at 0, 2, 4, 6, 8, 10, 12, 14, 16 and 18 h under normal conditions and 200 mmol/L NaCl/300 mmol/L mannitol treatment.
Data analysis
The experiments were repeated three times. Data are mean values from three independent experiments, with standard error of the means. Statistical analysis was performed using the Student's t-test by Microsoft EXCEL version 2003.
Results and discussion
Identification of OsDUF946 family members in rice
Five OsDUF946 gene family members were identified () by searching the rice genome annotation project database with domain number PF06101. The presence of these OsDUF946 genes in the rice genome was then confirmed with the SMART database (Figure S1 in the Supplementary Appendix). These genes were designated as OsDUF946.1 to OsDUF946.5 according to their positions on pseudomolecules (). The five OsDUF946 members were identified to be located on chromosome 2 (OsDUF946.1), 3 (OsDUF946.2 and OsDUF946.3), 7 (OsDUF946.4) and 10 (OsDUF946.5). The rice genome includes 10 duplicated blocks accounting for 45% of its total size [Citation36]. Therefore, it could be speculated that genome duplication might be the mechanism that underlies the distribution of OsDUF946 family members in different chromosomes, similar to that previously suggested for OsDUF866 members [Citation37].
Table 1. OsDUF946 genes and some properties of the putative proteins they encode.
Sequence analysis of OsDUF946 family proteins
The length of OsDUF946 proteins was predicted to vary from 551 aa (OsDUF946.4) to 3101 aa (OsDUF946.1). The OsDUF946.1 sequence includes two DUF946 domains, a C2 domain (protein kinase C conserved region 2), an SHR-BD domain and a VPS13-C domain (Figure S1 in the Supplementary Appendix); thus it was very long. The putative OsDUF946.1 and OsDUF946.5 proteins were predicted to be located in the plasma membrane and the nucleus, respectively, while OsDUF946.2, OsDUF946.3 and OsDUF946.4 were all predicted to be located in the microbody. Information about the amino acid sequence length, the full-length cDNA accessions, molecular weight (MW) and isoelectric point (pI) is given in .
The multiple sequence alignment analysis of the putative OsDUF946 members performed using Clustal Omega (Figure S2 in the Supplementary Appendix) showed that OsDUF946.2 and OsDUF946.3 had the highest sequence similarity among the five OsDUF946 members (54.7%). The MEME motif search tool identified three distinct motifs in the five OsDUF946 sequences (). Motif 1, motif 2 and motif 3 were found in OsDUF946.2, OsDUF946.3 and OsDUF946.4, respectively. Interestingly, the predicted OsDUF946.1 sequence includes four motif 2 and two motif 3 sequences but no motif 1, whereas the OsDUF946.5 sequence includes one motif 1 and two motif 2 sequences but no motif 3.
Figure 1. Conservative structural analysis of rice OsDUF946 family. (A) Motif 1, motif 2 and motif 3 were conserved motifs in rice OsDUF946 family obtained by MEME. (B) Distribution of conserved motifs in OsDUF946 proteins identified by MEME software.
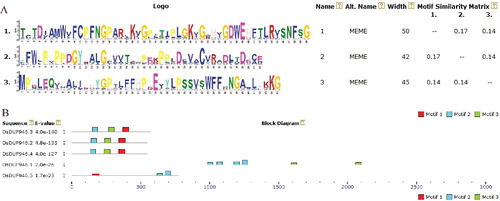
To examine the evolutionary relationships among the OsDUF946 family members in rice and Arabidopsis, a phylogenetic analysis was performed using MEGA4 [Citation38]. Here four Arabidopsis DUF946 members, AT1G04090.1, AT3G04350.1, AT5G18490.1, AT5G43950.1, were included as reference sequences. The results indicated that the putative OsDUF946 proteins could be classified into three major groups (I, II, III) (). Group I contained seven members (AT3G04350.1, AT5G18490.1, AT1G04090.1, AT5G43950.1, DUF946.2, DUF946.3 and DUF946.4), whereas group II and group III both contained only one member, DUF946.1 and DUF946.5, respectively.
Figure 2. Phylogenetic relationship of five OsDUF946 members in rice and four DUF946 members in Arabidopsis.
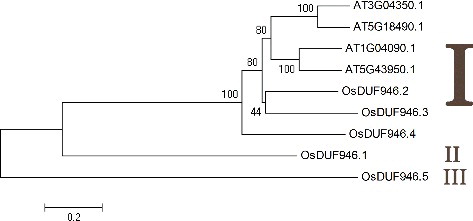
Interestingly, the putative proteins belonging to group I were characterized by only one intron, as opposed to the proteins belonging to group II and group III that had more introns (). Furthermore, we observed that the proteins with close phylogenetic relationships tended to have the same predicted subcellular localization. For example, DUF946.2, DUF946.3 and DUF946.4 were all predicted to be located in the microbody.
Expression patterns of OsDUF946 family members in various tissues
So far little is known about the expression patterns of OsDUF946 family genes in various tissues of rice at different stages. Therefore, we examined the expression of the OsDUF946 family members in 15 different tissues by real-time PCR. The results indicated that spatio-temporal expression patterns of the studied OsDUF946 genes were completely different (). For OsDUF946.1, the highest expression was in the pistil from 10–14 cm inflorescence, whereas the lowest expression was in the endosperm at 28 days after flowering. For OsDUF946.2, the highest expression was in the leaf blade from plants with four tillers, whereas the lowest expression was also in the endosperm at 28 days after flowering. For OsDUF946.3, the expression in the stem at the ripening stage was the highest, and the expression in the embryo at 28 days after flowering, the endosperm at 7 and 28 days after flowering was very low. For OsDUF946.4, the highest expression was in the root at the four-leaf stage, whereas the lowest expression was in the leaf sheath from plants with four tillers. For OsDUF946.5, the highest expression was observed in the embryo at 28 days after flowering, whereas the lowest expression was in the leaf sheath from plants with four tillers. These results suggested that the studied OsDUF946 family members could play different roles in all tissues at the stage of growth, development and maturation in rice.
Figure 3. Real-time PCR analysis of OsDUF946 genes in different tissues of Nipponbare rice. Fifteen representative tissues are as follows: Lb1, leaf blade at four-leaf stage; Lb2, leaf blade from plants with four tillers; Lb3, leaf blade at ripening stage; Ls1, leaf sheath at four-leaf stage; Ls2, leaf sheath from plants with four tillers; Rt1, root at four-leaf stages; Rt2, root from plants with four tillers; St1, stem from plants with four tillers; St2, stem at ripening stage; An, 1.2–1.5 mm anther; Pi, pistil from 10–14 cm inflorescence; Em1, embryo at 7 days after flowering; Em2, embryo at 28 days after flowering; En1, endosperm at 7 days after flowering; En2, endosperm at 28 days after flowering.
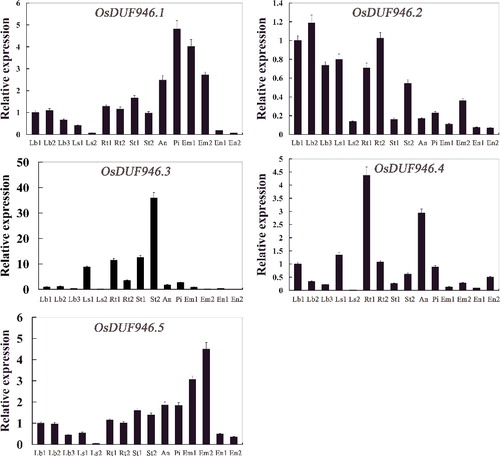
Expression analysis of OsDUF946 genes under various stress conditions
The expression of OsDUF946 genes in conditions of abiotic stress and ABA treatment was examined Nipponbare rice seedlings at the emergence of the fourth leaf (). The expression of OsDUF946.1 was significantly (P < 0.05) downregulated under salt, cold and heat conditions. The expression of OsDUF946.2 was significantly (P < 0.05) upregulated under salt conditions, yet downregulated under drought conditions. OsDUF946.3 showed significantly (P < 0.05) upregulated expression under heat stress conditions, yet downregulated under drought, salt and cold conditions. For OsDUF946.4, the expression was significantly (P < 0.05) upregulated under drought, salinity, cold conditions and ABA treatment, but downregulated under heat conditions. The expression of OsDUF946.5 was significantly (P < 0.05) upregulated under drought and salinity conditions, yet remained unchanged under cold, heat stress conditions and ABA treatment.
Figure 4. Relative expression levels of OsDUF946 genes in Nipponbare rice seedlings at the emergence of the fourth leaf under various stress conditions and ABA treatment detected by real-time PCR. D, drought; S, salt; C; cold; H, heat; A, ABA.
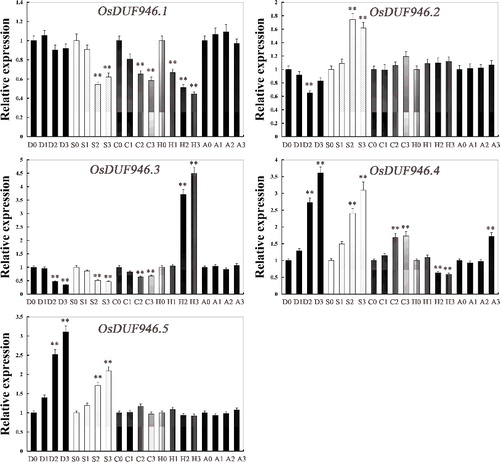
Some gene family members usually display a common significant increase or decrease under various stress conditions [Citation39–41]. In our study, five members of the OsDUF946 family in rice showed very different expression patterns in RNA level under various stress conditions and ABA treatment, suggesting that OsDUF946 genes play different roles in response to various stresses.
OsDUF946.4 improved the tolerance to salt and drought in transgenic E. coli
The expression of OsDUF946.4 and OsDUF946.5 increased under drought and salinity conditions, indicating that they might be involved in drought and salt tolerance. To test this hypothesis, we overexpressed these two genes in E. coli and then determined the drought and salinity resistance of E. coli recombinants based on their growth curves. The OD values of pET-32a, pET32a-OsDUF946.4 and pET32a-OsDUF946.5 were measured at 0–18 h under normal conditions and 200 mmol/L NaCl/300 mmol/L mannitol treatments (). Under normal conditions, the growth rate in E. coli transformed with pET32a-OsDUF946.4 and pET32a-Os DUF946.5 was the same as that of E. coli transformed with pET-32a. Under drought and salt conditions, the growth rate in E. coli transformed with pET32a-OsDUF946.4 was much higher than that of the control (Rosetta/pET-32a) from 2 to 18 h of exposure ((A)), whereas there was no evident difference in the growth rate between E. coli transformed with pET32a-OsDUF946.5 and the control ((B)). This indicated that overexpression of OsDUF946.4 in E. coli significantly improved the resistance to salt and drought, while overexpression of OsDUF946.5 in E. coli did not have such an effect.
Figure 5. Growth of E. coli recombinants overexpressing OsDUF946.4 (A) and OsDUF946.5 (B) under salt and drought stresses.
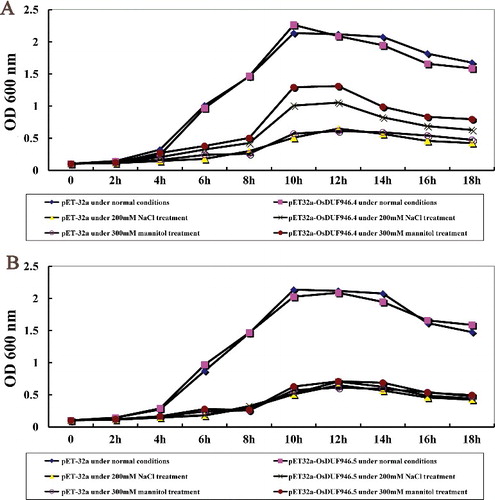
A number of stress-related proteins from plants are reported to improve the stress tolerance in bacteria. For example, the LEM3 protein from soybean and OsHsp90 protein from rice enhanced the high-salinity resistance and thermotolerance, respectively, of E. coli [Citation42,Citation43]. LEA proteins are mainly low-molecular-weight (10–30 kDa) proteins involved in protecting higher plants from damage caused by environmental stresses, especially drought or dehydration [Citation42–45]. HKT transporters mediate Na+ unloading from the xylem under salinity stress, which prevents Na+ overaccumulation in leaves, thereby protecting photosynthetic organs [Citation46–49]. It is, therefore, tempting to speculate that the overexpression of OsDUF946.4 might have resulted in upregulated expression of LEA protein-like and HKT-transporter-like genes in E. coli, which could have led to the enhanced tolerance to drought and salinity. Since overexpression of OsDUF946.5 did not improve the resistance to salinity and drought in E. coli, it could be hypothesized that, in E. coli transformed with pET32a-OsDUF946.5, the overexpression of OsDUF946.5 could not activate the downstream cascades leading to expression of drought and salt stress-related genes. .
Conclusions
This study provided important information regarding the OsDUF946 genes and showed the expression patterns of OsDUF946 genes in different tissues, and under various abnormal conditions, including abiotic stress and ABA treatment. Furthermore, this study suggested that OsDUF946.4 could improve the resistance to drought and salinity in transgenic E. coli. These data can provide important reference for further studies of the functions of OsDUF946 family and could contribute to the genetic improvement of rice.
Supplementary_Data.pdf
Download PDF (523.9 KB)Disclosure statement
No potential conflict of interest was reported by the authors.
Additional information
Funding
References
- Bateman A, Coggill P, Finn RD. DUFs: families in search of function. Acta Crystallogr Sect F. 2010;66:1148–1152.
- Goodacre NF, Gerloff DL, Uetz P. Protein domains of unknown function are essential in bacteria. MBio. 2014 [cited 2016 Jul 13];5:e00744–00713. Available from: http://mbio.asm.org/content/5/1/e00744-13.full
- Hauser R, Pech M, Kijek J, et al. RsfA (YbeB) proteins are conserved ribosomal silencing factors. PLoS Genet. 2012[cited 2016 Jul 13];8:e1002815. Available from: http://journals.plos.org/plosgenetics/article?id=10.1371/journal.pgen.1002815
- He X, Hou X, Shen Y, et al. TaSRG, a wheat transcription factor, significantly affects salt tolerance in transgenic rice and Arabidopsis. FEBS Lett. 2011;585:1231–1237.
- Kim SJ, Ryu MY, Kim WT. Suppression of Arabidopsis RING-DUF1117 E3 ubiquitin ligases, AtRDUF1 and AtRDUF2, reduces tolerance to ABA-mediated drought stress. Biochem Biophys Res Commun. 2012;420:141–147.
- Luo C, Guo C, Wang W, et al. Overexpression of a new stress-repressive gene OsDSR2 encoding a protein with a DUF966 domain increases salt and simulated drought stress sensitivities and reduces ABA sensitivity in rice. Plant Cell Rep. 2014;33:323–336.
- Wang L, Shen R, Chen LT, et al. Characterization of a novel DUF1618 gene family in rice. J Integr Plant Biol. 2014;56:151–158.
- Guo C, Luo C, Guo L, et al. OsSIDP366, a DUF1644 gene, positively regulates responses to drought and salt stresses in rice. J Integr Plant Biol. 2016;58:492–502.
- Boyer JS. Plant productivity and environment. Science. 1982;218:443–448.
- Prabha R, Ghosh I, Singh DP. Plant stress gene database: a collection of plant genes responding to stress condition. ARPN J Sci Technol. 2011;1(1);28–31.
- Wang W, Vinocur B, Altman A. Plant responses to drought, salinity and extreme temperatures: towards genetic engineering for stress tolerance. Planta. 2003;218:1–14.
- Herms DA, Mattson WJ. The dilemma of plants: to grow or defend. Q Rev Biol. 1992;67:283–335.
- Soltis DE, Soltis PS. The role of phylogenetics in comparative genetics. Plant Physiol. 2003;132:1790–1800.
- Xiang Y, Tang N, Du H, et al. Characterization of OsbZIP23 as a key player of the basic leucine zipper transcription factor family for conferring abscisic acid sensitivity and salinity and drought tolerance in rice. Plant Physiol. 2008;148:1938–1952.
- Bohnert HJ, Gong Q, Li P, et al. Unraveling abiotic stress tolerance mechanisms – getting genomics going. Curr Opin Plant Biol. 2006;9:180–188.
- Serrano R, Rodriguez-Navarro A. Ion homeostasis during salt stress in plants. Curr Opin Cell Biol. 2001;13:399–404.
- Zhu JK. Regulation of ion homeostasis under salt stress. Curr Opin Plant Biol. 2003;6:441–445.
- Zhu JK. Plant salt tolerance. Trends Plant Sci. 2001;6:66–71.
- Cushman JC, Bohnert HJ. Genomic approaches to plant stress tolerance. Curr Opin Plant Biol. 2000;3:117–124.
- Knight H, Knight MR. Abiotic stress signalling pathways: specificity and cross-talk. Trends Plant Sci. 2001;6:262–267.
- Shinozaki K, Yamaguchi-Shinozaki K. Molecular responses to dehydration and low temperature: differences and cross-talk between two stress signaling pathways. Curr Opin Plant Biol. 2000;3:217–223.
- Vierling E, Kimpel JA. Plant responses to environmental stress. Curr Opin Biotechnol. 1992;3:164–170.
- Sato H, Todaka D, Kudo M, et al. The Arabidopsis transcriptional regulator DPB3-1 enhances heat stress tolerance without growth retardation in rice. Plant Biotechnol J. 2016;14(8):1756–1767.
- Liu J, Zhang C, Wei C, et al. The RING finger ubiquitin E3 ligase OsHTAS enhances heat tolerance by promoting H2O2-induced stomatal closure in rice. Plant Physiol. 2016;170:429–443.
- Hong Y, Zhang H, Huang L, et al. Overexpression of a stress-responsive NAC transcription factor gene ONAC022 improves drought and salt tolerance in rice. Front Plant Sci. 2016;7:4. Available from: http://journal.frontiersin.org/article/10.3389/fpls.2016.00004/full
- Yoon DH, Lee SS, Park HJ, et al. Overexpression of OsCYP19-4 increases tolerance to cold stress and enhances grain yield in rice (Oryza sativa). J Exp Bot. 2016;67:69–82.
- Min HJ, Jung YJ, Kang BG, et al. CaPUB1, a hot pepper U-box E3 ubiquitin ligase, confers enhanced cold stress tolerance and decreased drought stress tolerance in transgenic rice (Oryza sativa L.). Mol Cells. 2016;39:250–257.
- Alam MM, Tanaka T, Nakamura H, et al. Overexpression of a rice heme activator protein gene (OsHAP2E) confers resistance to pathogens, salinity and drought, and increases photosynthesis and tiller number. Plant Biotechnol J. 2015;13:85–96.
- Schultz J, Milpetz F, Bork P, et al. SMART, a simple modular architecture research tool: identification of signaling domains. Proc Natl Acad Sci U S A. 1998;95:5857–5864.
- Letunic I, Doerks T, Bork P. SMART: recent updates, new developments and status in 2015. Nucleic Acids Res. 2015;43(Database issue):D257–D260.
- Bailey TL, Boden M, Buske FA, et al. MEME SUITE: tools for motif discovery and searching. Nucleic Acids Res. 2009;37:202–208.
- Sievers F, Wilm A, Dineen D, et al. Fast, scalable generation of high-quality protein multiple sequence alignments using Clustal Omega. Mol Syst Biol. 2011;7(7):1429–1432.
- Yoshida S, Forno DA, Cook JH, et al. Laboratory manual for physiological studies of rice. Manila: International Rice Research Institute; 1976. p. 61–67.
- Livak KJ, Schmittgen TD. Analysis of relative gene expression data using real-time quantitative PCR and the 2(-ΔΔC(T)) method. Methods. 2001;25:402–408.
- LaVallie ER, DiBlasio EA, Kovacic S, et al. A thioredoxin gene fusion expression system that circumvents inclusion body formation in the E. coli cytoplasm. Biotechnology (N Y). 1993;11:187–193.
- Wang X, Shi X, Hao B, et al. Duplication and DNA segmental loss in the rice genome: implications for diploidization. New Phytol. 2005;165:937–946.
- Li L, Xie C, Ye T, et al. Molecular characterization, expression pattern, and function analysis of the rice OsDUF866 family. Biotechnol Biotechnol Equip. 2016 Dec 22 [cited 2017 Jan 24]. doi: 10.1080/13102818.2016.1268932
- Tamura K, Dudley J, Nei M, et al. MEGA4: Molecular Evolutionary Genetics Analysis (MEGA) software version 4.0. Mol Biol Evol. 2007;24:1596–1599.
- Ito Y, Katsura K, Maruyama K, et al. Functional analysis of rice DREB1/CBF-type transcription factors involved in cold-responsive gene expression in transgenic rice. Plant Cell Physiol. 2006;47:141–153.
- Dubouzet JG, Sakuma Y, Ito Y, et al. OsDREB genes in rice, Oryza sativa L., encode transcription activators that function in drought-, high-salt- and cold-responsive gene expression. Plant J. 2003;33:751–763.
- Su CF, Wang YC, Hsieh TH, et al. A novel MYBS3-dependent pathway confers cold tolerance in rice. Plant Physiol. 2010;153:145–158.
- Liu D, Lu Z, Mao Z, et al. Enhanced thermotolerance of E. coli by expressed OsHsp90 from rice (Oryza sativa L.). Curr Microbiol. 2009;58:129–133.
- Liu Y, Zheng Y, Zhang Y, et al. Soybean PM2 protein (LEA3) confers the tolerance of Escherichia coli and stabilization of enzyme activity under diverse stresses. Curr Microbiol. 2010;60:373–378.
- Browne J, Tunnacliffe A, Burnell A. Anhydrobiosis: plant desiccation gene found in a nematode. Nature. 2002;416:38–38.
- Hong-Bo S, Zong-Suo L, Ming-An S. LEA proteins in higher plants: structure, function, gene expression and regulation. Colloids Surf B Biointerfaces. 2005;45:131–135.
- Rus A, Lee BH, Munoz-Mayor A, et al. AtHKT1 facilitates Na+ homeostasis and K+ nutrition in planta. Plant Physiol. 2004;136:2500–2511.
- Kader MA, Seidel T, Golldack D, et al. Expressions of OsHKT1, OsHKT2, and OsVHA are differentially regulated under NaCl stress in salt-sensitive and salt-tolerant rice (Oryza sativa L.) cultivars. J Exp Bot. 2006;57:4257–4268.
- Platten JD, Egdane JA, Ismail AM. Salinity tolerance, Na+ exclusion and allele mining of HKT1;5 in Oryza sativa and O. glaberrima: many sources, many genes, one mechanism? BMC Plant Biol. 2013 [cited 2016 Jul 13];13:32. Available from: https://bmcplantbiol.biomedcentral.com/articles/10.1186/1471-2229-13-32
- Schroeder JI, Delhaize E, Frommer WB, et al. Using membrane transporters to improve crops for sustainable food production. Nature. 2013;497:60–66.