ABSTRACT
Fifteen actinomycetes were isolated from deep-sea sediments in Calyptogena communities in the Japan Trench (depth: 5352 m) and Sagami Bay (depth: 1171 m) under incubation at 4–37 °C. A relatively large number of colonies were obtained with incubation at 30 °C from the deep-sea sediment of Sagami Bay. We found Rhodococcus, Nocardioides and Micromonospora actinomycetes in both sediments, and additionally isolated an Aeromicrobium actinomycete from the Japan Trench and Streptomyces and Actinomadura actinomycetes from Sagami Bay. Actinomadura sp. DS-MS-114 isolated from the deep-sea sediment in Sagami Bay produced an antibacterial compound against Staphylococcus aureus NBRC12732T. Structural analysis by liquid chromatography–high-resolution electrospray ionization mass spectrometry (LC-HRESIMS) and proton nuclear magnetic resonance (1H-NMR) indicated that the antibacterial compound was 5,6-dihydro-1,8-dihydroxy-3-methylbenz[a]anthracene-7,12-quinone, which is known as an immunosuppressant. The deep-sediment in the Calyptogena community may be useful as a source of various actinomycetes producing physiologically active substances.
Introduction
The exploration of deep-sea environments has emerged as a new frontier in drug discovery [Citation1]. Actinomycetes are one of the best sources of bioactive natural compounds, and a wide variety of antimicrobial substances have been obtained and applied for drug development [Citation2,Citation3]. Although deep-sea sediments represent an environment which is distinct from that associated with terrestrial samples, several actinomycetes belonging to the genera Micromonospora, Rhodococcus and Streptomyces from a deep-sea sediment in the north-western Pacific Ocean (depth: 300–6499 m) and the genus Dermacoccus from Mariana Trench sediment (depth: 10 898 m) were isolated [Citation4,Citation5].
Calyptogena biological communities have been found at deep-sea cold-seep areas in subduction zones of continental margins, mud volcanoes and deep-sea trenches [Citation6–8]. These biological communities consist of mussels, clams, tubeworms and micro-organisms including mainly abundant sulphur-reducing bacteria, methanotrophic archaea and heterotrophic micro-organisms [Citation9–11]. We have already isolated Aneurinibacillus sp. YR247 belonging to the heterotrophic Firmicutes from the Calyptogena community in Sagami Bay at a depth of 1171 m and showed that the bacterial isolate exhibits various antimicrobial activities [Citation12]. Although we demonstrated that the Calyptogena community is useful as a bacterial isolation source, taxonomic classification of deep-sea actinomycetes in the community is uncertain and little is known about the actinomycetes producing antimicrobial compounds in the Calyptogena community.
In this study, using deep-sea sediments of the Calyptogena communities of Japan trench (depth: 5352 m) and Sagami Bay (depth: 1171 m) as the isolation sources, we obtained actinomycetes; taxonomically classified these actinomycetes and investigated an actinomycete that produced an antibacterial compound. The inhibitory activities of the compound against fungi, yeasts and bacteria were evaluated. Additionally, the structure of the antibacterial compound was determined.
Materials and methods
Screening of actinomycetes in calyptogena communities
Deep-sea sediments were collected inside Calyptogena communities in the Japan Trench (39°6.390′ N 143°53.502′ E) at a depth of 5352 m in September 2009 and in Sagami Bay (35°0.072′ N 139°13.477′ E) at a depth of 1171 m in August 2010 using a Hyper-Dolphin remotely operated vehicle [Citation12]. These deep-sea sediments were collected and immediately stored at −80 ˚C. To obtain actinomycetes, the sediment (ca. 50 µL volume) was spread on glycerol ammonium-salt plate consisting of (w/v) 1% glycerol, 0.1% NH4Cl, 0.1% K2HPO4, 0.05% NaCl, 0.05% MgSO4·7H2O, 0.1% CaCO3, 50 µg/mL cycloheximide, 50 µg/mL nystatin, 20 µg/mL nalidixic acid and 2% agar (pH 7.7), in August 2010. After incubation at 4, 10, 30 or 37 °C for 30 d, several colonies had grown on these plates at these temperatures. The colonies were repeatedly purified and separated, suspended in 15% glycerol and stored at −80 °C. The bacterial isolates were aerobically cultured in 10 mL of Luria–Bertani (LB) modified medium containing 0.1% Bacto tryptone (Difco Laboratories, Detroit, MI, USA), 0.5% Bacto yeast extract (Difco), 0.5% glucose, 1.0% NaCl, 0.2% MgSO4·7H2O, 0.02% K2HPO4, 50 µg/mL cycloheximide, 50 µg/mL nystatin and 20 µg/mL nalidixic acid (pH 6.9) at each temperature for 3–10 d. The bacterial cell pellet obtained at each temperature was stored at −20 °C.
Taxonomic classification of bacterial isolates from calyptogena communities
A total of 18 bacterial isolates that exhibited fine growth in LB-modified medium were evaluated. DNA fraction was extracted from each bacterial cell pellet using an Illustra bacteria genomic Prep Mini Spin Kit (GE Healthcare, Munich, Germany). The genomic DNA of each strain was used as a template. To determine the taxonomic classification, the 16S rRNA gene fragment was amplified from the DNA fraction by polymerase chain reaction (PCR) with eubacterial primers 27f: 5′-GAGTTTGATCCTGGCTCAG-3′ and 1525r: 5′-AAAGGAGGTGATCCAGCC-3′ [Citation13]. The PCR conditions were previously reported [Citation12]. The 5’-terminal region (500 bp) of each 16S rRNA gene fragment was sequenced by FASMAC DNA Sequencing Services (Kanagawa, Japan) and analyzed with NCBI (National Center for Biotechnology Information) BlastN program (https://blast.ncbi.nlm.nih.gov/Blast.cgi) [Citation14].
Phylogenetic analysis of bacterial isolate
Strain DS-MS-114, which exhibited high antibacterial activity against Staphylococcus aureus NBRC12732T (see below), was phylogenetically analyzed. The 16S rRNA gene fragment of strain DS-MS-114 was amplified and sequenced as described above. Its 16S rRNA sequence consists of 1408 nucleotides. Nucleotide substitution rates (Knuc) were determined, and a distance matrix tree was constructed by using the neighbour-joining (NJ) method with the CLUSTAL_X program [Citation15–17]. Alignment gaps and unidentified base positions were not taken into consideration for the calculations. The topology of the phylogenetic tree was evaluated by performing a bootstrap analysis with 1000 replications [Citation18]. NJ plot software (http://pbil.univ-lyon1.fr/software/njplot.html) was used to prepare a graphical view of the phylogenetic tree.
Production and purification of the antibacterial compound
Strain DS-MS-114 was aerobically cultured in 2.7 L of yeast-starch medium containing 1% soluble starch (Difco), 0.05% yeast extract (Difco) and 1% artificial seawater (pH 7.0) at 37 °C for 7 d. After cultivation, the culture supernatant (2.6 L) was obtained by centrifugation at 6000g at 4 °C for 30 min. The culture supernatant was adjusted to pH 8.0 with NaOH, extracted with 3.3 L of ethyl acetate and placed on a rotary evaporator. The solvent was removed at 37 °C and 80 hPa for 2 h and 180 mg of the residue obtained was resuspended in chloroform to prepare the 50 mg/mL crude extract. Then, the crude extract in chloroform was subjected to silica gel chromatography (C200, 1.2 × 20 cm, Wako Pure Chemical, Osaka, Japan). Elution was performed with chloroform. Using agar-spot assay with S. aureus NBRC12732T, the active fraction was identified and collected. To confirm the antibacterial compound, 10 µL of the fraction at 10 mg/mL was developed using hexane:ethyl acetate (8:2, v/v) on a thin-layer chromatography (TLC) silica gel plate (TLC aluminium sheets, 8 × 8 cm, SilicaGel 60F254, Merck Co., Kenilworth, NJ, USA) and phosphomolybdic acid staining was performed. The residue (6.7 mg) in the active fractions was collected by rotary evaporation at 37 °C and 80 hPa for 2 h, resuspended in methanol and used for the evaluation of antimicrobial activity by the agar-spot assay.
For structural analysis, the residue (6.7 mg) in the methanol fraction was subjected to preparative thin-layer chromatography. The residue was developed using hexane:ethyl acetate (8:2, v/v) on a TLC silica gel plate, followed by detection of the antibacterial compound by phosphomolybdic acid staining. The active spot area of another developed TLC plate was collected and the residue was extracted with an aliquot of methanol. The antibacterial activity of the methanol fraction against S. aureus NBRC12732T was detected by the agar-spot assay. The methanol fraction containing the residue (1.0 mg) was used for structural analysis.
Detection of antibacterial activity
The antibacterial activity of bacterial isolates against S. aureus NBRC12732T and the antimicrobial activity of the methanol fraction of strain DS-MS-114 against Aspergillus brasiliensis NBRC9455, Candida albicans NBRC1594, S. aureus NBRC12732T, and Escherichia coli NBRC3301T were evaluated by the agar-spot assay. For a total of 18 bacterial isolates, each strain was aerobically cultured in 5.0 mL of yeast-starch medium at 4–37 ˚C for 7 d. After cultivation, the culture supernatant was obtained by centrifugation at 6000g at 4 °C for 30 min. For S. aureus NBRC12732T, modified LB plates containing 0.1% Bacto tryptone (Difco), 0.5% Bacto yeast extract (Difco), 0.5% glucose, 1.0% NaCl, 0.2% MgSO4·7H2O, 0.02% K2HPO4, 2% agar (pH 7.0) and 1.0 × 107 cfu/mL of S. aureus NBRC12732Tcells were prepared. Then, the bacterial culture supernatant (50 µL) was spotted onto the surface of modified LB plates pre-seeded with S. aureus NBRC12732T. After incubation for 2 d at 37 °C, the inhibition zone was checked. After fractionation by silica gel chromatography, for the methanol fraction of strain DS-MS-114, modified LB plates containing 1.0 × 107 cfu/mL of C. albicans NBRC1594 cells, S. aureus NBRC12732T cells or E. coli NBRC3301T cells, modified LB plates containing 1.0 × 104 spores/mL A. brasiliensis NBRC9455 spores were prepared. Then, 100 µg of the methanol fraction (with 50 µL at 2 mg/mL) was spotted onto the surface of modified LB plates containing each micro-organism. After incubation for 1–3 d at 28–37 °C, the inhibition zone was checked. All experiments for evaluation of the antimicrobial activity were carried out in triplicate.
Structural analysis of the antimicrobial compound from strain DS-MS-114
The ultraviolet (UV) spectrum was obtained on a U2800 spectrophotometer (Hitachi High-Technologies Corp., Tokyo, Japan). The antimicrobial compound was analyzed by reversed-phase liquid chromatography-high resolution electrospray ionization mass spectrometry (LC-HRESIMS, LTQ Orbitrap XL mass spectrometer; Thermo Fisher Scientific, Waltham, MA, USA) in positive ion mode. The sample solution (5 µL) was eluted with a linear gradient between (A) CH3CN containing 0.1% formic acid and (B) 0.1% aqueous formic acid (5% A from 0 to 3 min; from 5% to 100% A, from 3 to 10 min; 100% A from 10 to 20 min) at a flow rate of 0.3 mL/min using an ODS column (CAPCELL PAK C18 IF 2 µm, 2.0 × 100 mm, Shiseido, Tokyo, Japan). The proton nuclear magnetic resonance (1H-NMR) spectrum was measured with a JEOL JNM-ECA600 spectrometer at 25 °C using tetramethylsilane as an internal reference.
Results and discussion
Actinomycetes from deep-sea sediments in Calyptogena communities
The numbers of bacterial colonies isolated on glycerol ammonium-salt plates at 4–37 °C for 30 d are indicated in . These colonies in most cases were obtained with 2.0–6.0 × 10 cfu/mL wet sediment. A slightly larger number of colonies with 3.8 × 102 cfu/mL wet sediment was obtained from the deep-sea sediment of Sagami Bay with incubation at 30 °C. It was reported that the highest numbers of bacterial colonies were obtained at shallow sites of the Japan Sea (depth: 289 m), Sagami Bay (depth: 1168 m) and Suruga Bay (depth: 1151–1487 m) with 106–107 cfu/mL wet sediment among all deep-sea areas near the Japanese archipelago (depth: 289–10 898 m) [Citation4]. Similarly, the location of the Calyptogena community in Sagami Bay (depth: 1171 m) is shallower than that in the Japan Trench (depth: 5352 m), and higher counts of bacterial colonies were obtained from the relatively shallow Calyptogena community in Sagami Bay ().
Table 1. Distribution of cultivable bacteria and abundance of actinomycetes in deep-sea sediments.
Using a total of 18 colonies with good growth in LB modified medium, 1.5 kbp of the 16S rRNA gene fragments were amplified. The 5'-regions of the amplified DNA fragments were sequenced at the length of 500 bp, and the bacterial isolates were classified [Citation14]. The results in indicated that the 15 actinomycetes from the deep-sea Calyptogena communities belonged to the following genera: Rhodococcus, Aeromicrobium, Nocardioides, Micromonospora, Rhodococcus/Nocardia, Streptomyces and Actinomadura, with sequence similarities of 99–100%. Although many actinomycetes were isolated from surface marine environment [Citation19], Streptomyces, Rhodococcus, Actinomadura and Micromonospora actinomycetes had already been found in the deep-sea sediments of the Japan Trench and/or Sagami Bay [Citation4,Citation20]. Nocardioides actinomycete was isolated from deep-sea sediment (depth: 3800 m) [Citation20]. At the time of writing, we isolated Aeromicrobium actinomycetes from the deep-sea Calyptogena community in the Japan Trench (depth 5352 m) for the first time. The identification of deep-sea actinomycetes and characterization of secondary metabolites would provide ways to exploit the utility of these actinomycetes as novel sources of useful substances for pharmaceutical biotechnology.
Detection of antibacterial activity and phylogenetic analysis of strain MS-DS-114
S. aureus is known as a cause of several common illnesses [Citation21]. Additionally, S. aureus resistant to methicillin or vancomycin have been isolated in recent years [Citation22,Citation23], and the development of pharmaceuticals against those bacteria has attracted much attention. To identify an effective micro-organism possessing antibacterial activity against S. aureus NBRC12732T, the antibacterial activities were evaluated using 18 of the bacterial culture supernatants (50 µL) by agar-spot assay. As a result, the culture supernatant of strain DS-MS-114 isolated from Sagami Bay (depth: 1171 m) cultured at 37 °C exhibited a distinct antibacterial activity against S. aureus NBRC12732T.
Its 16S rRNA gene consisted of 1408 nucleotides. The results from the phylogenetic analysis based on 16S rRNA gene sequence data are shown in ; strain DS-MS-114 clearly falls within the genus Actinomadura and belongs to actinomycetes. The nucleotide sequence of the 16S rRNA gene of strain DS-MS-114 was most closely related to those of Actinomadura miaoliensis BC44T-5T (EF116925) and Actinomadura keratinilytica WCC-2265T (EU637009) with similarities of 99% to both. The next closest level of similarity was 97% similarity to Actinomadura fibrosa ATCC49459T (AF163114), Actinomadura rudentiformis HMC1T (NR_043687), Actinomadura hibisca IMSNU22185T (NR_042031), Actinomadura kijaniata DSM43764T (NR_026344), Actinomadura namibiensis DSM44197T (AJ420134) and Actinomadura oligospora ATCC43269T (NR_025021). The 16S rRNA sequence of strain MS-DS-114 was submitted to the NCBI GenBank database (Accession No. LC194234).
Purification of the antibacterial compound and evaluation of antimicrobial activity
The antibacterial compound was purified from 2.6 L of the culture supernatant of strain MS-DS-114 using silica gel chromatography, and approximately 6.7 mg of residue in the active fraction was obtained. The Rf value of the antibacterial compound (100 µg at 10 mg/mL) was 0.52 using hexane:ethyl acetate (8:2, v/v) on TLC silica gel plates ((A)). As shown in (B), 100 µg of the active fraction (50 µL at 2 mg/mL) exhibited antimicrobial activity against the Gram-positive bacterium S. aureus NBRC12732T (inhibition zone 12.7 mm) but not against the fungus A. brasiliensis NBRC9455, yeast C. albicans NBRC1594 or the Gram-negative bacterium E. coli NBRC3301T. The inhibitory zone remained the same for several days (data not shown). Antimicrobial activity of the solvent against the test strains was not detected by the agar spot assay. Related Actinomadura actinomycetes produce several antimicrobial compounds against Clostridium perfringens S107, Clostridium difficile 630, Trypanosoma brucei brucei strain GUTat 3.1, S. aureus, Kocuria rhizophila or Proteus hauseri [Citation24–29]. Compared with related studies, we demonstrated that strain DS-MS-114 produces an antibacterial compound against S. aureus NBRC12732T.
Structural analysis of the antibacterial compound
For structural analysis, the residue (6.7 mg) in the active fraction was subjected to preparative thin-layer chromatography, and 1.0 mg of the purified antibacterial compound was obtained with a dark red plate (λmax 226, 269 and 450 in MeOH). As shown in (A) and (B), the purified antibacterial compound was analyzed with reversed-phase LC-HRESIMS in positive ion mode. The antibacterial compound was eluted at 10.18 min, and the protonated molecule was detected at m/z 307.0966 ([M+H]+). The molecular weight of the antibacterial compound was estimated to be 306.0966, and its molecular formula C19H14O4 was determined on the basis of the HRESIMS result. The 1H-NMR analysis showed the following: 1H-NMR (CDCl3), δ12.10 (1H, s), 9.14 (1H, s), 7.76 (1H, dd 1.0, 7.5 Hz), 7.63 (1H, dd 7.5, 8.3 Hz), 7.30 (1H, dd 1.0, 8.3 Hz), 6.78 (1H, brs), 6.66 (1H, brs), 2.80 and 2.71 (2H, m, 2H, m) and 2.31 s (3H, s). These results for the antibacterial compound correspond with those of 5,6-dihydro-1,8-dihydroxy-3-methylbenz[a]anthracene-7,12-quinone () [Citation26]. Actinomadura actinomycetes produce antibiotics belonging to the polyether family [Citation30], anthracyclin family [Citation31], naphtoquinone family [Citation32] and macrolactam family [Citation33]. Additionally, Actinomadura sp. AC104 produce an antibacterial compound against S. aureus and the compound contains a benzenic ring disubstituted by aliphatic chains [Citation25]. Compared with related studies, our results indicated that the antibacterial compound produced by strain DS-MS-114 differs from the known antibiotics produced by other Actinomadura species.
Figure 3. LC-HRESIMS analysis of the purified antibacterial compound obtained by preparative thin-layer chromatography. Ultraviolet detection (A) of the purified antibacterial compound at a retention time of 10.18 min, with the protonated molecule at m/z 307.0966 by HRESIMS in positive ion mode (B).
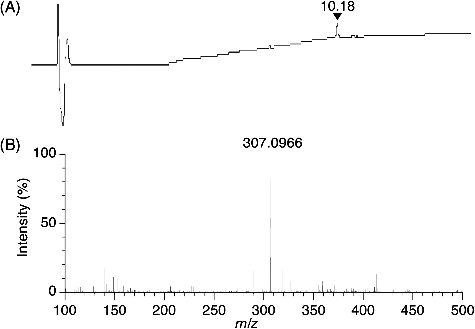
The antibacterial compound, namely, 5,6-dihydro-1,8-dihydroxy-3-methylbenz[a]anthracene-7,12-quinone, was previously reported as an intermediate for synthesis of tetrangulol [Citation26]. Tetrangulol is an antibiotic produced by Streptomyces rimosus and has a benz[a]anthracene-7,12-quinone skeleton. Tetrangulol and 5,6-dihydro-1,8-dihydroxy-3-methylbenz[a]anthracene-7,12-quinone belong to the angucyclines, which is a group of polyketide natural products including antitumour and/or antibiotic compounds [Citation27]. Therefore, the antitumour activity of 5,6-dihydro-1,8-dihydroxy-3-methylbenz[a]anthracene-7,12-quinone is expected to be examined. Additionally, the immunosuppressant activity of 5,6-dihydro-1,8-dihydroxy-3-methylbenz[a]anthracene-7,12-quinone against Con A-induced blastogenesis of T lymphocytes was previously reported [Citation28]. On the other hand, to the best of our knowledge, we demonstrated the antibacterial activity of 5,6-dihydro-1,8-dihydroxy-3-methylbenz[a]anthracene-7,12-quinone against S. aureus NBRC12732T for the first time. Therefore, the inhibitory mechanism of the antimicrobial compound against S. aureus strains should be elucidated.
Conclusions
We demonstrated various actinomycetes from Calyptogena communities in the Japan Trench (depth: 5352 m) and Sagami Bay (depth: 1171 m) and isolated Actinomadura sp. DS-MS-114 producing 5,6-dihydro-1,8-dihydroxy-3-methylbenz[a]anthracene-7,12-quinone as an antibiotic compound against S. aureus. Currently, little is known concerning deep-sea actinomycetes producing physiologically active substances. Study of deep-sea actinomycetes will provide a better understanding of deep-sea biological ecology, which is essential to discover novel bioactive compounds.
Acknowledgments
The authors thank Dr. Ryuichi Sawa, Dr. Masayuki Igarashi and Dr. Masaki Hatano, Institute of Microbial Chemistry (BIKAKEN), Tokyo, Japan, for their valuable help. This work was supported by JSPS under Grant-in-Aid for Young Scientists (B) (grant number 26870731, to A.K.) and Grant-in-Aid for Scientific Research (C) (grant number 17K07736, to A.K.).
Disclosure statement
No potential conflict of interest was reported by the author(S).
Additional information
Funding
References
- Skropeta D. Deep-sea natural products. Nat Prod Rep. 2008;25:1131–1166.
- Igarashi M, Sawa R, Kinoshita N, et al. Pargamicin A, a novel cyclic peptide antibiotic from Amycolatopsis sp. J Antibiot. 2008;61:387–393.
- Sawa R, Takahashi Y, Hashizume H, et al. Amycolamicin: a novel broad-spectrum antibiotic inhibiting bacterial topoisomerase. Chem-Eur J. 2012;18:15772–15781.
- Colquhoun JA, Heald SC, Li L, et al. Taxonomy and biotransformation activities of some deep-sea actinomycetes. Extremophiles. 1998;2:269–277.
- Zotchev SB. Marine actinomycetes as an emerging resource for the drug development pipelines. J Biotechnol. 2012;158:168–175.
- Kulm L, Suess E, Moore J, et al. Oregon subduction zone: venting, fauna, and carbonates. Science. 1986;231:561–566.
- Li L, Guenzennec J, Nichols P, et al. Microbial diversity in Nankai Trough sediments at a depth of 3,843 m. J Oceanogr. 1999;55:635–642.
- Li L, Kato C, Horikoshi K. Microbial diversity in sediments collected from the deepest cold-seep area, the Japan Trench. Mar Biotechnol. 1999;1:391–400.
- Boetius A, Ravenschlag K, Schubert CJ, et al. A marine microbial consortium apparently mediating anaerobic oxidation of methane. Nature. 2000;407:623–626.
- Fujiwara Y, Kato C, Masui N, et al. Dual symbiosis in the cold-seep thyasirid clam Maorithyas hadalis from the hadal zone in the Japan Trench, western Pacific. Mar Ecol-Prog Ser. 2001;214:151–159.
- Li L, Kato C. Microbial diversity in the sediments collected from cold-seep areas and from different depths of the deep-sea. In: Horikoshi K, Tsujii K, editors. Extremophiles in deep-sea environments. Tokyo: Springer; 1999. p. 55–88.
- Kurata A, Yamaura Y, Tanaka T, et al. Antifungal peptidic compound from the deep-sea bacterium Aneurinibacillus sp. YR247. World J Microb Biot. 2017; [ cited 2017 Mar 15];33:73. DOI:10.1007/s11274-017-2239-0.
- Lane D. 16S/23S rRNA sequencing. In: Stackebrandt E, Goodfellow M, editors. Nucleic acid techniques in bacterial systematics. Chichester, UK: John Wiley & Sons; 1991. p. 115–175.
- Matarante A, Baruzzi F, Cocconcelli PS, et al. Genotyping and toxigenic potential of Bacillus subtilis and Bacillus pumilus strains occurring in industrial and artisanal cured sausages. Appl Environ Microb. 2004;70:5168–5176.
- Kimura M. A simple method for estimating evolutionary rates of base substitutions through comparative studies of nucleotide sequences. J Mol Evol. 1980;16:111–120.
- Saitou N, Nei M. The neighbor-joining method: a new method for reconstructing phylogenetic trees. Mol Biol Evol. 1987;4:406–425.
- Thompson JD, Gibson TJ, Plewniak F, et al. The CLUSTAL_X windows interface: flexible strategies for multiple sequence alignment aided by quality analysis tools. Nucleic Acids Res. 1997;25:4876–4882.
- Felsenstein J. Confidence limits on phylogenies: an approach using the bootstrap. Evolution. 1985;39:783–791.
- Lam KS. Discovery of novel metabolites from marine actinomycetes. Curr Opin Microbiol. 2006;9:245–251.
- Ward AC, Bora N. Diversity and biogeography of marine actinobacteria. Curr Opin Microbiol. 2006;9:279–286.
- Jafari N, Behroozi R, Farajzadeh D, et al. Antibacterial activity of Pseudonocardia sp. JB05, a rare salty soil actinomycete against Staphylococcus aureus. Biomed Res Int. 2014 [ cited 2014 Aug 14]; [7 p.]. DOI:10.1155/2016/6598307.
- Chambers HF, DeLeo FR. Waves of resistance: Staphylococcus aureus in the antibiotic era. Nature Rev Microbiol. 2009;7:629–641.
- Vuong C, Yeh AJ, Cheung GY, et al. Investigational drugs to treat methicillin-resistant Staphylococcus aureus. Expert Opin Investig Drugs. 2016;25:73–93.
- Badji B, Mostefaoui A, Sabaou N, et al. Identification of a new strain of Actinomadura isolated from Saharan soil and partial characterization of its antifungal compounds. Afr J Biotechnol. 2011;10:13878–13886.
- Badji B, Zitouni A, Mathieu F, et al. Antimicrobial compounds produced by Actinomadura sp. AC104 isolated from an Algerian Saharan soil. Can J Microbiol. 2006;52:373–382.
- Brown PM, Thomson RH. Naturally occurring quinones. Part XXVI. A synthesis of tetrangulol (1,8-dihydroxy-3-methylbenz [a] anthracene-7,12-quinone). J Chem Soc Perk T1. 1976;9;997–1000.
- Kaliappan KP, Ravikumar V. Angucyclinone antibiotics: total syntheses of YM-181741, (+)-ochromycinone, (+)-rubiginone B2, (-)-tetrangomycin, and MM-47755. J Org Chem. 2007;72:6116–6126.
- Yamazaki T, Nemoto K, Yamashita N, et al., inventors. Pharmaceutical compositions containing benz[a]anthracene-7,12-quinones as immunosuppressants. Japan patent JP 06345641, 1994 Dec 20.
- Lahoum A, Aouiche A, Bouras N, et al. Antifungal activity of a Saharan strain of Actinomadur a sp. ACD1 against toxigenic fungi and other pathogenic microorganisms. J Med Mycol. 2016;26:193–200.
- Nakamura G, Isono K. A new species of Actinomadura producing a polyether antibiotic, cationomycin. J Antibiot. 1983;36:1468–1472.
- Bycroft BW. Dictionary of antibiotics & related substances. London: CRC Press; 1987.
- Kakinuma S, Suzuki K, Hatori M, et al. Biosynthesis of the pradimicin family of antibiotics. J Antibiot. 1993;46:430–440.
- Hegde V, Patel M, Horan A, et al. Macrolactams: A novel class of antifungal antibiotics produced by Actinoma dura spp. SCC 1776 and SCC 1777. J Antibiot. 1992;45:624–632.