ABSTRACT
The process of methanogenesis, which is in the essence of the widely distributed technologies for biogas production, is slow, has multiple steps and occurs under anaerobic conditions. These factors make the control and the management of the anaerobic digestion difficult and impose the testing and implementation of new indicators for control which are fast and focused on the activity and functioning of the methanogenic community in addition to the traditionally used technological parameters. In this study, the biogas production process in wastewater treatment plant ‘Kubratovo’ was analysed in two different seasonal situations and a correlation was made between the studied parameters for control. The complex methodological arsenal included the activities of aerobic and anaerobic dehydrogenase, fluorescent image analysis and key chemical and technological indicators. The correlation analysis showed that the fluorescent image analysis parameters (number/mean size of clusters; fluorescence intensity; area) had the strongest relationship with the biogas production. Based on these results, we propose a fluorescent indicative system for control of the biogas production technologies. It is a fast tool for assessment the effectiveness of the process of anaerobic digestion. Its focus on the activity and functioning of the biological system could contribute to the optimization of the whole performance of these technologies and could serve as a prediction tool for potential problems in the future.
Introduction
The essence of the technologies for biogas production is the process of anaerobic digestion. It is performed by a syntrophic and synergistic microbial consortium and consists of four stages: hydrolysis, acidogenesis, acetogenesis and methanogenesis [Citation1,Citation2]. The process is slow and occurs under anaerobic conditions [Citation3]. The products from the metabolism of each group of micro-organisms play the role of substrates for the micro-organisms of the next step of the process [Citation4]. Each of the four microbial functional groups has different optimal ambient parameters. The key micro-organisms of biogas production are the methanogens [Citation5]. They belong to the domain Archaea and are particularly sensitive to changes in the environment such as temperature and pH fluctuations. Their growth and activity could be inhibited by the presence of high levels of volatile fatty acids, hydrogen, ammonia, micro-nutrients and other compounds in their environment [Citation6–8]. All these factors make the control and the management of the anaerobic digestion difficult and impose the testing and implementation of new indicators for control which are fast and focused on the activity and functioning of the methanogenic community involved in the biological process of biogas production in addition to the traditionally used technological parameters.
One of the frequently used indicators for functional control of the biological processes is enzyme activities. These enzymes catalyze the main, additional and alternative metabolic pathways. Several enzymes participate in the multi-step process of anaerobic digestion but some of them can be used as indicators of the overall metabolic activity of the microbial community. The total dehydrogenase activity (DHA) is a well-verified indicator for the total metabolic condition of the biological systems. It gives information about the biodegradation rate of the conventional and toxic pollutants. Knowledge of the key players and associated metabolic pathways involved in each stage of the process is required to effectively manage the process of biogas production [Citation9]. DHA activity can be determined by the use of different tetrazolium salts. Those most used in the practice are: 3,4,5-triphenyl tetrazolium chloride (TTC); 2-(р-iodophenyl)−3-(р-nitrophenyl)−5-phenyl tetrazolium chloride (INT); 5-cyano-2,3-diotil tetrazolium chloride (CTC), etc. These salts are colourless and after reduction by means of the dehydrogenases, are transformed to water insoluble monoformazans in microbial cells. They are characterized by a stable red colour, which is determined quantitatively by spectrophotometry [Citation10–12]. Despite the fact that the total DHA activity is a proven indicator for the total microbial activity, the search for more specific bio-indicators of the rate of biogas production still continues.
The application of fluorescent techniques in the control of biological processes increases and more and more new methods are used. In anaerobic environments, coenzyme F420 is associated exclusively with methanogenic bacteria. It occurs in all methanogens. It emits blue-green fluorescent light when irradiated with ultraviolet (UV) light. The methane producers can be distinguished from other micro-organisms by using a fluorescent microscope and excitation with UV light [Citation13,Citation14]. Another analytical approach in biotechnology, in particularly in wastewater treatment and anaerobic digestion, is quantitative image analysis. It circumvents the tedious and time-consuming traditional techniques. It is based on extraction of information from digital images. The images constitute a sum of pixels, which, after computer processing and analysis, allow the extraction of rigorous morphological quantitative data. Computers can extract quantitative information better than humans and may reduce the bias in image interpretation [Citation15].
The aim of this study was to analyse the process of methanogenesis in the wastewater treatment plant (WWTP) ‘Kubratovo’ (Bulgaria) in two different seasonal situations, to explore the correlation between the studied parameters for control and to propose a fast tool that represents the biogas production. The complex methodological arsenal included the activities of aerobic and anaerobic dehydrogenase, fluorescent image analysis and key chemical and technological indicators.
Materials and methods
The samples analysed in this study were taken from the full-scale digesters of WWTP ‘Kubratovo’ (), which use sludge formed in the process of wastewater treatment as a substrate. The WWTP operates four mesophilic digesters (D1, D2, D3, D4) with gas mixing, a volume of 7000 m3 each, working at 100%. There are two streams of redundant sludge to the digesters: primary and secondary sludge. The first one goes directly to the digesters, whereas the secondary sludge undergoes a mechanical pre-treatment step and addition of flocculant in order to become thickened. In this study, two different situations (different periods of sampling) were analysed: the first sampling was in the summer (in July) and the second one, in the autumn (in October) (). The control points by place that were identified were from the inside of the digesters where the process of methanogenesis is in its active phase. The average retention time in the digesters of WWTP ‘Kubratovo’ is 14–16 days. The indicators investigated for comparative analyses included key physicochemical indicators (temperature, pH, chemical oxygen demand (COD), concentration of inorganic ammonium and phosphate), technological (dry matter (DM), organic DM (ODM), retention time, yield of biogas and methane content in the biogas), enzymological (total aerobic dehydrogenase activity (aeDHA), total anaerobic dehydrogenase activity (anDHA)) and fluorescent indicators (fluorescence of co-factor F420).
Table 1. Characteristics of the analysed technologies for biogas production
The samples from the digesters and the data about the temperature, the retention time, the ratio of volatile fatty acids to the total alkalinity (VFA/TA, aka. FOS/TAC), the biogas yield and the methane content were provided by ‘Sofiyska voda’ AD (Sofia, Bulgaria).
The total biogas production was presented as cubic metres of biogas per day (m3/d) and the specific biogas production, as m3 biogas/kgDM/d. The pH, the COD, the concentration of the ammonium ions and the phosphates were analysed according to APHA (American Public Health Association) [Citation16]. For the analyses of DM and ODM, a weight method was used, with drying at 105 and 550 °C, respectively. The aeDHA and the anDHA values were used as indicators for the total metabolic activity of the biomass in the digesters. The dehydrogenase activities were measured spectrophotometrically: the aeDHA activity, by using TTC [Citation17,Citation18]; and the anDHA activity, by using INT [Citation11]. The results for both DHAs were presented as µmolH+/min·g protein/g DM. The protein concentration in the cell extracts was measured by the micro-biuret method according to Kochetov [Citation19]. All data are average values from at least three independent measurements.
The fluorescence of co-factor F420 was determined by excitation with UV light on a fluorescent microscope Leica. Images of the observed objects were made at 100× magnification (real image size of 1283 × 958 μm) and 400× (real image size of 321 × 240 μm). The images were processed by the software program daime and were analysed regarding the following parameters: mean fluorescent intensity of the images (of the images with 400× magnification), area of the fluorescent objects as part of the total area of the images (of the images with 400× magnification), number and mean size of the fluorescent objects (of the images with 100× magnification). The latter two parameters refer to the formation of clusters of the biological system in the digesters due to the synergistic and syntrophic relationships of the complex microbial consortia. The intensity (brightness) of objects in the analysed colour images was measured in daime (Version 2.1) program [Citation20] by taking the maximal intensity of each pixel (voxel) in any colour channel. The intensity varies between zero and an upper limit depending on the bit depth of the image. As a comparative parameter in this study, the mean intensity of each image was analysed. The obtained data are average values from the analysis of at least three images of each sample at each of the mentioned magnifications.
Correlation analysis was performed using Microsoft Office Excel 2013.
Results and discussion
Chemical and technological parameters
The physicochemical and technological parameters measured in the studied digesters are presented in . There were no significant differences in the values of the analysed parameters in the two sampling seasons, except in the concentration of the phosphates, which increased in the autumn sampling. The ratio of the phosphates and the nitrogen in the anaerobic digestion has been reported to be 1/5–1/7 [Citation21]. In the present study, the ratio between P-PO4 and N-NH4+ (as the main inorganic form of nitrogen) in the summer sampling period was approximately 1:4–1:5 for the four digesters, and changed to approximately 1:2–1:3 in the autumn sampling. There are multiple reasons that could explain the increase of the phosphates. First of all, the concentration of phosphates and of other nutrients is directly related to their concentration in the incoming water at the entrance of the WWTP and their level of biodegradation during the water treatment process. The period August–October is characterized with low water level. The autumn is also associated with the end of the vegetative season and a decrease in the speed of the biotransformation processes. According to the general biodegrading principles and the natural geochemical cycles of carbon and phosphorus, the self-purification process is related to the increase of phosphorus concentrations. Moreover, in the summer season, a lot of phosphorus fertilizers are used in agriculture. The high water level, which causes dilution of waters during the spring season, and the phosphorus bio-assimilation in the water basins in the summer lead, respectively, to a decrease in the phosphorus concentrations [Citation22]. These seasonal fluctuations affect the water treatment processes in WWTPs and the nutrient accumulation in the sludge. All these factors could complexly impact the increase in the phosphorus concentration. Higher concentrations of phosphates during the autumn and the winter are a frequent problem in WWTPs and, in many cases, chemical reagents such as FeCl3 need to be added to the biobasins in order to eliminate the phosphates [Citation14]. Their biotransformation requires sufficient carbon content [Citation13]. In this study, there was a decrease in the C:N:P ratio measured as COD:N-NH4+:P-PO43− in all of the digesters during the autumn season. It changed from the summer to the autumn as follows: D1 – from 46:4:1 to 29:2:1; D2 – from 50:4:1 to 29:2:1; D3 – from 58:5:1 to 33:3:1; D4 – from 50:4:1 to 25:2:1. In anaerobic conditions, the phosphorus-accumulating bacteria release phosphates so their biotransformation in the anaerobic digesters requires even higher carbon content (from 120:3:1 to 330:5:1) than for the aerobic water treatment processes (C:N:P = 100:5:1) [Citation23–26]. The decrease in the C:N:P ratio could be a reason for the decrease in the biogas production in the autumn sampling.
Table 2. Physicochemical and technological parameters of the process in the digesters of WWTP ‘Kubratovo’.
The other important nutrient in the biodegradation processes is nitrogen. Ammonia is considered as an inhibitor of the anaerobic digestion process. The methanogenic micro-organisms are much more sensitive to ammonia than the hydrolytic, acidogenic and acetogenic bacteria. Actually, low concentrations of ammonia <200 mg/L are beneficiary for the anaerobic micro-organisms because they need nitrogen as a nutrient for their growth [Citation27]. Ammonium (NH4+) concentrations over 1700 mg/L are reportedly inhibitory for the process of methanogenesis. The inhibitory effect depends on other physicochemical conditions such as pH (over 7.6), temperature and biomass acclimation [Citation28]. The ammonium ion concentrations (from 1299.23 to 1479.8 mg/L) in the analysed samples were not as high as the inhibitory concentrations but were close to the threshold. The C:N ratio measured as COD:N-NH4+ remained stable during the two sampling periods in all of the digesters (it varied between 11:1 and 13:1). The FOS/TAC ratio ranging from 0.3 to 0.4 indicated that the biogas production was at its maximum and the biomass input should be kept constant. The values over 0.4 (Digester 2 and 4 from the July sampling and Digesters 1 and 4 from the October sampling) indicated that the plant might be overflowing and should be closely monitored [Citation29]. The pH values were within their optimum range in all the analysed situations.
Enzymological parameters
The results from the analyses of the aeDHA and the anDHA activities in the four digesters in the two sampling periods are shown in . The ratio between the aeDHA and anDHA activity was also analysed, as well as its relation to the biogas production. The total biogas yield varied between 7058 and 11,368 m3/day in the eight analysed samples. There was a decrease in the biogas production during the autumn season in each of the digesters. The aeDHA activity increased in all the studied digesters during the autumn sampling period. The same trend was observed for the anDHA activity, with the exception of the fourth digester, in which the anDHA activity maintained its value. The tetrazolium salts (TTC and INT) used in the determination of the dehydrogenase activities are involved in the electron transport chains, the place of INT preceding that of TTC. That means that the aeDHA activity is more common as an indicator and should include in itself the anDHA activity. The results obtained in this study showed that the aeDHA activity values were lower than these of the anDHA activity. This could be due to inhibition of the pathway in the electron transport chains after the position of INT due to the strictly anaerobic conditions.
Figure 2. Relationship between the biogas production and aeDHA (a) or anDHA (b) in the eight analysed samples.
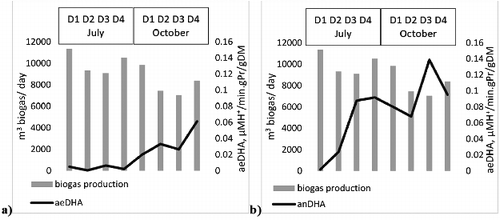
The relationship between the biogas production and the ratio between the analysed enzymatic activities is illustrated in . On the one hand, the ratio between the aeDHA and the anDHA activity shows the level of redox potential (a lower ratio is associated with a lower redox potential and more anaerobic conditions, whereas a higher ratio, with a higher redox potential and more anoxic or aerobic conditions). On the other hand, the ratio should be an indicator of the functioning of the methanogenic consortium. There was an increase in the aeDHA/anDHA ratio in the autumn sampling period in three of the digesters (D2; D3; D4) due to a 10-fold increase in the aeDHA activity in D3 and D4 and a 20-fold increase in D2 (). Oppositely, this ratio was much higher in the summer sampling in D1 than in the autumn one due to a 10-fold lower anDHA activity in July. The increase in this ratio indicates that the process was not in a terminal stage of anaerobic digestion. Since the digesters operated in WWTP ‘Kubratovo’ are of the one-stage type, all the phases of the biogas production process take place in one tank and are not separated. The obtained higher results for the aeDHA/anDHA ratio could be attributed to the fact that, at the moment of sampling, the process had been in an earlier fermentation phase in which the anaerobic conditions are not obligatory.
Figure 3. Relationship between the biogas production and the ratio aeDHA/anDHA in the eight analysed samples.
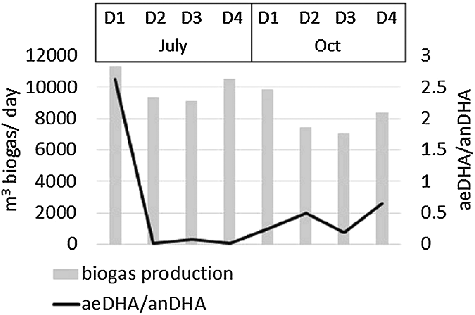
The high negative correlation between the aeDHA activity and the biogas production and between the anDHA activity and the biogas production indicated that these enzymological indicators are not specific for the methanogenic phase but more likely for the fermentative phase of methanogenesis. This suggestion was supported by the relatively low correlation between these enzyme activities and the specific biogas production. In this case, the biogas production per gram DM indicated that the key transformation of the substrate was in the fermentative phase. Similar results were obtained regarding the aeDHA/anDHA ratio. This ratio correlated positively with the total biogas production (r = 0.49) and had low negative correlation (r = −0.22) with the specific biogas production. In this case, a biological explanation could also be that these enzyme activities specifically indicate the fermentative phase, i.e. the preparation of the substrates for the methanogenic phase, and are not so suitable as indicators for the methanogenic phase itself. This guided our studies towards evaluation of fluorescent techniques, and specifically co-factor F420 fluoresce, for indication of the process.
Fluorescent image analysis
Representative fluorescent images of the biological system of each of the digesters in the two sampling periods are presented in , along with the results of the image analysis, the total and specific biogas yield and the methane quantity in the produced biogas. There was a decrease in the biogas yield in all of the digesters during the autumn sampling, except in the first one, where the specific biogas production increased. The methane quantity was stable in all of the digesters. There was a decrease in the fluorescence intensity in all the digesters from the summer to the autumn season, except in the digester, which corresponds to the results for the specific biogas yield and could be an indication for a reduced activity of the microbiological consortium.
Table 3. Fluorescent image analysis of the biological system in the two sampling periods of the four digesters of WWTP ‘Kubratovo’ vs. total and specific biogas production and methane content (%) in the biogas.
The determination of the technological, enzymological and fluorescent parameters for control in the digesters of WWTP ‘Kubratovo’ was followed by analysis of the relationship of each one of them to the biogas production. shows the coefficients of correlation between the indicators of the anaerobic digestion analysed in this study and the total and specific biogas yield. The highest correlation was that between the specific biogas production and the ratio number/size of clusters, the fluorescence intensity and the area of the fluorescent objects defined through the fluorescent image analysis. The low ratio number/size of clusters indicates that the clusters are not as large in number as they are in size, or in other words, that the microbial consortium is changing its structure and is forming active zones with concentration of synergistic and syntrophic relationships, where the methanogenic activity and biogas production is high. These formations (clusters) are constituted of different groups of micro-organisms which collaboratively degrade the organic matter and contribute to the increase of the biogas production by the methanogens. It could be concluded that these cooperative relationships between the micro-organisms are responsible for the high biogas production and the production of high quality biogas (with high per cent of methane in it). The lower the ratio number/size of the clusters is, the higher the biogas production is. The positive correlation between the fluorescence intensity and the specific biogas yield indicates that the higher the intensity is, the higher the activity of the microbial consortium and respectively the biogas production is. This demonstrates that the fluorescence of co-factor F420 corresponds to the condition of the microbial community performing the process of methanogenesis.
Table 4. Correlation between the analysed technological and functional parameters for control and the total and specific biogas production.
High correlation was also observed between the ODM and both types of biogas production. There were values over the medium level of correlation with the aeDHA activity (for the total and specific biogas yield) and the anDHA activity (only for the total biogas yield). The correlation analysis indicated that the aeDHA and anDHA activities were not appropriate indicators for fast control and exact identification of the condition of the microbial system in the digesters. Higher correlation with the biogas production could be sought with some of the other enzymes participating in the multistep process of methanogenesis and especially with the enzymes participating in the final steps of biogas production. The aeDHA and anDHA activities could be applied as additional indicators for assessment of the process in a detailed monitoring process.
Based on the obtained results, we suggest that the combination between the parameters of the fluorescent image analysis could give a fast and objective idea of the effectiveness of a digester's performance. To this end, a fluorescent indicative system was defined for control of the technologies for biogas production (). The elements for assessment the effectiveness of the performance of the digesters included in this system are the fluorescence intensity of the biological system and the ratio number/mean size of clusters formed from the microbial consortium. The performance of the four digesters of WWTP ‘Kubratovo’ in the two sampling periods was assessed as being highly effective, which confirms the fact that the whole WWTP covers its electricity needs with 111% due to the process of anaerobic digestion. The higher fluorescence intensity is an indicator for eminent activity of the biological system, and the lower values of the ratio number/mean size of clusters correspond to the formation of strong synergistic and syntrophic relationships in the microbial consortium. The performance of the third digester in the autumn sampling period was marginal between highly effective and satisfactorily effective. The zones where one of the parameters is not within its optimum values are defined as satisfactorily effective; however, in these cases, there is a need of strict monitoring of the process. The ineffective zone will include biological systems with low fluorescence intensity and weak synergistic and syntrophic relationships.
represents the same chart from above but enlarged so that the area engaged by the microbiological consortium measured by the image analysis can be seen. The size of the bubbles corresponds to the area as per cent of the fluorescent objects. It confirms the significance of this control parameter because, as it can be seen, the smaller the area percentage is, the closer the studied digesters are to the satisfactory zones. An essential element in the presented fluorescent indicative system for assessment the effectiveness of the performance of digesters is the use of co-factor F420. To the best of our knowledge, this is the first time such kind of assessment for control and management of the methanogenic processes is applied in a real full-scale technology for biogas production.
Conclusions
The analysed functional parameters for control of the technologies for biogas production had higher correlation to the biogas production than the traditionally used technological parameters. This makes them potential indicators for the process performance. The specific biogas yield showed highest correlation with the elements of the image analysis. The proposed fluorescent indicative system is a fast tool for assessment of the effectiveness of digesters. The analysis of the activity and the functioning of the biological system gave an adequate and exact picture of the process performance and could be used to predict potential future problems in the system. The identified relationship between the metabolic activity of the methanogenic consortium and the biogas production is a biotechnological solution for control and improvement of the process in such technologies. Future studies will focus on verification of this indicative system in other technologies for biogas production and deepening our understanding of the structure of the methanogenic communities by applying fluorescent in situ hybridization analysis for localization of the different species of methanogens in the clusters.
Acknowledgments
The authors express gratitude to the management team and the operators of WWTP ‘Kubratovo’ for providing the samples and sharing data about the technological parameters and the functioning of the process of biogas production.
Disclosure statement
The authors report no conflicts of interest.
Additional information
Funding
References
- Gaida D, Wolf C, Bongards M. Feed control of anaerobic digestion processes for renewable energy production: a review. Renew Sustain Energy Rev. 2017;68:869–875.
- Venkiteshwaran K, Bocher B, Maki J, et al. Relating anaerobic digestion microbial community and process function. Microbio Insights. 2015;8(S2):37–44.
- Komilis D, Barrena R, Grando RL, et al. A state of the art literature review on anaerobic digestion of food waste: influential operating parameters on methane yield. Rev Environ Sci Biotechnol. 2017;16(2):347–360.
- Ziemiński K, Frąc M. Methane fermentation process as anaerobic digestion of biomass: transformations, stages and microorganisms. Afr J Biotechnol. 2012;11(18):4127–4139.
- Lambrecht J, Cichocki N, Hübschmann T, et al. Flow cytometric quantification, sorting and sequencing of methanogenic archaea based on F420 autofluorescence. Microb Cell Fact. 2017; [cited 2017 Nov 17];16:180. DOI: 10.1186/s12934-017-0793-7
- Fotidis, IA, Karakashev D, Angelidaki I. The dominant acetate degradation pathway/methanogenic composition in full-scale anaerobic digesters operating under different ammonia levels. Int J Environ Sci Technol. 2014;11(7):2087–2094.
- Romero-Güiza MS, Vila J, Mata-Alvarez J, et al. The role of additives on anaerobic digestion: a review. Renew and Sustain Energy Rev. 2016;58:1486–1499.
- Wang P, Wang H, Qiu Y, et al. Microbial characteristics in anaerobic digestion process of food waste for methane production–A review. Biores Tech. 2018;248(Pt A):29–36.
- De Vrieze, Raort L, Roume H, et al. The full-scale anaerobic digestion microbiome is represented by specific marker populations. Water Res. 2016;104:101–110.
- Casida LE. Microbial metabolic activity in soil as measured by dehydrogenase determinations. Appl and Environ Microbiol. 1977;34(6):630–636.
- Caravelli A, Giannuzzi L, Zaritzky N. Effect of chlorine on filamentous microorganisms present in activated sludge as evaluated by respirometry and INT-dehydrogenase activity. Water Res. 2004;38(9):2395–2405.
- Gruden CL, et al. 5-Cyano-2, 3-ditolyl tetrazolium chloride (CTC) reduction in a mesophilic anaerobic digester: measuring redox behavior, differentiating abiotic reduction, and comparing FISH response as an activity indicator. J of microbiol methods. 2003;52(1):59–68.
- Gerardi M. The microbiology of anaerobic digesters. New Jersey: (NJ): Wiley; 2003.
- Bitton G. Wastewater microbiology. 4th ed. New Jerswy: (NJ): Wiley; 2011.
- Costa JC, Mesquita DP, Amaral AL, et al. Quantitative image analysis for the characterization of microbial aggregates in biological wastewater treatment: a review. Environ Sci Pollut Res. 2013;20(9):5887–5912.
- American Public Health Association. Standard methods for the examination of water and wastewaters. Washington (D.C.): American Public Health Association; 2012.
- Lenhard G. Die Dehydrogenaseaktivität des Bodens als Maß für die Mikroorganismentätigkeit im Boden [The dehydrogenase activity of the soil as a measure of the microorganism activity]. J Plant Nutr Soil Sci. 1956;73(1):1–11. German.
- Lenhard G, Nourse LD, Schwarz HM. The measurement of dehydrogenase activity of activated sludges. Advances in Water Pollution Research. In: Jaag O, Baars JK, Pearson EA, editors. Proceedings of the 2nd International Conference. Aug 1964; Tokyo, Japan. Oxford: Pergamon Press; 1965. p. 105–119.
- Kochetov GA. [Manual on enzymology]. Moscow: (Russian): High School; 1980.
- Daims H, Lücker S, Wagner M. daime, a novel image analysis program for microbial ecology and biofilm research. Environ Microbiol. 2006;8:200–213.
- de Lamos Chernicharo CA. Anaerobic reactors. New Delhi: IWA Publishing; 2007. ( Biological wastewater treatment series; Vol. 4.)
- Howell JA. The distribution of phosphorus in sediment and water downstream from a sewage treatment works. Biosci Horiz Int J Student Res. 2010;3(2):113–123.
- Rajeshwari KV, Balakrishnan M, Kansal A, et al. State-of-the-art of anaerobic digestion technology for industrial wastewater treatment. Renew Sustain Energy Rev. 2000;4:135–156.
- Weiland P. Biogas production: current state and perspectives. Appl Microbiol Biotechnol. 2010;85:849–860.
- Ghasimi SMD, Idris A, Chuah TG, et al. The effect of C:N:P ratio, volatile fatty acids and Na+ levels on the performance of an anaerobic treatment of fresh leachate from municipal solid waste transfer station. Afr J Biotechnol. 2009;8(18):4572–4581.
- Kamali M, Gameiro T, Costa MEV, et al. Anaerobic digestion of pulp and paper mill wastes–An overview of the developments and improvement opportunities. Chem Eng J. 2016;298:162–182.
- Cheng J. Biomass to renewable energy processes. New York (NY): Taylor & Francis; 2010.
- Ortner M, Drosg B, Stoyanova E, et al. Industrial residues for biomethane production. In: Korres N, O'Kiley P, Benzie AH, et al., editors. Bioenergy production by anaerobic digestion: using agricultural biomass and organic wastes. Oxon (OX): Routledge; 2013. p. 111–136.
- Lili M, Biró G, Sulyok E, et al. Novel approach on the basis of FOS/TAC method. In: International symposia ‘risk factors for environment and food safety’ & ‘natural resources and sustainable development’ & ‘50 years of agriculture researche in Oradea’. 2011 Nov 4–5; Oradea, Romania. Oradea (Romania): University of Oradea, Faculty of Environmental Protection; 2011. p. 802–807.