ABSTRACT
Biomineralization is a complicated process which involves multiple gene products in Pinctada fucata. Here, Pinctada fucata mantle gene 2 (pfmg2) was characterized in P. fucata. The putative PFMG2 protein has a Calponin Homology (CH) domain and an EF hand that can bind calcium. PFMG2 shares homology with transgelin in other species. Multi-tissue reverse transcriptase polymerase chain reaction (RT-PCR) indicates that pfmg2 mRNA is expressed ubiquitously, but its expression is high in the mantle tissue of P. fucata. Furthermore, in situ hybridization shows that pfmg2 localized at the edge of the mantle. Our results suggest that PFMG2 plays an important role in the shell mineralization process in pearl oyster. The analysis and the functional characterization of PFMG2 in P. fucata will provide new insight into the mineralization process.
KEYWORDS:
Introduction
The mollusk shell is a product of biomineralization that consists of a nacreous mother-of-pearl layer and a prismatic layer. Both layers are composed of CaCO3 crystals, matrix proteins and other biopolymers [Citation1]. The biomineralization in Pinctada fucata is a complicated and highly controlled process regulated by multiple genes [Citation2–9]. Investigation of this process will provide detailed insight into the steps of shell formation. Several genes have been reported to be important for this process [Citation5,Citation10–16]. In P. fucata, MSI31 and MSI60, rich in Gly and Ala, are reported to be framework proteins for the formation of CaCO3 crystals [Citation4,Citation17]. MSI7, expressed in the epithelia at the outer edge of the mantle, participates in the framework formation of the nacreous and prismatic layers [Citation8,Citation13]. Prismin 1, expressed in the mantle tissue, participates in calcite growth and has calcium-binding ability [Citation18]. KRMPs, lysine-rich matrix proteins, are involved in the shell repair process and the formation of the shell and pearl [Citation10]. A full description of processes mediating P. fucata shell formation by biomineralization requires further investigation of proteins necessary for this highly-controlled process.
P. fucata grows in the South China Sea and in the Japanese Sea. P. fucata has a high market value due to its pearl production. It has been recently reported that several genes involved in shell formation have been obtained through suppression subtractive hybridization (SSH) [Citation19,Citation20], which confirmed our SSH results [Citation21–24]. We have reported the cloning of pfmg2 (DQ104256) through SSH and nested PCR from P. fucata mantle cDNA library [Citation21], indicating that PFMG2 plays an important role in the process of biomineralization. However, the functional role of PFMG2 in shell biomineralization has not been elucidated.
In this study, the pfmg2 gene sequence and putative protein sequence were analysed in silico. In addition, multi-tissue semi-quantitative reverse transcriptase polymerase chain reaction (RT-PCR) and in situ hybridization in the mantle were performed to determine the expression pattern of pfmg2 in the tissue of P. fucata. We have determined that pfmg2 is highly expressed in the adductor muscle and epithelium of the mantle tissue, suggesting it may have an important role in shell formation.
Materials and methods
Animals
Live adult oysters, about 50–60 mm in height and 40–50 g in weight [Citation25], were purchased from Guofa Pearl Farm in Beihai, China, and maintained in aerated artificial seawater in the laboratory. Three individual samples were used in each experiment. The outer part of the mantle area was used for the experiment.
Prediction and analysis of putative protein
The amino-acid composition, isoelectric point and molecular mass of the putative PFMG2 protein were predicted using ProtParam at the ExPASy Proteomics Server, provided by the Swiss Institute of Bioinformatics (http://www.expasy.ch/). The putative conserved domain was predicted using NCBI (National Center for Biotechnology Information) BLAST (http://blast.ncbi.nlm.nih.gov/Blast.cgi). Multiple alignments and phylogenetic trees were created using Clustal W2, provided by the European Bioinformatics Institute (http://www.ebi.ac.uk/) [Citation26,Citation27]. We compared the PFMG2 protein sequence with the Transgelin protein sequence in Mus musculus, Rattus norvegicus, Bos taurus, Equus caballus, Homo sapiens, Gallus gallus, Xenopus laevis, Sparus aurata, Haliotis discus discus.
First-strand synthesis
The tissue sample was extracted and frozen in liquid nitrogen. Total RNA was extracted from P. fucata mantle, foot, adductor muscle, gill and viscera using Trizol, according to the manufacturer's recommendations (Invitrogen, USA). First-strand cDNA was synthesized using 1 μg RNA, 5 μmol/L oligo dTs as primers and 1 mmol/L deoxynucleoside triphosphate (dNTP) mix. Reactions were incubated at 65 °C for 5 min and then placed on ice for 1 min. Then 5 mmol/L MgCl2, 0.01 mol/L dithiothreitol (DTT), 2 U RNaseOUT, 10 U SuperScript III (Invitrogen, Carlsbad, CA, USA) were added immediately before incubating the reaction at 50 °C for 50 min followed by 85 °C for 5 min. This product was used as PCR template for specific gene amplification.
Semi-quantitative RT-PCR
Semi-quantitative RT-PCR was performed to investigate the tissue expression pattern of pfmg2 mRNA. Glyceraldehyde 3-phosphate dehydrogenase (Pf-gapdh) expression was analyzed as a positive control. PCR conditions were as follows: 94 °C for 90 s, 25 cycles of 94 °C for 30 s, 58 °C for 30 s and 72 °C for 1 min, followed by 5-min incubation at 72 °C (thermocycler UNO II, Biometra, GÖttingen, Germany). The following forward and reverse primers were used: Pf-gapdh 5′-GATGGTGCCGAGTATGTGGTA-3′ and 5′-CGTTGATTATCTTGGCGAGTG-3′[Citation24]. For pfmg2 mRNA, the PCR conditions were as follows: 94 °C for 90 s, 30 cycles of 94 °C for 30 s, 55 °C for 30 s and 72 °C for 1 min, followed by a 5 min incubation at 72 °C. The following forward and reverse primers were used: pfmg2 5’- ATGTCATCAACCAGAGCAAC-3’ and 5’-CTTCCTAATGCAGTTATACAAG-3’. Equal volumes of PCR products were applied to 1.5% agarose gel, stained with Gold View (SBS Genetech, China). Quantification of the relative expression of pfmg2 mRNA in the tissues of pearl oyster was done using the formula: densitometry of pfmg2/ Pf-gapdh. Scanning densitometry of the areas of interest was performed using the Molecular Analyst software quality one (Bio-Rad) to obtain the arbitrary density units in the RT-PCR experiment. RT-PCR was performed using the reference genes Pf-gapdh as internal controls. The ‘gene of interest/reference gene’ density ratio was then computed and represented in graphs [Citation28].
In situ hybridization
We performed non-radioactive in situ hybridization on paraffin sections of mantle tissues of P. fucata. Digoxigenin (DIG) labelled DNA probe, covering specific regions of the pfmg2 gene, was purchased from Invitrogen, and the slide without probe was included as a negative control. Slides were fixed with freshly prepared 4% paraformaldehyde solution for 10 min and washed by PBS. The slides were re-hydrated by sequential washing with graded ethanol solution (100–70%). Then the slides were incubated with proteinase K (100 μg/mL) in 10 mmol/L Tris-HCl pH 7.5 at 37 °C for 30 min before washing twice in water. The slides were pre-hybridized with 5 ng probe in buffer (50% formamide, 0.6 mol/L NaCl, 10 mmol/L Tris-HCl, pH 7.5, 1 mmol/L ethylenediaminetetraacetic acid (EDTA), 50 mg/mL heparin, 10 mmol/L dithiothreitol, 10% polyethylene glycol 8000, and Denhardt's solution), and incubated at 52 °C for 16 h. After that, the slides were washed with 2xSSC at 55 °C and then washed with 0.1xSSC. Nuclei were stained with Nuclear Fast Red for 5 min before further washing. Then, a labelled and hybridized probe was detected via DIG nucleic acid detection kit (Roche Molecular Biochemicals, Germany) [Citation21,Citation23].
Data analysis
Data were expressed as mean values ± standard error (SE) from at least three independent experiments. Statistical significance was computed by the unpaired Student's t-test; p < 0.05 was considered statistically significant.
Results and discussion
Sequence analysis of PFMG2
The Pfmg2 gene encodes a polypeptide with a theoretical molecular weight of 20.9 kD and an isoelectric point of 8.75 as predicted by ORF (NCBI) and ProtParam (ExPASy server), respectively. Sequence analysis revealed an EF-hand with a potential calcium-binding site located at amino acids 109–116 (109-FQTVDLYE-116). The polyadenylation signal (aataaa) is found followed by a poly(A) tail in the 3’-untranslated region (UTR) (). PFMG2 is rich in Gly (10.0%), Lys (9.5%), Leu (7.9%) and Ala (7.9%) residues (). Proteins previously reported to be important for biomineralization are also rich in glycine. Specifically, MSI60, MSI31, MSI7, Nacrein contain glycine residues at a frequency of 37.0%, 23.7%, 29.0% and 12.5%, respectively [Citation2,Citation4,Citation13], in P. fucata ().
Table 1. Predicted amino-acid composition from the coding region.
Table 2. Contents of four amino acids in PFMG2, MSI60, MSI31, MSI7 and Nacrein.
Homologic and phylogenetic analysis of PFMG2
PFMG2 was predicted to contain a Calponin Homology (CH) domain ((A), NCBI Blast). Since the function of PFMG2 is unknown in P. fucata, we used multiple sequence alignments to assign a probable function for this protein. The amino-acid sequence of PFMG2 was aligned with Transgelin protein sequences from different species and conserved regions were identified by Protein Blast at NCBI ((B)). The results showed that PFMG2 has 39% similarity (59% positive) with Bos taurus Transgelin, 39% similarity (59% positive) with Equus caballus Transgelin, 39% similarity (59% positive) with Homo sapiens Transgelin, 38% similarity (59% positive) with Rattus norvegicus Transgelin, 41% similarity (63% positive) with Gallus gallus Transgelin, 41% similarity (63% positive) with Xenopus laevis Transgelin, 38% similarity (61% positive) with Sparus aurata Transgelin, 38% similarity (59% positive) with Mus musculus Transgelin and 54% similarity (72% positives) with Haliotis discus discus Transgelin. The similarity between PFMG2 and Transgelin indicated that PFMG2 may perform similar functions as Transgelin.
Figure 2. Putative conserved sequence of the PFMG2 protein and phylogenetic analysis. (A) A putative conserved CH domain is found at the N-terminus. (B) Alignments of PFMG2 and Transgelin in Bos taurus [Bos] (NP_001039 614.1), Equus caballus [Equus] (NP_001103 604.1), Homo sapiens [Homo] (NP_001001 522.1), Rattus norvegicus [Rattus] (NP_113 737.1), Gallus gallus [Gallus] (NP_990 825.1), Xenopus laevis [Xenopus] (NP_001083 600.1), Sparus aurata [Sparus] (ADO67626.1), Mus musculus [Mus] (NP_035 656.1), Haliotis discus discus [Haliotis] (ABO26639.1). ‘*’ indicates residues that are identical in all sequences in the alignment. ‘:’ indicates the presence of conserved substitutions. ‘.’ indicates the presence of semi-conserved substitutions. (C) Phylogenetic analysis of PFMG2 protein and its closest putative homologues in different species. The phylogenetic tree shows the evolutionary relationship of the protein from various species mentioned in B.
![Figure 2. Putative conserved sequence of the PFMG2 protein and phylogenetic analysis. (A) A putative conserved CH domain is found at the N-terminus. (B) Alignments of PFMG2 and Transgelin in Bos taurus [Bos] (NP_001039 614.1), Equus caballus [Equus] (NP_001103 604.1), Homo sapiens [Homo] (NP_001001 522.1), Rattus norvegicus [Rattus] (NP_113 737.1), Gallus gallus [Gallus] (NP_990 825.1), Xenopus laevis [Xenopus] (NP_001083 600.1), Sparus aurata [Sparus] (ADO67626.1), Mus musculus [Mus] (NP_035 656.1), Haliotis discus discus [Haliotis] (ABO26639.1). ‘*’ indicates residues that are identical in all sequences in the alignment. ‘:’ indicates the presence of conserved substitutions. ‘.’ indicates the presence of semi-conserved substitutions. (C) Phylogenetic analysis of PFMG2 protein and its closest putative homologues in different species. The phylogenetic tree shows the evolutionary relationship of the protein from various species mentioned in B.](/cms/asset/5ab7ba2b-21f1-412d-8d6c-3a892eed7c1d/tbeq_a_1348254_f0002_oc.jpg)
To predict the evolutionary relationships of PFMG2 in P. fucata and Transgelin in these species, a phylogenetic tree was built using Clustal W2 to align the primary amino-acid sequences (EMBL-EBI). PFMG2 shows the highest degree of sequence similarity with Haliotis discus discus Transgelin, and is closely related to Transgelin in Sparus aurata ((C)).
Transgelin is a member of the calponin family of actin-binding proteins [Citation29]. The CH domain, an actin-binding domain, is found in many cytoskeletal and signal transduction proteins [Citation30,Citation31]. Transgelin has an N-terminal CH domain which contains a potential Ca2+-binding EF-hand [Citation29,Citation32,Citation33]. PFMG2 was predicted to have high homology with Transgelin (), which indicates that PFMG2 may bind to skeletal actin to form a protein framework for shell biomineralization. Importantly, PFMG2 was also predicted to have a CH domain at its N-terminus which contains a Ca2+-binding EF hand. Consistent with the amino-acid compositions of framework protein MSI31 and MSI60 [Citation4], PFMG2 is also rich in Gly and Ala, further suggesting that it may serve to constitute a protein framework for the nacreous and prismatic layers of P. fucata. However, whether PFMG2 binds to calcium or not and the functional role of PFMG2 in biomineralization process are of interest to further study in the shell formation.
Pfmg2 expression analysis in P. fucata
To examine the mRNA expression pattern of pfmg2 in pearl oyster, semi quantitative RT-PCR was performed. Pfmg2 mRNA was expressed in all the five tissues ((A,B)). This suggests that pfmg2 is ubiquitously expressed in pearl oyster P. fucata. However, the pfmg2 mRNA levels in the mantle and adductor muscle were much higher. This indicates that PFMG2 may play essential roles mainly in mantle and adductor muscle.
Figure 3. Pfmg2 gene expression pattern in P. fucata. (A) RT-PCR for analysis of pfmg2 gene expression in five tissues from P. fucata: 1, mantle; 2, foot; 3, adductor muscle; 4, gill; 5, viscera; gapdh was included as a positive control. The pfmg2 DNA PCR product is 395 bp in length, and Pf-gapdh is 229 bp. (B) 1, mantle; 2, foot; 3, adductor muscle; 4, gill; 5, viscera. Data are expressed as means ± SE from all experiments, as indicated **p < 0.01.
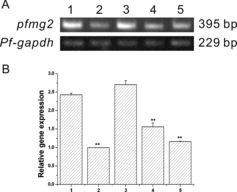
Pfmg2 expression pattern in oyster mantle tissue
In situ hybridization was performed to precisely locate the site of pfmg2 mRNA expression in mantle tissue. The signals of pfmg2 were detected in the epithelium of the mantle, and no hybridization signal was detected in other parts of the mantle ((A,B)), or in the negative control ((C,D)).
Overall, multi-tissue RT-PCR revealed that pfmg2 is expressed ubiquitously in pearl oyster (), indicating it could serve as an important framework protein for shell formation. Moreover, pfmg2 was highly expressed in the tissue of the mantle and the adductor muscle, and was specifically expressed in the epithelium of the mantle (). Since the mantle is the essential tissue for shell formation, and the epithelium is important for the formation of both layers [Citation4,Citation34,Citation35], it is predictable that PFMG2 should be important in the formation of the nacreous and prismatic layers. In addition, in the previous report, Transgelin, which binds to actin, was shown to be involved in smooth muscle contraction [Citation29]. PFMG2 has high homology with Transgelin, which indicates that it could have a potential role in adductor muscle contraction to control the mineralization process in P. fucata.
Conclusions
In this study, we reported the primary sequence characterization and mRNA expression pattern of pfmg2 in P. fucata. Interestingly, we found that PFMG2 may bind with calcium and that it has homology with Transgelin by in silico analysis. Furthermore, pfmg2 mRNA was shown to be highly expressed in the mantle and the adductor muscle tissue. In the mantle, pfmg2 was specifically expressed in the epithelium. To the best of our knowledge, these results indicate for the first time that PFMG2 is likely to be involved in calcium deposition during shell formation and to perform important functions in the biomineralization process in pearl oyster. From this study, biochemical characterization of PFMG2 will provide new insight into the mineralization process in P. fucata.
Disclosure statement
All authors have no conflicts of interest.
Additional information
Funding
References
- Wilbur KM, Watabe N. Experimental studies on calcification in molluscs and the alga Cocolithus huxleyi. Ann N Y Acad Sci. 1963;109:82–112.
- Miyamoto H, Miyashita T, Okushima M, et al. A carbonic anhydrase from the nacreous layer in oyster pearls. Proc Natl Acad Sci U S A. 1996;93:9657–9660.
- Shen X, Belcher AM, Hansma PK, et al. Molecular cloning and characterization of lustrin A, a matrix protein from shell and pearl nacre of Haliotis rufescens. J Biol Chem. 1997;272:32472–32481.
- Sudo S, Fujikawa T, Nagakura T, et al. Structures of mollusc shell framework proteins. Nature. 1997;387:563–564.
- Marin F, Corstjens P, de Gaulejac B, et al. Mucins and molluscan calcification – molecular characterization of mucoperlin, a novel mucin-like protein from the nacreous shell layer of the fan mussel Pinna nobilis (Bivalvia, Pteriomorphia). J Biol Chem. 2000;275:20667–20675.
- Xie LP, Wu YT, Dai YP, et al. A novel glycosylphosphatidylinositol-anchored alkaline phosphatase dwells in the hepatic duct of the pearl oyster, Pinctada fucata. Mar Biotechnol. 2007;9:613–623.
- Wang Z, Wang B, Chen G, et al. An alpha-2 macroglobulin in the pearl oyster Pinctada fucata: characterization and function in hemocyte phagocytosis of Vibrio alginolyticus. Fish Shellfish Immunol. 2016;55:585–594.
- Shi Y, Zheng X, Zhan X, et al. cDNA microarray analysis revealing candidate biomineralization genes of the pearl oyster, Pinctada fucata martensii. Mar Biotechnol. 2016;18:336–348.
- Du YP, Chang HH, Yang SY, et al. Study of binding interaction between Pif80 protein fragment and aragonite. Sci Rep. 2016 [ cited 2016 Aug 3]; DOI:10.1038/srep30883.
- Liang J, Xie J, Gao J, et al. Identification and characterization of the lysine-rich matrix protein family in Pinctada fucata: indicative of roles in shell formation. Mar Biotechnol. 2016;18(6):645–658.
- Samata T, Hayashi N, Kono M, et al. A new matrix protein family related to the nacreous layer formation of Pinctada fucata. FEBS Lett. 1999;462:225–229.
- Kono M, Hayashi N, Samata T. Molecular mechanism of the nacreous layer formation in Pinctada maxima. Biochem Biophys Res Commun. 2000;269:213–218.
- Zhang Y, Xie L, Meng Q, et al. A novel matrix protein participating in the nacre framework formation of pearl oyster, Pinctada fucata. Comp Biochem Physiol B. 2003;135:565–573.
- Zhao M, Shi Y, He M, et al. PfSMAD4 plays a role in biomineralization and can transduce bone morphogenetic protein-2 signals in the pearl oyster Pinctada fucata. BMC Dev Biol. 2016 [ cited 2016 Apr 26];16:9. DOI: 10.1186/s12861-016-0110-4.
- Zheng X, Cheng M, Xiang L, et al. The AP-1 transcription factor homolog Pf-AP-1 activates transcription of multiple biomineral proteins and potentially participates in Pinctada fucata biomineralization. Sci Rep. 2015 [cited 2015 Sep 25];5:14408. DOI: 10.1038/srep14408.
- Li H, Zhang B, Huang G, et al. Differential gene expression during larval metamorphic development in the pearl oyster, Pinctada fucata, based on transcriptome analysis. Int J Genomics. 2016 [ cited 2016 Oct 23];2016:2895303. DOI: 10.1155/2016/2895303.
- Sato Y, Inoue N, Ishikawa T, et al. Pearl microstructure and expression of shell matrix protein genes MSI31 and MSI60 in the pearl sac epithelium of Pinctada fucata by in situ hybridization. PLoS One. 2013 [ cited 2013 Jan 14];8:e52372. DOI: 10.1371/journal.pone.0052372.
- Takagi R, Miyashita T. Prismin: a new matrix protein family in the Japanese pearl oyster (Pinctada fucata) involved in prismatic layer formation. Zoolog Sci. 2010;27:416–426.
- Fang D, Xu G, Hu Y, et al. Identification of genes directly involved in shell formation and their functions in pearl oyster, Pinctada fucata. PLoS One. 2011 [ cited 2011 Jul 01];6:e21860. DOI: 10.1371/journal.pone.0021860.
- Wang N, Kinoshita S, Nomura N, et al. The mining of pearl formation genes in pearl oyster Pinctada fucata by cDNA suppression subtractive hybridization. Mar Biotechnol (NY). 2012;14:177–188.
- Liu HL, Liu SF, Ge YJ, et al. Identification and characterization of a biomineralization related gene PFMG1 highly expressed in the mantle of Pinctada fucata. Biochemistry. 2007;46:844–851.
- Wang X, Harimoto K, Fuji R, et al. Pinctada fucata mantle gene 4 (PFMG4) from pearl oyster mantle enhances osteoblast differentiation. Biosci Biotechnol Biochem. 2015;79:558–565.
- Wang X, Liu S, Xie L, et al. Pinctada fucata mantle gene 3 (PFMG3) promotes differentiation in mouse osteoblasts (MC3T3-E1). Comp Biochem Physiol B. 2011;158:173–180.
- Wang X, Hinshaw S, Liu S, et al. Pinctada fucata mantle gene 5 (PFMG5) from pearl oyster mantle inhibits osteoblast differentiation. Biosci Biotechnol Biochem. 2011;75:991–993.
- Zhou YJ, He ZX, Huang J, et al. Cloning and characterization of the activin like receptor 1 homolog (Pf-ALR1) in the pearl oyster, Pinctada fucata. Comp Biochem Phys B. 2010;156:158–167.
- Liu SF, Ai C, Ge ZQ, et al. Molecular cloning and bioinformatic analysis of SPATA4 gene. J Biochem Mol Biol. 2005;38:739–747.
- Liu B, Liu S, He S, et al. Cloning and expression analysis of gonadogenesis-associated gene SPATA4 from rainbow trout (Oncorhynchus mykiss). J Biochem Mol Biol. 2005;38:206–210.
- Wang X, Wang G, Liu L, et al. The mechanism of a chitosan-collagen composite film used as biomaterial support for MC3T3-E1 cell differentiation. Sci Rep. 2016 [ cited 2016 Dec 21];6:39322. DOI: 10.1038/srep39322.
- Assinder SJ, Stanton JA, Prasad PD. Transgelin: an actin-binding protein and tumour suppressor. Int J Biochem Cell Biol. 2009;41:482–486.
- Banuelos S, Saraste M, Djinovic Carugo K. Structural comparisons of calponin homology domains: implications for actin binding. Structure. 1998;6:1419–1431.
- Djinovic Carugo K, Banuelos S, Saraste M. Crystal structure of a calponin homology domain. Nat Struct Biol. 1997;4:175–179.
- Lawson D, Harrison M, Shapland C. Fibroblast transgelin and smooth muscle SM22alpha are the same protein, the expression of which is [down-regulated in many cell lines. Cell Motil Cytoskeleton. 1997;38:250–257.
- Fu Y, Liu HW, Forsythe SM, et al. Mutagenesis analysis of human SM22: characterization of actin binding. J Appl Physiol. 2000;89:1985–1990.
- Miyamoto H, Miyoshi F, Kohno J. The carbonic anhydrase domain protein nacrein is expressed in the epithelial cells of the mantle and acts as a negative regulator in calcification in the mollusc Pinctada fucata. Zoolog Sci. 2005;22:311–315.
- Suzuki M, Murayama E, Inoue H, et al. Characterization of Prismalin-14, a novel matrix protein from the prismatic layer of the Japanese pearl oyster (Pinctada fucata). Biochem J. 2004;382:205–213.