ABSTRACT
We reported previously that EGAM1 homeoproteins (EGAM1 and EGAM1N), transcribed from the Crxos gene as splicing variants, are expressed in preimplantation mouse embryos and mouse embryonic stem (ES) cells. Exogenous expression of these proteins affects the maintenance of an undifferentiated state and the progression of differentiation in mouse ES cells. Human tetrapeptide-repeat homeobox 1 (TPRX1), a member of the eutherian totipotent cell homeobox (ETCHbox) genes, is an ortholog of Crxos. However, the roles of TPRX1 in the differentiation of human pluripotent cells are still unknown. Because the TPRX1 transcripts were undetectable in an undifferentiated state and during the progression of differentiation in wild-type human embryonal carcinoma NT2/D1 cells, it would be advantageous to clarify the relationship between the exogenous expression of TPRX1 and the induction of genes encoding lineage-specific transcription factors in pluripotent cells. The expression of GATA6 and FOXA2, crucial transcription factors for the formation of the primitive endoderm, was upregulated, whereas that of CDX2, a crucial transcription factor for the formation of the trophectoderm, was downregulated by enforced expression of TPRX1. Overall, we suggest that TPRX1 is capable of modulating the expression of lineage-specific transcription factors in pluripotent cells derived from humans.
Introduction
In mice, the pluripotent inner cell mass, the precursor cells of all fetal and adult cell lineages, and the trophectoderm, precursor cells of placental cell lineages, arise during preimplantation development between the morula stage and the blastocyst stage. Embryonic stem (ES) cells [Citation1] and trophoblast stem cells [Citation2] are established from the inner cell mass and the trophectoderm, respectively. The primitive endoderm is generated from a portion of cells constituting the inner cell mass and gives rise to the extraembryonic endoderm cell lineage.
Transcription factors are expressed to coordinately regulate cell fates in the developing conceptus, such as preimplantation embryos. Recently, we identified the structurally related homeoproteins EGAM1 (GenBank accession No. AB472692, also known as CRXOS isoform a) and EGAM1N (No. AB472693, CRXOS isoform c) in preimplantation mouse embryos [Citation3]. These homeoproteins encoded by the Crxos gene (Gene ID 546024) on chromosome 7 () are expressed as splicing variants in both mouse embryos and mouse ES cells. EGAM1 and EGAM1N harbour homeodomains, such as the paired-like type (PRDL HD1) and the engrailed-like type (ENL HD) in EGAM1 and the paired-like type (PRDL HD1) in EGAM1N. When expressed exogenously in mouse ES cells, EGAM1 promoted differentiation into the primitive endoderm and inhibited differentiation towards the trophectoderm, which arise during the pre- and peri-implantation periods of early embryogenesis [Citation3,Citation4]. Conversely, EGAM1N supported the maintenance of an undifferentiated state [Citation3,Citation5]. EGAM1 homeoproteins were localized to the nuclei of mouse ES cells [Citation6], and homeodomains are known as a DNA-binding region [Citation7]; therefore, we postulated that EGAM1 homeoproteins act as DNA-binding transcription factors.
Figure 1. Relationship between the mouse Crxos gene and the human TPRX1 gene. (A) The flanking regions around mouse Crxos gene and human TPRX1 gene. ch., chromosome. (B) Schematic diagram of the primary amino acid sequence for EGAM1 homeoproteins and TPRX1 protein. The mouse EGAM1 homeoproteins are expressed as splicing variants. Boxes filled with the same patterns indicate the regions completely matching in each protein. Tetra-peptide repeat, the protein sequence contains 58 repeated copies of a four-amino acid motif (variants of Pro-Ile-Pro-Gly).
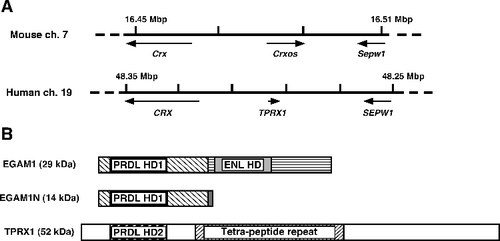
In humans, tetrapeptide-repeat homeobox 1 (TPRX1, Gene ID 284355) harbouring another paired-like type homeodomain (PRDL HD2) on chromosome 19 is a functionally unclassified gene [Citation8] (). Recently, Maeso et al. reported that ETCHbox (eutherian totipotent cell homeobox) genes, including TPRX1, ARGFX, LEUTX, DPRX and PARGFX, arose by tandem gene duplication from the retinally expressed CRX gene, followed by asymmetric sequence evolution in mammals [Citation9]. They also argued the Crxos gene is an ortholog of ETCHbox genes, especially of TPRX1 (), and suggested that ETCHbox genes are potentially involved in cell fate specification in preimplantation embryos [Citation9]. Indeed, TPRX1 is expressed in preimplantation embryos, testes and eyes in humans, with closely similar expression patterns to those of EGAM1 homeoproteins [Citation3,Citation8–11]. However, the roles of TPRX1 in gene expression in human pluripotent cells are still unknown. Against this background, in order to clarify the roles of orthologs for Crxos, we focused on the functions of TPRX1 in human embryonal carcinoma NT2/D1 cells, as a model of human pluripotent cells.
Materials and methods
Construction of the TPRX1 expression vector
A human TPRX1 cDNA (also known as CRX like homeobox 2, GenBank accession No. DQ340180 [Citation12]) was purchased from OriGene (Rockville, MD, USA). An expression vector for the TPRX1 cDNA was constructed using a dicistronic mRNA-expressing pMN1Pur vector [Citation3,Citation5,Citation13]. This vector contains a powerful and versatile CAG promoter [Citation14–16] and can express a single mRNA encoding a gene of interest, an internal ribosomal entry site (IRES) and a puromycin-resistance gene. Such a dicistronic vector ensures the co-expression of the desired gene in cells expressing the selectable marker gene. The cDNA corresponding to the coding sequence for TPRX1 in combination with DNA sequences encoding FLAG-tag at the 5’-end of the TPRX1 cDNA was inserted into the cloning site (XbaI/EcoRI) of the pMN1Pur vector.
Culture of human embryonal carcinoma NT2/D1 cells and transfection of TPRX1-expressing vector
NT2/D1 cells [Citation17,Citation18] (ECACC No. 01071221) were purchased from DS Pharma Biomedical (Osaka, Japan). The cells were routinely maintained with Dulbecco's modified Eagle's medium (4.5 g/L glucose, Sigma, St. Louis, MO, USA) supplemented with 2 mmol/L glutamine and 10% fetal calf serum (FCS, Biowest, Nuaille, France). NT2/D1 cells were transfected with the FLAG-tagged TPRX1-expressing vector by the lipofection method, and stable transfectants were obtained by cultivation with puromycin (0.25 μg/mL, Sigma), as reported previously [Citation13,Citation19]. Differentiation of NT2/D1 cells was induced by cultivation with medium containing retinoic acid (10−6 mol/L, all-trans type, Sigma).
RNA extraction and quantitative RT-PCR analysis
Extraction of total RNA, synthesis of cDNA, and quantitative reverse transcription polymerase chain reaction (qRT-PCR) were carried out in accordance with the methods reported previously [Citation13,Citation20,Citation21]. The set of gene-specific primers used are listed in . Hydroxymethylbilane synthase (HMBS) was analyzed as a housekeeping gene.
Table 1. Sequences of primers for quantitative reverse transcription polymerase chain reaction.
Western blotting
Cellular proteins separated by sodium dodecyl sulfate polyacrylamide gel electrophoresis (SDS-PAGE) followed by transfer onto a polyvinylidene fluoride (PVDF) membrane (Immobilon-P, Billerica, MA, USA) were incubated with a PVDF Blocking Reagent (Toyobo, Osaka, Japan). Subsequently, the membrane was incubated with primary antibodies: rabbit anti-FLAG (1:270,000, #600–401–383, Rockland Immunochemicals, Limerick, PA, USA) or rabbit anti-ACTB (also known as β-ACTIN, 1:20,000, #IMG-5142A, IMGENEX, San Diego, CA, USA). The membrane was incubated with horseradish peroxidase-conjugated anti-rabbit IgG (1:5,000, #AP132P, Chemicon, Temecula, CA, USA) as a secondary antibody. The enzyme reaction was detected using ECL prime Western Blotting Reagent (GE Healthcare Bio-Sciences, Piscataway, NJ, USA) and a LAS-4000 image analyzer (Fujifilm, Tokyo, Japan).
Immunofluorescence microscopy
Immunofluorescence microscopy was performed as reported previously [Citation6,Citation13]. In brief, primary (rabbit anti-FLAG IgG, 1:2,000, #F7425, Sigma) and secondary (fluorescein isothiocyanate (FITC)-labeled anti-rabbit IgG, 1:1,000, #A120–201F, BETHYL Laboratories, Montgomery, TX, USA) antibodies were diluted in 1% bovine serum albumin in phosphate-buffered saline. 4′,6-Diamidino-2-phenylindole (DAPI, 100 ng/mL) was added to the reaction mixture of the secondary antibody.
Statistical analysis
The results obtained by qRT-PCR analysis were subjected to one-way analysis of variance (ANOVA) followed by the Steel–Dwass test or the Tukey–Kramer multiple comparison test.
Results and discussion
Expression of TPRX1 mRNA in an undifferentiated state and during the progression of differentiation was undetectable in NT2/D1 cells
Morphological changes in NT2/D1 cells were evident between the undifferentiated state and during the stages of differentiation (A). As shown in B, the expression levels of mRNAs for pluripotency factors OCT4 and NANOG were downregulated during the progression of differentiation. On the other hand, the expression levels of the neuroectoderm marker ASCL1 (a transcription factor also known as MASH1) [Citation22,Citation23] were significantly upregulated (C, P < 0.05). The expression levels of the primitive endoderm marker GATA6 [Citation24,Citation25], encoding a transcription factor, were also upregulated, and those of the trophectoderm marker CDX2 [Citation26,Citation27], also encoding a transcription factor, were transiently upregulated during the progression of differentiation. The expression of endogenous TPRX1 mRNA was almost undetectable, whereas exogenous TPRX1 mRNA in NT2/D1 cells transfected with a TPRX1 expression vector (+FLAG/TPRX1) was clearly detected (D). These results suggested that the expression levels of TPRX1 were extremely low, and TPRX1 may be dispensable for the maintenance of an undifferentiated state and the progression of differentiation in NT2/D1 cells.
Figure 2. Changes in gene expression during the progression of differentiation in human embryonal carcinoma NT2/D1 cells. (A) Cell morphology of wild-type NT2/D1 cells in an undifferentiated state (Un) and after the induction of differentiation with 10−6 mol/L retinoic acid after a 14-day culture period (D14). Low, low cell density. High, confluent. Scale bar, 100 µm. (B) The expression levels of genes encoding pluripotency factors were quantified by qRT-PCR and normalized to that of HMBS, a housekeeping gene. Data are expressed as means ± SEM (n = 3 independent dishes) and the expression levels were compared with that of cells in an undifferentiated state (Un) at low cell density. *P < 0.05. (C) The expression levels of genes encoding lineage-specific transcription factors were quantified by qRT-PCR. Data were obtained and processed as described in (B). (D) The expression levels of TPRX1 were quantified by qRT-PCR. The expression levels of exogenous TPRX1 mRNA in NT2/D1 cells transfected with a TPRX1 expression vector (+FLAG/TPRX1) were regarded as a positive control. ASCL1 for neuroectoderm; GATA6 for primitive endoderm; CDX2 for trophectoderm.
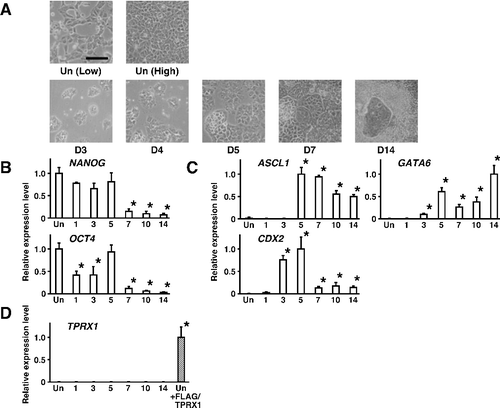
Effect of exogenous expression of TPRX1 protein on the induction of gene expression encoding lineage-specific transcription factors in NT2/D1 cells
Overexpression of specific genes or knockdown of gene expression are useful techniques for analyzing gene functions in cells. Although TPRX1 is expressed in preimplantation human embryos [Citation9,Citation10], TPRX1 transcripts were undetectable in an undifferentiated state and during the progression of differentiation in wild-type human embryonal carcinoma NT2/D1 cells. We considered that it would be advantageous to clarify the relationship between the exogenous expression of TPRX1 and the induction of genes encoding lineage-specific transcription factors in pluripotent cells as a model for preimplantation embryos.
As shown in A, overexpression of the FLAG-tagged TPRX1 protein was clearly shown in both an undifferentiated state and during the progression of differentiation in NT2/D1 cells (FLAG/TPRX1 transfected cells). In particular, the expression levels were very high after a 7-day culture period during the progression of differentiation. It is likely that the CAG promoter worked well during the progression of differentiation in NT2/D1 cells. FLAG-tagged TPRX1 protein localized specifically in the nuclei of transfectants (FLAG/TPRX1, B), suggesting that the protein probably plays a role in the nucleus. On the other hand, no marked differences in the cell morphology of FLAG/TPRX1 transfected cells were observed compared with those of the control transfectants (C).
Figure 3. Enforced expression of TPRX1 protein in NT2/D1 cells. (A) Expression of FLAG-tagged TPRX1 protein (Mr, 53 kDa) in NT2/D1 cells (FLAG/TPRX1) was analyzed by Western blotting. ACTB (also known as β-ACTIN; Mr, 42 kDa) is indicated as a loading control. The numbers over the bands indicate the culture period (days) after the induction of differentiation. NT2/D1 cells transfected with an empty vector (pMN1Pur as a control) are indicated as ‘Empty.’ Un, undifferentiated state. A vertical bar on the left side of the FLAG/TPRX1 panel indicates the position of non-specific signals. (B) Subcellular localization of FLAG-tagged TPRX1 protein in TPRX1 transfectants. FLAG-tagged TPRX1 was detected using fluorescein isothiocyanate (FITC)-labeled secondary antibodies. Cell nuclei were detected using 4′,6-diamidino-2-phenylindole (DAPI). Scale bar, 50 µm. (C) Cell morphology of NT2/D1 transfected cells in an undifferentiated state (Un) and following a 14-day culture period after the induction of differentiation (D14). Scale bar, 100 µm.
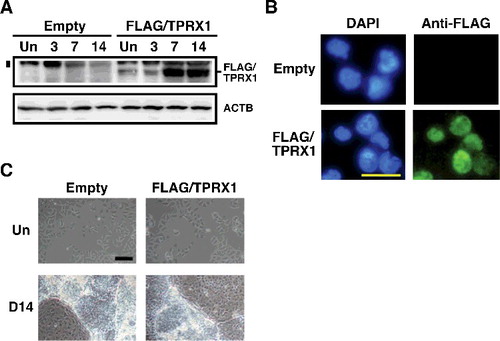
The induction of gene expression for crucial, lineage-specific transcription factors was analyzed (). The expression patterns of NANOG and OCT4 were similar in the control and FLAG/TPRX1 transfected cells before and after the induction of differentiation. During the progression of differentiation, the expression levels of GATA6, a master regulator for generation of the primitive endoderm, and those of FOXA2, a crucial transcription factor for the progression of differentiation into primitive endoderm [Citation28,Citation29], were upregulated in FLAG/TPRX1 transfected cells. Conversely, those of CDX2, a master regulator for formation of the trophectoderm, were downregulated. The expression levels of another crucial transcription factor, EOMES, for the progression of differentiation into the trophectoderm [Citation30,Citation31] were unchanged. The expression levels of ASCL1, a master regulator for the generation of the neuroectoderm, and T, a master regulator for the generation of the mesoderm [Citation32,Citation33], were almost unchanged. These results suggested that, in NT2/D1 cells, the enforced expression of TPRX1 protein directed differentiation into the primitive endoderm together with partially inhibiting differentiation into the trophectoderm. Notably, this effect of the TPRX1 gene closely corresponded to that of EGAM1 protein encoded by the Crxos gene in mouse ES cells [Citation3,Citation4]. Our findings support, at least in part, the possibility that TPRX1 plays a role in the specification of cell fate in preimplantation human embryos, as reported previously [Citation9,Citation10].
Figure 4. Changes in the expression levels of genes encoding crucial transcription factors by enforced expression of TPRX1 protein. (A) The expression levels of genes encoding pluripotency factors were quantified by qRT-PCR analysis. Data represent the sum of two independent transfection experiments, because the results obtained from trials matched well, and are expressed as means ± SEM (n = 6 independent dishes). *P < 0.05. (B) qRT-PCR analysis of lineage-specific transcription factors (n = 6 independent dishes). *P < 0.05. ASCL1 for neuroectoderm; T for mesoderm; GATA6 and FOXA2 for primitive endoderm; CDX2 and EOMES for trophectoderm.
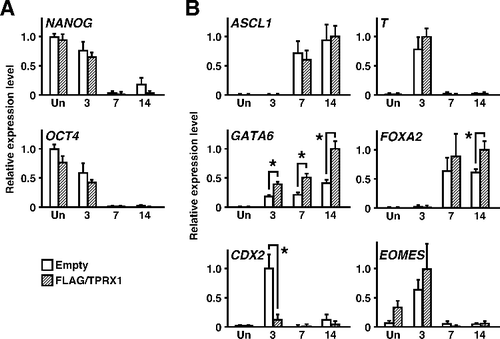
Conclusions
From this study, we suggest that TPRX1 is capable of modulating the expression of lineage-specific transcription factors in human pluripotent cells. Our results also suggested that human TPRX1 is not only the evolutionary ortholog of mouse Crxos, but also there are functional similarities between TPRX1 and Crxos in pluripotent cells.
Disclosure statement
The authors declare that we have no conflict of interest.
Additional information
Funding
References
- Evans MJ, Kaufman MH. Establishment in culture of pluripotential cells from mouse embryos. Nature. 1981;292(5819):154–156.
- Tanaka S, Kunath T, Hadjantonakis A, et al. Promotion of trophoblast stem cell proliferation by FGF4. Science. 1998;282(5396):2072–2075.
- Saito K, Abe H, Nakazawa M, et al. Cloning of complementary DNAs encoding structurally related homeoproteins from preimplantation mouse embryos: their involvement in the differentiation of embryonic stem cells. Biol Reprod. 2010;82(4):687–697.
- Soma M, Iha M, Kihara Y, et al. Preferential emergence of cell types expressing markers for primitive endoderm lineages in mouse embryonic stem cells expressing exogenous EGAM1 homeoprotein. J Biosci Bioeng. 2012;114(3):342–346.
- Sato S, Nakazawa M, Kihara Y, et al. Partial inhibition of differentiation associated with elevated protein levels of pluripotency factors in mouse embryonic stem cells expressing exogenous EGAM1N homeoprotein. J Biosci Bioeng. 2015;120(5):562–569.
- Sato S, Morita S, Iha M, et al. Intact structure of EGAM1 homeoproteins and basic amino acid residues in the common homeodomain of EGAM1 and EGAM1C contribute to their nuclear localization in mouse embryonic stem cells. J Biosci Bioeng. 2013;116(2):141–146.
- Prochiantz A, Di Nardo AA. Homeoprotein signaling in the developing and adult nervous system. Neuron. 2015;85(5):911–925.
- Booth HA, Holland PW. Annotation, nomenclature and evolution of four novel homeobox genes expressed in the human germ line. Gene. 2007;387(1–2):7–14.
- Maeso I, Dunwell TL, Wyatt CD, et al. Evolutionary origin and functional divergence of totipotent cell homeobox genes in eutherian mammals. BMC Biol. 2016 [cited 2017 Sep 05];14(1):45. DOI: 10.1186/s12915-016-0267-0.
- Madissoon E, Jouhilahti EM, Vesterlund L, et al. Characterization and target genes of nine human PRD-like homeobox domain genes expressed exclusively in early embryos. Sci Rep. 2016 [cited 2017 Sep 05];6:28995. DOI: 10.1038/srep32053.
- Saito K, Abe H, Nakazawa M, et al. Ontogenic expression patterns of transcripts encoding EGAM1 homeoproteins during murine organogenesis. Biotechnol Biotec Eq. 2012;26(5):3321–3323.
- Zhong YF, Holland PW. The dynamics of vertebrate homeobox gene evolution: gain and loss of genes in mouse and human lineages. BMC Evol Biol. 2011 [cited 2017 Sep 05];11:169. DOI: 10.1186/1471-2148-11-169.
- Iha M, Watanabe M, Kihara Y, et al. Effect of ectopic expression of homeoprotein EGAM1C on the cell morphology, growth, and differentiation in a mouse embryonic stem cell line, MG1.19 cells. Reproduction. 2012;143(4):477–489.
- Niwa H, Yamamura K, Miyazaki J. Efficient selection for high-expression transfectants with a novel eukaryotic vector. Gene. 1991;108:193–200.
- Kobayashi M, Yamauchi Y, Tanaka A, et al. Improved dicistronic mRNA expression vectors for efficient selection of transfectants highly expressing foreign genes. Biotechniques. 1996;21(3):398–402.
- Abe H, Nakazawa M, Kasuga K, et al. The CAG promoter is more active than the CEF promoter for the expression of transgenes in a mouse ES cell line E14-derived EB3 cells. Biotechnol Biotecnol Equip. 2011;25:2301–2304.
- Lee VM, Andrews PW. Differentiation of NTERA-2 clonal human embryonal carcinoma cells into neurons involves the induction of all three neurofilament proteins. J Neurosci. 1986;6(2):514–521.
- Mancino M, Strizzi L, Wechselberger C, et al. Regulation of human Cripto-1 gene expression by TGF-beta1 and BMP-4 in embryonal and colon cancer cells. J Cell Physiol. 2008;215(1):192–203.
- Kobayashi M, Yamauchi Y, Tanaka A. Stable expression of antisense Rb-1 RNA inhibits terminal differentiation of mouse myoblast C2 cells. Exp Cell Res. 1998;239(1):40–49.
- Soma M, Iha M, Sato S, et al. Factors affecting the expression of differentiation marker genes for the primitive endoderm lineage in a mouse extra-embryonic endoderm stem cell line, XEN26 cells. Biotechnol Biotecnol Equip. 2011;25(4):2679–2682.
- Saito K, Ogawa A, Toyofuku K, et al. Relationships between homeoprotein EGAM1C and the expression of the placental prolactin gene family in mouse placentae and trophoblast stem cells. Reproduction. 2011;141(2):259–268.
- Memic F, Knoflach V, Sadler R, et al. Ascl1 Is Required for the Development of Specific Neuronal Subtypes in the Enteric Nervous System. J Neurosci. 2016;36(15):4339–4350.
- Ball DW, Azzoli CG, Baylin SB, et al. Identification of a human achaete-scute homolog highly expressed in neuroendocrine tumors. Proc Natl Acad Sci U S A. 1993;90(12):5648–5652.
- Morrisey EE, Tang Z, Sigrist K, et al. GATA6 regulates HNF4 and is required for differentiation of visceral endoderm in the mouse embryo. Genes Dev. 1998;12(22):3579–3590.
- Schrode N, Saiz N, Di Talia S, et al. GATA6 levels modulate primitive endoderm cell fate choice and timing in the mouse blastocyst. Dev Cell. 2014;29(4):454–467.
- Strumpf D, Mao CA, Yamanaka Y, et al. Cdx2 is required for correct cell fate specification and differentiation of trophectoderm in the mouse blastocyst. Development. 2005;132(9):2093–2102.
- Niwa H, Toyooka Y, Shimosato D, et al. Interaction between Oct3/4 and Cdx2 determines trophectoderm differentiation. Cell. 2005;123(5):917–929.
- Hwang JT, Kelly GM. GATA6 and FOXA2 regulate Wnt6 expression during extraembryonic endoderm formation. Stem Cells Dev. 2012;21(17):3220–3232.
- Dufort D, Schwartz L, Harpal K, et al. The transcription factor HNF3beta is required in visceral endoderm for normal primitive streak morphogenesis. Development. 1998;125(16):3015–3025.
- Russ A, Wattler S, Colledge W, et al. Eomesodermin is required for mouse trophoblast development and mesoderm formation. Nature. 2000;404:95–99.
- Kwon GS, Hadjantonakis AK. Eomes::GFP-a tool for live imaging cells of the trophoblast, primitive streak, and telencephalon in the mouse embryo. Genesis. 2007;45(4):208–217.
- Herrmann BG, Labeit S, Poustka A, et al. Cloning of the T gene required in mesoderm formation in the mouse. Nature. 1990;343(6259):617–622.
- Wilson V, Rashbass P, Beddington RS. Chimeric analysis of T (Brachyury) gene function. Development. 1993;117(4):1321–1331.