ABSTRACT
SQUAMOSA PROMOTER-BINDING PROTEIN-LIKE (SPL) transcription factor genes are functionally diverse; they control a number of fundamental aspects of plant growth and development, including vegetative phase change, flowering time, branching and leaf initiation rate. In our previous study, expression profiling showed that Bra033221, a transcript-derived fragment of an AtSPL8 ortholog, was up-regulated at flower bud differentiation stage 5. This result suggested that Bra033221 has a function similar to that of AtSPL8. In the present study, BrcSPL8, an AtSPL8 homolog, was cloned from pak choi (Brassica rapa subsp. chinensis) based on Bra033221 using reverse transcription-polymerase chain reaction (RT-PCR). The full-length cDNA was 1117 bp and contained a complete open reading frame (ORF) of 987 bp; this ORF encoded a predicted protein with 328 amino acid residues, a calculated molecular mass of 36.55 kDa and an isoelectric point of 8.85. BrcSPL8 was expressed in all analysed apices. Its expression levels before flower differentiation stage 1 were low and almost invariable, and the highest expression was detected in the apex at flower differentiation stage 5, suggesting that BrcSPL8 has a role during flower development in pak choi.
Introduction
Pak choi (Brassica rapa subsp. chinensis) is an original Chinese vegetable of the Cruciferae family. It is cultivated throughout China, and has also been recently introduced into North America, Europe and other countries in Asia [Citation1]. Premature bolting occurs during spring cultivation, and affects the product quality and yield [Citation2]. Therefore, research on the mechanism of flowering in pak choi has important theoretical and practical value.
Flower development in plants is controlled by complex and intricate gene-regulatory networks. These networks integrate information from endogenous, hormonal and environmental regulatory pathways [Citation3]. The SQUAMOSA PROMOTER-BINDING PROTEIN-LIKE (SPL) family of transcription factors plays key roles in endogenous regulatory pathways; in particular, they are involved in the age-dependent regulation of flowering in Arabidopsis thaliana [Citation4,Citation5]. High levels of SPL factors in Arabidopsis thaliana promote phase change and, subsequently, flowering [Citation6]. SPL genes were originally identified in Antirrhinum majus based on the ability of two proteins encoded by closely related genes, AmSBP1 and AmSBP2, to bind to the promoter of the floral meristem identity gene SQUAMOSA (SQUA) [Citation7,Citation8]. SPL genes are found in all green plants, including unicellular green algae, mosses, gymnosperms and angiosperms [Citation9–11]. There are 17 members of the SPL gene family in Arabidopsis thaliana. Among them, AtSPL8 was the first SPL gene to be functionally characterized [Citation12]. Mutations in AtSPL8 have a profound effect on seed set, petal trichome production, and root growth [Citation8,Citation12,Citation13]. Three independent transposon-tagged mutants have been isolated, all of which exhibit a strong reduction in fertility. In addition to its effect on micro-sporogenesis, SPL8 knockout also seems to affect megasporogenesis, sepals trichome formation and stamen filament elongation [Citation12]. In the SPL8 mutant background, a constitutively expressed SPL8 transgene cannot restore fertility. Moreover, the constitutive overexpression of SPL8 affects fertility by impairing anthers dehiscence, which likely results from a constitutive gibberellin (GA) response and suggests a positive role of SPL8 in GA-mediated anther development [Citation13].
Despite the lack of functional data, SPL8 expression patterns have been analyzed. Previous studies have shown that SPL8 genes have different expression patterns in different species. SlySBP8a and SlySBP8b, AtSPL8 co-orthologs in tomato, are highly expressed in carpels and are more highly expressed in young than in old fruits, but they show very low expression in roots, seedlings and stamens [Citation14]. Conversely, the AtSPL8 ortholog VvSBP19 in grapevine (Vitis vinifera) has not been detected to be expressed in fruits [Citation15]. In Aegilops tauschii, AeSPL8 is expressed in all organs except the shell, and its expression is highest in the stamen and pistils [Citation16]. However, TuSPL8 is expressed in the shoots, stems and stamens of Triticum urartu, and its expression is highest in the shoots and stems [Citation16]. In Betula platyphylla Suk., BplSPL8 is expressed in all tissues, but its expression is highest in the male inflorescences and lowest in the seed and pollen [Citation17]. In Betula luminifera, BlSPL8 can be detected in all organs, including the roots, stems, leaves, inflorescences, seeds, buds, phloem and xylem, with the highest transcription levels in leaves and inflorescences; however, there is almost no transcription in the seeds, phloem or xylem [Citation18]. In Sea-Island cotton, GbSBP8 is mainly expressed in the ovules [Citation19]. In addition, the expression levels of SPL8 from a cotton cytoplasmic male sterile line and maintainer line gradually decrease with pollen development [Citation20]. The expression of SmSPL8, an AtSPL8 homolog in Salvia miltiorrhiza, is highest in the flowers and lower in other organs, such as the roots, stems and leaves [Citation21].
In our previous study, expression profiling showed that Bra033221, a transcript-derived fragment of an AtSPL8 ortholog, was up-regulated at flower bud differentiation stage 5. This indicates a function for Bra033221 similar to that of AtSPL8 during floral organ development in pak choi [Citation22].
In the present study, BrcSPL8 was isolated from the shoot apices of pak choi inbred line ‘75#’ based on Bra033221 using reverse transcription-polymerase chain reaction (RT-PCR). The expression patterns in the apices at different developmental stages were analysed using quantitative real-time PCR (qPCR). An attempt was made to reveal the relationship between gene expression and flower development and to clarify the role of BrcSPL8 during flowering, which may provide a foundation for further study on the molecular mechanism of flower development in pak choi.
Materials and methods
Plant material and growth conditions
The pak choi inbred line ‘75#’, which bolts easily, was used in this study and was provided by the Institute of Vegetable Research, Shanxi Academy of Agricultural Sciences.
The germinating seeds were kept at 4 °C for 20 d for low temperature treatment. After this treatment, the cotyledon-stage seedlings were transplanted to a bowl filled with a nutritional seedling matrix and grown in a greenhouse under a 32 °C daytime maximum temperature and a 10 °C night minimum temperature with approximately 12.5 h of natural light. Standard agricultural management was practiced throughout.
Total RNA extraction and cDNA synthesis
The shoot apices at flower bud differentiation stage 5 were collected from the plants and used for total RNA extraction. Total RNA was extracted using an RNA Simple Total RNA Kit according to the manufacturer's instructions (Tiangen, DP432, China). First-strand cDNA was synthesized using TransScript® First-Strand cDNA Synthesis SuperMix for RT-PCR according to the manufacturer's instructions (TransGen, AT301, China).
Gene cloning and sequencing
To isolate the SPL8 homolog, PCR primers were designed using Primer 3 software based on the genome sequence of Brassica rapa subsp. pekinensis in the Brassica data base (http://brassicadb.org) published by the Institute of Vegetables and Flowers, Chinese Academy of Agricultural Sciences. The forward and reverse primer sequences were 5′-GTTGTAGTAGCAGAGAGCGCATA-3′ and 5′- CAACGTAATAGCTAGCGTCGTTT-3′, respectively. The SPL8 homolog was amplified by RT-PCR using PrimeSTAR® HS DNA Polymerase (TaKaRa, R010A, Japan) under the following reaction conditions: 5 min at 94 °C; 40 cycles of 15 s at 94 °C, 15 s at 55.5 °C, 2 min at 72 °C and a 5 min extension at 72 °C. The PCR fragments were cloned into the pMD19-T vector (TaKaRa, 6013, Japan) and sequenced by Sangon Biotech Co., Ltd. (Shanghai, China).
Bioinformatics analysis
The sequence was analysed using DNAMAN version 6.0 (Lynnon Biosoft Corporation, USA, www.lynon.com). For phylogenetic and sequence alignment analysis, the amino-acid sequences of SPL8 from other plants were obtained from the GenBank database (www.ncbi. nlm.nih.gov/genbank). Then, a multi-sequence alignment was conducted using DNAMAN version 6.0. A phylogenetic tree was constructed with MEGA5.1 software using the nearest neighbour-joining method (1,000 replicates for bootstrap values) and Jones-Taylor-Thornton (JTT) with the Freqs. (+F) substitution matrix model [Citation23]. In addition, the protein domain encoded by BrcSPL8 was predicted using SMART (http://smart.embl-heidelberg.de/).
Gene expression analysis
To analyse the expression patterns of BrcSPL8 during the floral development of pak choi, the BrcSPL8 expression levels in the shoot tips at different stages of flower development were assessed using real-time PCR. Total RNA used for real-time PCR was extracted from 0.1 g of tissue samples, which were collected from six plants. The stage of flower differentiation was determined as described by Song et al. [Citation1]. For reverse transcription, the concentration of each RNA sample was adjusted to 500 ng/µL. First-strand cDNA was obtained using the PrimeScript® RT reagent kit for Perfect Real Time as directed by the manufacturer (TaKaRa, DRR037A, Japan). The pak choi ACTIN gene (forward primer 5'-GTTGCTATCC AGGCTGTTCT-3' and reverse primer 5'-AGCGTGAGGAAGAGCATAAC −3') was used as a loading control. The specific primer sequences used for expression analysis were as follows: 5'- GTGTATGACCCACGCATCAC-3' and 5'- ACTTCGGTCTTGGGGAT GAG-3'. Real-time PCR reactions were repeated three times in 25 μL reactions containing 12.5 μL SYBR Premix Ex Taq, 1.0 μL forward primer (10 μmol/L), 1.0 μL reverse primer (10 μmol/L), 2 μL diluted cDNA and sterile ddH2O on an ABI 7500 instrument (ABI, California, USA). The thermal cycling parameters were as follows: 1 min at 94 °C and 40 cycles of 30 s at 94 °C, 30 s at 55 °C and 30 s at 72 °C. The relative mRNA levels of the SPL8 homolog were normalized to those of ACTIN using the 2−ΔΔCt method [Citation24].
Results and discussion
Molecular cloning and sequence analysis of BrcSPL8
A full-length SPL cDNA was obtained from the apex of pak choi by RT-PCR, and the gene was designated as BrcSPL8. The length of the PCR product was 1117 bp, and it contained a complete open reading frame (ORF) of 987 bp. The ORF encoded a predicted protein of 328 amino acid residues (), with a calculated molecular mass of 36.55 kDa and an isoelectric point of 8.85. SMART showed conserved domain features of SBP in the predicted BrcSPL8 protein, which had sequence and functional similarity to plant SPL8s.
Multiple amino-acid sequence alignment revealed homology between the predicted amino-acid sequence of BrcSPL8 and those of other proteins (). BrcSPL8 showed an amino-acid sequence similarity of 99% with BrSPL8 from Brassica rapa, 96% with BoSPL8 from Brassica oleracea var. oleracea, 95% with BnSPL8 from Brassica napus, 90% with RsSPL8 from Raphanus sativus, 85% with CsSPL8 from Camelina sativa and 70% with AtSPL8 from Arabidopsis thaliana.
Figure 2. Comparison of the predicted protein sequence of BrcSPL8 with other SPL8 proteins. The GenBank accession numbers of these proteins are as follows: AtSPL8 (BAC42797.1), BnSPL8 (CDX90071.1), BoSPL8 (XP_013584300.1), BrSPL8 (XP_009119750.1), CsSPL8 (XP_010480322.1), RsSPL8 (XP_018439145.1).
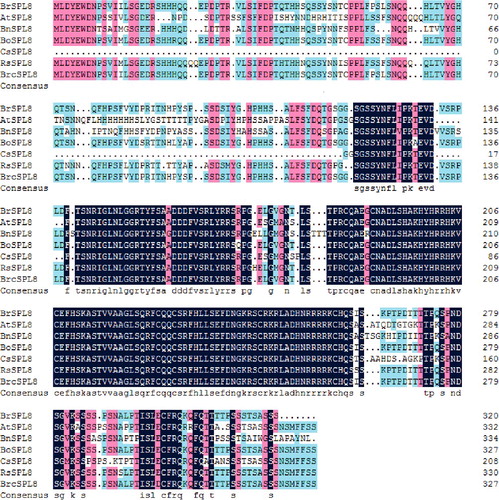
Subsequently, to evaluate the molecular evolutionary relationships among BrcSPL8 and other SPL8s, a phylogenic tree was constructed. All the SPL8 proteins from Brassicaceae species formed one cluster. BrcSPL8 was more closely related to the SPL8 proteins from cruciferous vegetables, especially Brassica vegetables ().
BrcSPL8 gene expression in the process of flower development
Real-time PCR was performed to determine the expression level of BrcSPL8 in the apex during different stages of flower development (). BrcSPL8 transcripts were produced in all analysed apices. The expression levels before flower differentiation stage 1 were low and almost invariable. However, a dramatic elevation was observed at flower differentiation stage 5. The expression was highest at stage 5. Previous studies have shown that SPL transcription factors activate the transcription of floral integrator genes, such as SOC1, and floral meristem identity genes, such as LEAFY (LFY), AP1 and FRUITFUL (FUL) [Citation5,Citation25,Citation26]. The trend in BrcSPL8 expression was consistent with the expression of BrcLFY [Citation1]. GmSPL8a and GmSPL8b are highly expressed in flowers and young leaves, whereas their expression is reduced in roots and seeds [Citation27], which indicates that GmSPL8a and GmSPL8b play an important role during floral development in soybean. These results suggest that BrcSPL8 plays a role during flower development in pak choi.
Conclusions
In this study, an SPL8 gene, BrcSPL8, was isolated from the pak choi apex at stage 5 of flower bud differentiation. A phylogenetic analysis and sequence alignment of BrcSPL8 were performed. In addition, the expression pattern of BrcSPL8 in the apex at different flower development stages was analysed using qPCR. The expression was the highest at flower bud differentiation stage 5. These results showed that BrcSPL8 plays a role during flower development and will facilitate research on the flower development mechanisms in pak choi.
Disclosure
statementNo potential conflict of interest was reported by the authors.
Additional information
Funding
References
- Song HX, Fu C, Yin LH, et al. Cloning and expression analysis of LEAFY homologue in Pak Choi (Brassica rapa subsp. chinensis). Biotechnol Biotechnol Equip. 2015;29(6):1035–1042.
- Liu RY, Hou LP, Wang L, et al. [ Effect analysis of low temperature promoting flowering in Chinese cabbage]. ACTA Agriculturae Boreali-Sinica. 2009;24(6):193–197. Chinese.
- Wils CR, Kaufmann K. Gene-regulatory networks controlling inflorescence and flower development in Arabidopsis thaliana. BBA-Gene Regul Mech. 2017;1860(1):95–105.
- Amasino R. Seasonal and developmental timing of flowering. Plant J. 2010;61(6):1001–1013.
- Fornara F, Coupland G. Plant phase transitions make a SPLash. Cell. 2009;138(4):625–627.
- Jarillo JA, Pineiro M. Timing is everything in plant development. The central role of floral repressors. Plant Sci. 2011;181(4):364–378.
- Klein J, Saedler H, Huijser P. A new family of DNA binding proteins includes putative transcriptional regulators of the Antirrhinum majus floral meristem identity gene SQUAMOSA. Mol Gen Genet. 1996;250(1):7–16.
- Preston JC, Hileman LC. Functional evolution in the plant SQUAMOSA-PROMOTER BINDING PROTEIN-LIKE (SPL) gene family. Front Plant Sci. 2013 [cited 2017 Sep 01];4:80. DOI:10.3389/fpls.2013.00080
- Cardon GH, Hohmann S, Nettesheim K, et al. Functional analysis of the Arabidopsis thaliana SBP-box gene SPL3: a novel gene involved in the floral transition. Plant J. 1997;12:367–377.
- Arazi T, Talmor-Neiman M, Stav R, et al. Cloning and characterization of micro-RNAs from moss. Plant J. 2005;43(6):837–848.
- Riese M, Zobell O, Saedler H, et al. SBP-domain transcription factors as possible effectors of cryptochrome-mediated blue light signalling in the moss Physcomitrella patens. Planta. 2007;227(2):505–515.
- Unte US, Sorensen AM, Pesaresi P, et al. SPL8, an SBP-box gene that affects pollen sac development in Arabidopsis. Plant Cell. 2003;15(4):1009–1019.
- Zhang Y, Schwarz S, Saedler H, et al. SPL8, a local regulator in a subset of gibberellin-mediated developmental processes in Arabidopsis. Plant Mol Biol. 2007;63(3):429–439.
- Salinas M, Xing S, Hohmann S, et al. Genomic organization, phylogenetic comparison and differential expression of the SBP-box family of transcription factors in tomato. Planta. 2012;235(6):1171–1184.
- Wang Y, Hu Z, Yang Y, et al. Genome-wide identification, phylogeny, and expression analysis of the SBP-box gene family in grapevine. Russ J Plant Physiol. 2010;57(2):273–282.
- Wang BN. Comparative analysis and functional study of SQUAMOSA promoter binding protein-Like (SPL) genes in wheat [master's thesis]. Beijing: Chinese Academy of Agricultural Sciences; 2015.
- Guan MX, Liu XM, Zhang Y, et al. [ Isolation and transcription expression analysis of SPL8 transcription factors gene of Betula platyphylla]. J Nanjing Forest Univ (Nat Sci Edn). 2013;37(3):17–22. Chinese.
- Niu MY. Cloning, expression and functional analysis of flowering related SPL gene in Betula luminifera [master's thesis]. Lin'an: Zhejiang A&F University; 2015.
- Xin J. Cloning and expression analyses of the transcription factor GbSBP8 involved in fiber development of sea-island cotton [master's thesis]. Shanghai: Shanghai Jiao Tong University; 2007.
- Pei WF. Gossypium harknessii cytoplasmic male sterile line and its maintainer line [master's thesis]. Beijing: Chinese Academy of Agricultural Sciences; 2013.
- Zhang LS. Clone and analysis of secondary metabolism related genes: SmKSL3 and SmSPLs in Salvia miltiorrhiza [dissertation]. Guiyang: Guizhou University; 2015.
- Song HX, Ping AM, Sun MX, et al. Identification of genes related to floral organ development in pak choi by expression profile. Genet Mol Res. 2017 [cited 2017 Apr 17];16(1): gmr16019233. DOI:10.4238/gmr16019233
- Tamura K, Peterson D, Peterson N, et al. MEGA5: molecular evolutionary genetics analysis using maximum likelihood, evolutionary distance, and maximum parsimony methods. Mol Biol Evol. 2011;28(10):2731–2739.
- Livak KJ, Schmittgen TD. Analysis of relative gene expression data using real-time quantitative PCR and the 2−ΔΔCT method. Methods. 2001;25(4):402–408.
- Wang JW, Czech B, Weigel D. miR156-regulated SPL transcription factors define an endogenous flowering pathway in Arabidopsis thaliana. Cell. 2009;138(4):738–749.
- Wu G, Park MY, Conway SR, et al. The sequential action of miR156 and miR172 regulates developmental timing in Arabidopsis. Cell. 2009;138(4):750–759.
- Tripathi RK, Goel R, Kumari S, et al. Genomic organization, phylogenetic comparison, and expression profiles of the SPL family genes and their regulation in soybean. Dev Genes Evol. 2017;227(2):101–119.