Abstract
The continent of Antarctica, as a combination of constantly low temperatures, strong winds, short summer season, and high solar radiation, is a highly extreme habitat suggesting appropriate conditions for growth of psychrophilic microorganisms. Five psychrophilic yeast strains were isolated from the samples taken from the region of the Bulgarian Base on Livingston Island, Antarctica: Cryptococcus laurentii AL65, Sporobolomyces salmonicolor AL36, Debaryomyces hansenii, Leucosporidium scotii and Rhodotorula glutinis, and their biomass yield and exopolysaccharides production were investigated. Best growth was observed for L. scotii and C. laurentii AL65, with 7.5 and 6.0 g/L biomass, respectively, and highest exopolysaccharide yield was established for L. scotii. Metabolic profiling revealed phylogenetically based diversity in the identified metabolic profiles. The proton nuclear magnetic resonance (1H NMR) spectroscopy analyses of compounds extracted from the biomass of the strains revealed significant differences in the metabolites between individual yeast strains in our investigation including: among the amino acids alanine, valine, threonine, leucine and tyrosine; some organic acids such as gamma-aminobutyric acid, acetic acid, formic acid and others. Glucose was identified in all investigated strains. The highest diversity of compounds was observed in D. hansenii strain, division Ascomycota. The main compounds in the metabolic profile of Basidiomycota strains were sugars. The statistical analysis revealed significant differences in the studied metabolites among the yeast strains. This result suggests that, together with 16S rRNA gene and enzyme gene analyses, metabolite profiling could be also used as a marker for a phylogenetic distance in fungi evolution.
Keywords:
Introduction
The investigations on Antarctic yeasts were initiated in the 1960s with the description of their biodiversity and investigations on their adaptive properties [Citation1]. Antarctic yeasts are a valuable bio-resource to generate novel bioactive molecules and develop new microbial biotechnological processes. The advantages of these processes are the controlability of the conditions and their independence of the geographical location, climate characteristics of the region, etc., as well as the relatively short fermentation time and the low value of the substrates used. A particular advantage of these processes is the natural protection against contamination, due to the pH decrease in the culture medium and its protection against contaminating agents.
The industrial demand for non-pathogenic and biotechnologically unexplored microorganisms in recent decades motivates scientists to study the inhabitants of various extreme habitats, such as the ice-covered continent of Antarctica [Citation2, Citation3], etc. There are undoubted benefits from the usage of microorganisms with rapid metabolism at low temperature and exopolysaccharides (EPSs) biosynthesized by psychrophilic yeasts have received biotechnological recognition because of their unique structures and functional properties [Citation4, Citation5]. As a result of long-standing studies, new exopolysaccharides from psychrophilic yeasts have been isolated and their good emulsifying, foaming and stabilizing properties have determined their inclusion in experimental cosmetic formulations: day cream, night cream and toilet milk. The results have shown better emulsifying properties than synthetic emulsifiers used in cosmetic products as a standard [Citation5–8]. However, the information about the potential use of biologically active substances synthesized by psychrophilic yeasts in medicine and pharmacy is still scarce.
Psychrophilic microorganisms can survive at the unfavourable environmental conditions by synthesizing unique substances with proven biological value: exopolysaccharides, glycerol, trehalose in the cell, anti-freeze proteins, and a high ratio of unsaturated fatty acids in the membrane lipids, β-carotene, coenzyme Q10, ergosterol, etc. [Citation9–11]. The synthesis of cryoprotective molecules is a part of the mechanisms to withstand low temperatures [Citation12]. Although significant knowledge on the mechanisms of adaptation to cold stress in bacteria has been accumulated, the knowledge of the survival mechanisms of yeast in almost freezing temperatures is scarce. The adaptation mechanisms to temperature stress have been studied in Saccharomyces cerevisiae [Citation13]. A domination of Basidiomycetes in Antarctica has been reported [Citation14], and cold stress responses in the Antarctic basidiomycetous yeast Mrakia blollopis have been investigated using capillary electrophoresis–time-of-flight mass spectrometry [Citation15]. However, to the best of our knowledge, there is still no information about the mechanisms of low temperature adaptation in representatives of class Microbotryomycetes.
Various valuable compounds are synthesized by the genera Leucosporidium, Rhodotorula and Sporobolomyces belonging to class Microbotryomycetes. Synthesis of pigments, oils, carotenoids such as β-carotene, torulene and torularhodin and enzymes has been reported for Rhodotorula glutinis [Citation16–19]. The psychrophilic strain Sporobolomyces salmonicolor AL1 has been reported as a producer of antioxidant substances (ergosterol, β-carotene, coenzyme Q10, torulene and torularhodin) and extracellular glucomannan with cosmetic application [Citation9, Citation20, Citation21]. Biotechnological research of Leucosporidium antarcticum has shown the production of proteinase, α-glucosidase, lipase, acidic and alkaline phosphatase [Citation22]. The exopolysaccharide mannan has been produced by R. rubra 14 and R. acheniorum MC [Citation23, Citation24]. Isoprenoid compounds with antioxidant activity, such as ergosterol, coenzyme Q10 and β–carotene, have been confirmed in the biomass of psychrophilic yeasts S. salmonicolor AL1, C. albidus AS55, Cryptococcus laurentii AS56 and C. laurentii AS58. High amounts of tocopherols are produced by C. albidus AL55 and C. laurentii AL56 [Citation9].
Metabolomics reveals the unique chemical fingerprints that specific cellular processes leave behind [Citation25] and can be used for faster identification of low molecular weight metabolites of organisms and study of their corresponding properties for molecules of interest. Different approaches like gas chromatography–mass spectrometry (GC-MS), liquid chromatography LC/MS and capillary electrophoresis CE/MS have been explored. GC/MS-based metabolomics has contributed the development of a data-mining system for metabolite identification using soft independent modelling of class analogy [Citation26, Citation27] and has been used extensively in analytical practice for qualitative and quantitative identification of small molecules [Citation25, Citation28, Citation29]. However, proton nuclear magnetic resonance (1H NMR) spectroscopy gives reproducible results, a possibility for registration of many peaks and their easy identification.
This work was motivated by the lack of information on low molecular weight metabolites for the Antarctic yeasts from class Microbotryomycetes. The aim was to characterise the biologically active substances synthesized by representatives of Antarctic yeasts by 1H NMR-based metabolic profiling.
Materials and methods
Microorganisms
The strains were isolated from the region of the Bulgarian Base on Livingston Island, Antarctica, and identified as belonging to two divisions: C. laurentii AL65, S. salmonicolor AL36, Leucosporidium scotii and R. glutinis 8337 were identified as belonging to the division Basidiomycota and Debaryomyces hansenii to Ascomycota [Citation30, Citation31]. They were stored in the Antarctic yeast collection of the Laboratory of Applied Biotechnology, the Stephan Angeloff Institute of Microbiology-BAS.
Culture media and cultivation conditions
The fermentation medium contained: sucrose: 40 g/L; (NH4)2SO4: 2.5 g/L; KH2PO4: 1.0 g/L; MgSO4.7H2O: 0.5 g/L; NaCl: 0.1 g/L; CaCl2.2H2O: 0.1 g/L; yeast extract (Sigma-Aldrich)[AQ2]: 1.0 g/L. The initial pH was adjusted to pH 5.3 ± 0.3, and the medium was sterilized for 30 min at 70.93 kPa. The inoculum from each psychrophilic strain was obtained on a rotary shaker at 220 rpm, 22 °C for 48 h. The inocula were prepared in 500 mL flasks containing 50 mL of the medium. The fermentation medium was inoculated with 10.0% w/v inoculum. Submerged cultivation was performed in 500 mL flasks containing 50 mL of the medium, at 22 °C for 120 h with shaking at 220 rpm.
Analytical methods
The biomass was separated by centrifugation at 5000 g for 30 min. The dry biomass was determined after drying at 105 °C to constant mass. The biomass gathered by the submerged and surface cultivation was washed out and lyophilized (Alpha 1-2, Christ, Germany). The exopolysaccharides were isolated from the supernatant by precipitation with cold ethanol. The total carbohydrate content of the culture supernatant was determined by the method of Dubois et al. [Citation32]. The results in the figures are presented as mean values from three experiments with standard deviation (±SD).
Standards and chemicals
CH3OH-d4 (99.8%) (Deutero GmbH, Germany), D2O (99.9%) (Deutero GmbH, Germany) and trimethylsilylpropionic acid sodium salt-d4 (TMSP-d4) (Sigma-Aldrich).
Extraction and NMR analyses
Freeze-dried biomass samples (100 mg) were homogenized with equal amounts of CD3OD (0.75 mL) and D2O (KH2PO4 buffer, pH 6.0), containing 0.005% (w/v) TSPA-d4 (0.75 mL). After 40 min of ultrasonication (35 kHz; UCI-50Raypa® R. Espinar S.L., Barcelona, Spain) samples were centrifuged (14,000 g, 20 min) and the supernatant was transferred to an NMR tube (5 mm) for further spectral analysis as described by Kim et al. [Citation12].
Proton (1H) as well as two-dimensional (2D) NMR spectra (J-resolved, 1H-1H correlated spectroscopy (1H-1H COSY) and heteronuclear single quantum correlation (HSQC)) were recorded at 25 °C on an AVII+ 600 spectrometer (Bruker, Karlsruhe, Germany), operating at a proton NMR frequency of 600.11 MHz [Citation33]. For internal lock, deuterated methanol was used and the relaxation time was 4.07 s. The resulting 1H NMR spectra were further processed by referencing to the internal standard trimethylsilylpropanoic acid (TSPA), phased and baseline corrected, by running MestReNova software (v10.0, Mestrelab Research, Santiago de Compostela, Spain).
Multivariate data analysis
Normalization to a constant sum (reference sample normalization for binned spectra) and the Pareto scaling method were used to prepare the input for principal component analysis (PCA) and hierarchical cluster analysis (HCA). For multivariate data analyses, the 1D 1H NMR spectra were preprocessed by ACDLABS 12.0/NMR Processor Academic Edition. The spectra were handled in two ways. In the first one, all 20 spectra were simultaneously integrated in variable size intervals by ACD intelligent bucketing routine in the region between δ 0.5 and 5.5 ppm. The data reduction resulted in 130 bins of size 0.04 ppm at 50% width looseness. In the second one, the spectral peak lists at a threshold signal-to-noise ratio of 10 were generated. A total of 135 peaks were formed after automatic summing and alignment of moving window grouped signals. Residual solvent signals of CH3OH-d4 in the range of 3.25–3.33 ppm and D2O 4.77–4.83 ppm were removed before statistical analysis. MetaboAnalyst 3.0 for metabolomics data was used for pattern recognition.
Results and discussion
Among the investigated yeast strains, C. laurentii AL65, S. salmonicolor AL36, D. hansenii, L. scotii and R. glutinis 8337, showed accumulation of different amounts of biomass in deep culture, ranging from 2.8 to 7.5 g/L ().
The biomass yield was the highest for the strains L. scotii (around 7.5 g/L) and C. laurentii AL65 (6.0 g/L) at 120 h of deep cultivation, which is comparable with that previously reported for C. laurentii AL100 and C. laurentii AL62 [Citation5, Citation8].
EPS synthesis was growth-associated and reached maximal levels at the beginning of the stationary phase. Highest EPS yield was observed for L. scotii (0.48 g/L), other strains produced EPS in the range of 0.039–0.339 g/L ().
The pH value decreased during the fermentation process reaching 2.0–2.4 in the supernatant of each culture and was a specific characteristic different adaptation strategies to Antarctic conditions according to the phylogenetic position. for the process of extracellular biopolymer synthesis [Citation5, Citation8]. This low pH value is of important biotechnological significance hindering microbial contamination of the processes. The type and monosaccharide composition of the EPS synthesized by the Antarctic strains isolated by us were described in details in our previous research [Citation5, Citation8]. In this study, we made an attempt to connect the EPS synthesis as an adaptive mechanism towards extreme Antarctic conditions with other adaptive metabolic features revealed by metabolic profiling.
The results from the metabolite profiling of the investigated strains proved the presence of different metabolites in the yeast cells probably connected with different adaptation strategies to Antarctic conditions according to the phylogenetic position. The highest variety of compounds, including amino acids, sugars and organic acids, were identified in the profile of D. hansenii, division Ascomycota (). The strong sugar domination observed in the profile of all Basidiomycota representatives suggests an active participation of sugar metabolism in the yeast from this division, opposed to the variety of amino acids in the cells of the Ascomycota representatives ( and ). The comparison of the metabolite profiles of M. blollopis [Citation15] and C. laurentii (current investigation), both belonging to class Tremellomycetes, revealed that organic acids and aromatic amino acids were not identified in either of these two strains. The high level of EPS synthesis and identified glucose molecules in the metabolic profiles of the two representatives of class Microbotryomycetes, L. scotii and S. salmonicolor, suggests that they have developed EPS synthesis as a protective mechanism. Sequencing of the complete genomes made it possible to determine the phylogenetic relationships between metabolic genes in various organisms and their comparison confirmed the phylogenetic tree constructed on the basis of 16S rRNA [Citation34]. The obtained results suggest that metabolite profiles should also be used for the study of the evolutional history of microbial life.
Figure 2. 1H NMR spectra of extracted metabolites. D. hansenii (1); L. scotii (2); C. laurentii (3); S. salmonicolor (4); and R. glutinis (5).
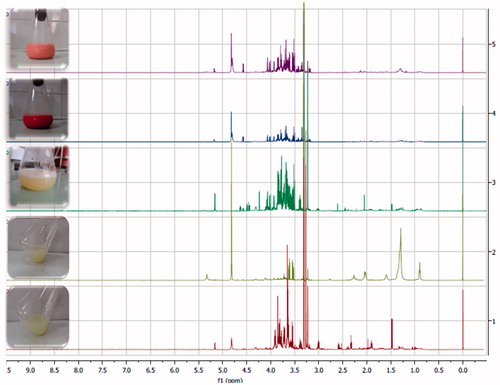
Table 1. 1H NMR chemical shifts (δ, ppm) and coupling constant (J, Hz) identified by relevant 1H NMR and 2D NMR spectra.
The metabolic profiles of the strains belonging to class Microbotryomycetes, S. salmonicolor AL36, L. scoti and R. glutinis showed strong sugar domination, suggesting an active participation of sugar metabolism in the growth of the yeasts from this class at Antarctic conditions. A variety among the sugars was also observed for the representatives of another two classes of Basidiomycota, Saccharomycetes (D. hansenii) and Tremellomycetes (C. laurentii), some of the amino acids were not identified. Choline was presented in the cells of all investigated strains. The GABA was identified only in D. hansenii ().
Our further work will focus on the metabolic pathways for biotechnologically important metabolites like alanine, leucine and/or tyrosine. Some of the amino acids presented in the cells, like alanine, leucine, valine and threonine, are indispensable and are used for medical treatment. Tyrosine is also considered to be involved in signal transduction and regulation of enzyme activity in organisms [Citation35]. GABA is a metabolite known as a mediator-relaxant in the human brain and is involved in brain-related treatments [Citation36, Citation37]. Much hope is laid on GABA-based rugs as the next wave in the therapy of central nervous system disorders [Citation37].
The fermentation processes exploring psychrophilic yeasts have several advantages like low-cost fermentation medium and cultivation conditions appropriate for large-scale processes. Additionally, the decrease of pH to 2.0 during the fermentation process prevents microbial contamination. The resulting glucomannan, arabinomannan and xylomannan synthesized by the Antarctic yeasts S. salmonicolor AL1, C. laurentii AL100 and C. laurentii AL62 have found application as emulsifiers in model emulsions [Citation5, Citation6, Citation21]. The approach for fast characterization of the synthesized metabolites and the possibility for direction of the synthesis towards desired targets are of great impact for present- day biotechnological processes.
The two unsupervised pattern-recognition methods, PCA and HCA, in this study were used to evaluate the difference between yeast strains of interest regarding the intrinsic variation within each taxonomic group. The pattern of spectral peaks ( and ) was similar to that of bins, except for the more compact clusters formed. The scores plot of the first and the second principal component and the dendrogram both revealed that the five clusters were clearly separated from each other.
The class distribution pattern of the two red strains, S. salmonicolor and R. glutinis ( and ), was the closest in regard to their spectral features but relatively distant from the other three groups, which were also remote themselves.
Figure 4. HCA Dendograms of 20 spectral peak tables. Note: Euclidean distance and minimum variance linkage method (Ward’s) are used.
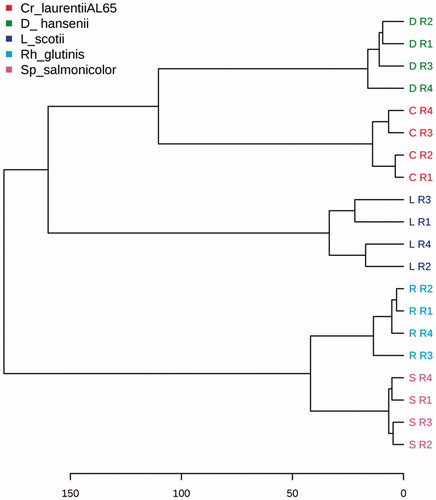
Additionally, projection and orthogonal projections to latent structures discriminant analysis (PLS/O-PLS-DA) were applied to validate the model and to facilitate the interpretation of the results. The performance parameters of R2 = 0.991 and Q2 = 0.983 for the 3-component PLS confirmed the robustness of the model. The large difference between R2X 0.198 and R2Y 0.941 (Q2 = 0.915) for p1 O-PLS revealed that only a small percentage of the sample variation explains nearly the whole class variation on Y.
Significant regions were identified based on S-plot and variable importance in projection (VIP). The most important signals contributing to class distribution and corresponding to potential characteristic metabolites comprised mainly the range of 3.2–3.8 ppm of monosaccharides and sugar alcohols, also near 4.6 and 5.2 ppm and some chemical shifts about 1.5 and 3 ppm of certain amino acids like alanine and gammaaminobutyric acid (GABA) ().
In the present work, an overview of the overall metabolic profiles of phylogenetically different yeasts was made using NMR spectroscopy, and the new data were combined with chemometric surveys of the corresponding yeast species. These spectral data can be successfully explored as a reference point for directing biotech processes towards a desired target product, and could be used to overcome some difficulties in fungal taxonomy.
Conclusions
Antarctic yeasts are a valuable resource for obtaining biologically active substances. The amounts of accumulated biomass and extracellular polysaccharide demonstrate possibilities of scaling-up the processes in a fully controlled environment. Regardless of the climate conditions of the environment, the economically viable nutrient medium, the relatively short cultivation time and the low pH are indisputable advantages for the production of target metabolites. Metabolic profiling, applied for the first time in characterization of Antarctic yeasts, revealed phylogenetically based diversity in identified metabolic profiles. The statistical analysis of the 1D spectra showed that the five studied strains differ significantly in regard to their metabolic composition; however, some similarity connected with their phylogenetic position was observed. The methods for metabolic profiling based on NMR allowed the identification of intracellular metabolites such as alanine, valine, leucine, GABA and some organic acids. Some of these, along with extracellular polysaccharides, can be used in the practice as part of waste-free and more cost-effective technologies.
Acknowledgements
Authors are thankful to Assoc. Prof. Dr. Kostantza Pavlova for kindly supplying of the Antarctic yeast strains.
Disclosure statement
No potential conflict of interest was reported by the authors.
Additional information
Funding
References
- Connell LB, Rodriguez RR, Redman RS, et al. Cold adapted yeasts in Antarctic desserts. In: Buzzini P, Margesin R, editors. Cold-adapted yeasts: biodiversity, adaptation strategies and biotechnological significance. Springer: Berlin, Heidelberg; 2013. p. 75–98.
- Gerday C, Aittaleb M, Bentahir M, et al. Cold-adapted enzymes: from fundamentals to biotechnology. Trends Biotechnol. 2000;18:103–107.
- Vishniac H. Yeast biodiversity in the Antarctic. In: Rosa C, Péter G, editors. Biodiversity and ecophysiology of yeasts. Springer: Berlin, Heidelberg; 2006. p. 419–439.
- Margesin R, Schinner F. Effect of temperature on oil degradation by a psychrophilic yeast in liquid culture and in soil. FEMS Microb Ecol. 1997;24:243–249.
- Pavlova K, Rusinova-Videva S, Kuncheva M, et al. Synthesis and characterization of an exopolysaccharide from Antarctic yeast strain Cryptococcus laurentii AL100. Appl Biochem Biotech. 2011;163:1038–1052.
- Kuncheva M, Panchev I, Pavlova K, et al. Functional characteristics of an exopolysaccharide from Antarctic yeast strain Cryptococcus laurentii AL62. Biotechnol Biotechnol Equip. 2013;27(5):4098–4102.
- Pavlova K, Dobreva S, Panchev I, et al. Cosmetic application of arabinomannan and xylomannan produced by Antarctic yeast strains. Sci Works Univ Food Technol. 2012;59:330–333.
- Rusinova-Videva S, Pavlova K, Georgieva K. Effect of different carbon sources on biosynthesis of exopolysaccharide from Antarctic strain Cryptococcus laurentii AL62. Biotech Biotechnol Equip. 2011;25:80–84.
- Dimitrova S, Pavlova K, Lukanov L, et al. Synthesis of coenzyme Q10 and beta-carotene by yeasts isolated from Antarctic soil and lichen in response to ultraviolet and visible radiations. Appl Biochem Biotechnol. 2010;162(3):795–804.
- Zlatanov M, Pavlova K, Antova G, et al. Biomass production by Antarctic yeast strains: an investigation on the lipid composition. Biotech Biotechnol Equip. 2010;24:2096–2101.
- Rusinova-Videva S, Dimitrova S, Georgieva K, et al. Effect of Zn2+, Cu2+ and Fe2+ ions for accumulation of ergosterol, β–carotene and coenzyme Q10 by Antarctic yeast strain Sporobolomyces salmonicolor AL1. Compt rend de l’Acad Bul Sci. 2016;69(8):1003–1010.
- Nicolaus B, Kambourova M, Oner E. Exopolysaccharides from extremophiles: from fundamentals to biotechnology. Environ Technol. 2010;31:1145–1158.
- Aguilera J, Randez-Gil F, Prieto JA. Cold response in Saccharomyces cerevisiae: new functions for old mechanisms. FEMS Microbiol Rev. 2007;31:327–341.
- Tsuji M, Fujiu S, Xiao N, et al. Cold adaptation of fungi obtained from soil and lake sediment in the Skarvsnes ice-free area, Antarctica. FEMS Microbiol Lett. 2013;346:121–130.
- Tsuji M. Cold-stress responses in the Antarctic basidiomycetous yeast Mrakia blollopis. Royal Society Open Sci. 2017;3:1–13.
- Kot A, Blazejak S, Kurez A, et al. Rhodotorula glutinis – potential of lipids, carotenoids, and enzymes for use in industries. Appl Microbiol Biotechnol. 2016;100:6103–6117.
- Hernandez-Almanza A, Montanez J, Aguilar-Gonzalez M, et al. Rhodotorula glutinis as source of pigments and metabolites for food industry. Food Biosci. 2014;5:64–72.
- Petrik S, Marova I, Haronikova A, et al. Production of biomass, carotenoid and other lipid metabolites by several red yeast strains cultivated on waste glycerol from biofuel production-a comparative screening study. Annal Microbiol. 2013;63(4):1537–1551.
- Marova I, Carnecka M, Halienova A, et al. Use of several waste substrates for carotenoid-rich yeast biomass production. J Env Manage. 2012;95:S338–S342.
- Dimitrova S, Pavlova K, Lukanov L, et al. Production of metabolites with antioxidant and emulsifying properties by Antarctic strain Sporobolomyces salmonicolor AL1. Appl Biochem Biotech. 2013;169:301–311.
- Kuncheva M, Pavlova K, Panchev I, et al. Emulsifying power of mannan and glucomannan produced by yeasts. Int J Cosmet Sci. 2007;29:377–384.
- Turkiewicz M, Pazgier M, Kalinowska H, et al. A cold-adapted extracellular serine proteinase of the yeast Leucosporidium antarcticum. Extremophiles. 2003;7:435–442.
- Elinov N, Vitovskaya G, Marikhin V, et al. Mannan produced by Rhodoturula rubra strain 14. Carbohydr Res. 1979;75:185–190.
- Grigorova D, Pavlova K, Panchev I. Preparation and preliminary characterization of exopolysaccharides by yeast Rhodotorula acheniorum MC. Appl Biochem Biotech. 1999;81:181–191.
- Chae YK, Kim SH, Ellinger JE, et al. Dosage effects of salt and pH stresses on Saccharomyces cerevisiae as monitored via metabolites by using two dimensional NMR spectroscopy. Bull Korean Chem Soc. 2013;34(12):3602–3608.
- Tsugawa H, Tsujimoto Y, Arita M, et al. GC/MS based metabolomics: development of data mining system for metabolite identification by using soft independent modeling of class analog (SIMCA). BMC Bioinformat. 2011;12:131.
- Theodoridis G, Gika H G, Wilson ID. Mass spectrometry-based holistic analytical approaches for metabolite profiling in systems biology studies. Mass Spec Rev. 2011;30(5):884–906.
- Lewis IA, Schommer SC, Hodis B, et al. Method for determining molar concentrations of metabolites in complex solutions from two-dimensional 1H-13C NMR spectra. Anal Chem. 2007;79:9385–9390.
- Lourenço AB, Ascenso JR, Sá-Correia I. Metabolic insights into the yeast response to propionic acid based on high resolution 1H NMR spectroscopy. Metabol. 2011;7:457–468.
- Pavlova K, Gushterova A, Savova I, et al. Isolation and taxonomic study of Antarctic yeast from Livingston Island for exopolysaccharide-producing strains. Bul Ant Res Life Sci. 2004;4:27–34.
- Pavlova K, Mecheva R, Savova I, et al. Study of yeast isolated from penguin plumage from western Antarctica. Bull Ant Res Life Sci. 2006;5:51–62.
- Dubois M, Gilles K, Hamilton J, et al. Colorimetric methods for determination of sugars and related substances. Anal Chem. 1956;28:350–356.
- Georgiev MI, Radziszewska A, Neumann M, et al. Metabolic alterations of Verbascum nigrum L. Plants and SAArT transformed roots as revealed by NMR-based metabolomics. Plant Cell Tiss Organ Cult. 2015;123:349–356.
- Oren A. Systematics of Archaea and Bacteria. In: Minelli A, Contrafatto G, editors. Biological science: fundamentals and systematics. EOLSS Publishers: Paris; 2009. p. 244–269.
- Mahoney C, Castellani J, Kramer F, et al. Tyrosine supplementation mitigates working memory decrements during cold exposure. Physiol Behav. 2007;92:575–582.
- Chapouthier G, Venault P. A pharmacological link between epilepsy and anxiety? Trends Pharmacol Sci. 2001;22(10):491–493.
- Foster A, Kemp J. Glutamate- and GABA-based drugs: the next wave of CNS therapeutics. Curr Opin Pharm. 2006;6:5–6.