Abstract
Although SH3GLB1 is well known as an autophagy-related protein, its response mechanism to virus infection has yet to be determined. Through digital gene expression (DGE) profile analysis, we identified that SH3GLB1 may be a key gene responding to classical swine fever virus (CSFV) infection in porcine alveolar macrophages (PAMs). The present study found that the mRNA expression and the SH3GLB1 protein level in CSFV Shimen infected PAMs were significantly higher than those of mock-infected PAMs. Furthermore, confocal microscopy analysis suggested that SH3GLB1 was temporally co-localised with CSFV E2. These findings contributed in developing our understanding of the mechanism of CSFV infection.
Keywords:
Introduction
SH3GLB1, also known as Bax-interacting factor 1 (Bif-1), is a member of the endophilin B gene family [Citation1,Citation2]. SH3GLB1 was first discovered as a Bax-interacting gene and encodes a protein that incorporates a carboxyl terminal SH3 domain and an amino-terminal N-BAR domain [Citation1–5]. As a membrane curvature-inducing protein, SH3GLB1 can interact with an autophagy protein, Beclin1, with the help of UVRAG (ultraviolet irradiation resistance-associated gene) and plays a role as an activator of class III phosphatidylinositol 3-kinasecomplex II (PIK3C3CII) [Citation4–8]. Furthermore, SH3GLB1 participates in the steps upstream of autophagosome formation, located in an autophagic region, and forms a complex with UVRAG and Beclin1 as a potential activator of autophagy and suppressor of tumours [Citation3].
Over-expression of SH3GLB1 has an inhibitory effect on cell proliferation. Consequently, SH3GLB1 plays an important role in the occurrence and development of tumours and acts as a tumour growth-suppressing gene in tumour progression [Citation9–11]. The downregulation of SH3GLB1 can suppress the maturation of autophagosomes and accelerate the development of spontaneous tumours in mice [Citation12]. Furthermore, SH3GLB1 inhibits the migration of breast cancer cells by inhibiting endoderm maturation and promoting EGFR degradation [Citation13]. As a result of different types of expression between tumour cells and normal cells, SH3GLB1 is regarded as a biomarker for predicting the occurrence, development and prognosis of cancer [Citation14–16].
Since SH3GLB1 has attracted increasing research attention in recent years, considerable efforts will be put into elucidating the regulatory molecular mechanisms of SH3GLB1. In this article, we identified SH3GLB1 as a potential classical swine fever virus (CSFV)-infection-associated gene in macrophages. Therefore, we used the macrophage as a model and further conducted experiments to investigate the differential expression of SH3GLB1 in CSFV-infected macrophages. Interestingly, upregulation of SH3GLB1 expression was found in macrophages infected by the CSFV Shimen strain.
Materials and methods
Cell culture and virus
Porcine alveolar macrophages (PAMs) were cultured in RPMI 1640 medium (GE, USA) containing 10% fetal bovine serum (FBS; Gibco Life Technologies, USA) at 37 °C with 5% CO2. The CSFV Shimen strain is from the Control Institute of Veterinary Bioproducts and Pharmaceuticals (Beijing, China) [Citation17]. The PAM cell line 3D4/21 (CRL-2843) was obtained from the American Type Culture Collection (Manassas, VA, USA) [Citation18]. RNA and protein were extracted from PAMs co-cultured with the virus for different time points post-infection [Citation17].
Quantitative reverse transcription polymerase chain reaction (qRT-PCR)
The total RNA was extracted by Trizol (Takara, JP) to determine the expression levels of SH3GLB1 with qRT-PCR in PAMs by the following specific oligo-sequences: 5′-TCTTCTTCCAACCTCCCT-3′ and 5′- AATCCATTCCAGCAACAC- 3′. The qRT-PCR thermal cycler program was 95 °C for 15 min, 35 cycles at 95 °C for 10 s, 58 °C for 20 s and a final extension at 72 °C for 31 s (Applied Biosystems 7300, USA). Detection was performed with three replicates of each sample. After collecting the average threshold cycle (Ct) data, these data were analysed and interpreted using the 2−ΔΔCT method [Citation17].
Western blotting
We collected the cells and washed them three times with phosphate-buffered saline (PBS); then we lysed the host cells with RAPI Lysis Buffer (Beyotime, Shanghai, China). Loading quantities of protein were determined by the BCA method. About 30 μg of protein sample was subjected to 10% resolving polyacrylamide (PAA) gel at 90 V for 90 min. We used the PAGE gel to determine the molecular weight of β-actin and SH3GLB1 protein, and then transferred the target protein onto polyvinylidene fluoride (PVDF) membranes (Millipore, Massachusetts, USA) at 100 V for 60 min. After blocking for 2 h at 25 °C ± 2 °C, the membranes were stained at 4 °C overnight with Bif-1 antibody (CST, Massachusetts, USA) diluted 1000-fold and β-actin antibody (Biodragon Immunotech, Beijing, China) diluted 10,000-fold. We then added in the second antibody. ECL detection was done by a Gene Gnome XRQ Chemiluminescence Detector (Syngene, Cambridge, UK), and the gray-scale value of the bands was quantified by Image J (National Institutes of Health, USA).
Confocal microscopy
After fixing the cells in 4% paraformaldehyde at 25 °C± 2 °C for 20 min, and breaking the cell membranes in 1% Triton-X 100 for 8 min, Bif-1 antibodies (CST, USA) and E2 antibodies were incubated with the solution at a 1:100 dilution at 4 °C overnight. After washing with PBS, the cells were incubated with a secondary antibody (YEASEN, Shanghai, China) at a 1:200 dilution for 1 h at 25 °C ± 2 °C. After staining with DAPI for 8 min, the cells were observed using a confocal microscope (LSM510 META, Zeiss, Germany).
Interacting proteins and KEGG pathway analysis
Ten proteins were identified to interact with SH3GLB1 by STRING 10.5 (https://string-db.org/) [Citation19] and Gene ID Conversion Tool in DAVID 6.8 (https://david.ncifcrf.gov/) [Citation20]. Then, the gene ID was used to perform the pathway enrichment analysis by Kobas3.0 (http://kobas.cbi.pku.edu.cn/) [Citation21,Citation22] based on the Kyoto Encyclopedia of Genes and Genomes (KEGG) database.
Statistical analysis
Statistical analysis was conducted by one-way analysis of variance (ANOVA) using the Statistical Package for Social Science (SPSS) 16.0 software (SPSS Inc., Chicago, IL, USA), and the level of p < 0.05 was considered to be significant [Citation23].
Results and discussion
Upregulation of SH3GLB1 mRNA expression by CSFV Shimen infection
We performed digital gene expression (DGE) profiling to investigate the expression of SH3GLB1 in previous studies [Citation17]. As shown in , SH3GLB1 was up-regulated in its clustered heat maps by CSFV Shimen. To validate the DGE analysis data, we further studied the differential expression of SH3GLB1 under CSFV Shimen infection by qRT-PCR. The results from the analysis of qRT-PCR were consistent with the DGE data, showing good agreement between the DGE analysis and RT-PCR (). These results suggest that SH3GLB1 may play key roles in the development of CSFV infection.
Figure 1. mRNA expression of SH3GLB1 during CSFV Shimen infection. (a) DGE analysis showing upregulation of SH3GLB1 with CSFV Shimen infection; (b) SH3GLB1 mRNA expression level during CSFV Shimen infection determined by qRT-PCR analysis.
Note: Statistical analysis was performed by ANOVA; **p < 0.01.

Abundance of SH3GLB1 is positively related to the infection of CSFV Shimen in macrophages
We further considered if the protein level showed the same trend as the mRNA level. In order to confirm that activation of the RNA transcript level resulted in significant up-regulation of SH3GLB1 after CSFV Shimen infection, we detected SH3GLB1 by western blotting. According to the results of the western blotting analysis, the SH3GLB1 protein level increased with increasing infection time (). The expression of β-actin, as a loading control, remained the same in the CSFV Shimen-infected cells. The confocal results showed that the expression level of SH3GLB1 in infected cells was significantly higher than that in mock cells (). These results validated that CSFV infection directly resulted in the upregulation of SH3GLB1 expression in macrophages.
KEGG pathway enrichment analysis of SH3GLB1
For further identification of the functions and metabolic pathways involving SH3GLB1 in CSFV infection, we selected 10 proteins associated with SH3GLB1 via protein interaction analysis () and performed a pathway analysis of these proteins based on the KEGG database (). It was determined that the protein function focused on endocytosis, which plays an important role in the fusion of lysosomes and autophagosomes at a later stage of autophagy [Citation24,Citation25].
Figure 4. Role of SH3GLB1 as predicted by KEGG analysis. (a) Predicted protein–protein interactions involving SH3GLB1; (b) KEGG pathway enrichment analysis predicting SH3GLB1 involvement in several pathways, primarily, endocytosis, ErBb signalling pathway and proteoglycans in cancer.
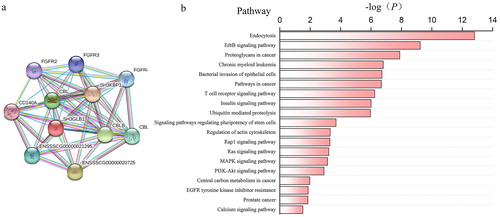
SH3GLB1 is one of the key genes of the autophagy pathway [Citation1–9]. Autophagy, as a kind of physiological phenomenon, degrades cytoplasmic proteins or organelles in order to maintain cellular homeostasis. Autophagosomes are fused with lysosomes in the endocytic pathway that become autolysosomes which are returned to the cytoplasm in the form of amino acids after being degraded [Citation26,Citation27]. Autophagy is widely involved in the development and formation of various physiological diseases.
Former research showed that autophagy played an important role in the process of CSFV infecting host cells [Citation28–31]. It has been reported that autophagy was induced in ST cells after CSFV infection, which is conducive to virus replication [Citation28]. CSFV infection causes a significant increase in the quantity of autophagic vesicles in the cytoplasm and forms 2 ubiquitin-like binding systems, which is considered an important indicator of autophagy, including LC3-I/LC3-II transformation and the ATG12-ATG5 binding system [Citation29]. CSFV replicates and matures with the vesicle membrane after triggering a complete autophagy reaction [Citation31]. Although further studies are required to elucidate the precise role of SH3GLB1 in CSFV-mediated initiation of autophagy, this study identified SH3GLB1 as an activator of autophagy and provided new evidence in support of the concept that induction of autophagy could contribute to the infection of CSFV.
Our results indicate that CSFV Shimen may induce autophagy in macrophages by activating the SH3GLB1 pathway, and SH3GLB1-mediated autophagy may further contribute to CSFV replication. The specific mechanism of CSFV-induced autophagy and promotion of the virus replication through the SH3GLB1 pathway remains to be studied in future.
Conclusions
In this study, we performed qRT-PCR to evaluate the SH3GLB1 mRNA expression and western blotting to detect the abundance of the SH3GLB1 protein. Our results indicated that the expression of SH3GLB1 was significantly up-regulated in macrophages as a result of CSFV Shimen infection. These results will potentially provide a useful basis for further study into the regulation mechanism of SH3GLB1 in the CSFV infection response.
Acknowledgements
The authors thank Wcgene Biotech Shanghai and Dr Karen M. von Deneen (School of Life Science and Technology, Xidian University) for their excellent technical assistance.
Disclosure statement
No potential conflict of interest was reported by the authors.
Funding
This study was supported by the National Natural Science Foundation of China under grant number 31470535.
References
- Takahashi Y, Karbowski MH, Kazi A, et al. Loss of Bif-1 suppresses Bax/Bak conformational change and mitochondrial apoptosis. Mol Cell Biol. 2005;25:9369–9382.
- Pierrat B, Simonen M, Cueto M, et al. SH3GLB, a new endophilin-related protein family featuring an SH3 domain. Genomics. 2001;71:222–234.
- Takahashi Y, Meyerkord CL, Wang HG. Bif-1/endophilin B1: a candidate for crescent driving force in autophagy. Cell Death Differ.. 2009;16:947–955.
- Liu Y, Takahashi Y, Desai N, et al. Bif-1 deficiency impairs lipid homeostasis and causes obesity accompanied by insulin resistance. Sci Rep. 2016;6:20453. [12 p.] DOI:10.1038/srep20453
- Cuddeback SM, Yamaguchi H, Komatsu K, et al. Molecular cloning and characterization of Bif-1. A novel Src homology 3 domain-containing protein that associates with Bax. J Biol Chem. 2001;276:20559–20565.
- Takahashi Y, Meyerkord CL, Wang HG. BARgaining membranes for autophagosome formation: Regulation of autophagy and tumorigenesis by Bif-1/Endophilin B1. Autophagy. 2008;4:121–124.
- Takahashi Y, Coppola D, Matsushita N, et al. Bif-1 interacts with Beclin 1 through UVRAG and regulates autophagy and tumorigenesis. Nat Cell Biol. 2007;9:1142–1151.
- Thoresen SB, Pedersen NM, Liestøl K, et al. A phosphatidylinositol 3-kinase class III sub-complex containing VPS15, VPS34, Beclin 1, UVRAG and BIF-1 regulates cytokinesis and degradative endocytic traffic. Exp Cell Res. 2010;316:3368–3378.
- Kim SY, Oh YL, Kim KM, et al. Decreased expression of Bax-interacting factor-1 (Bif-1) in invasive urinary bladder and gallbladder cancers. Pathology. 2008;40:553–557.
- Xu L, Wang Z, He SY, et al. Bax-interacting factor-1 inhibits cell proliferation and promotes apoptosis in prostate cancer cells. Oncol Rep. 2016;36:3513. [9 p.] DOI: 10.3892/or.2016.5172
- Takahashi Y, Hori T, Cooper TK, et al. Bif-1 haploinsufficiency promotes chromosomal instability and accelerates Myc-driven lymphomagenesis via suppression of mitophagy. Blood. 2013;121:1622–1632.
- Khan MM, Strack S, Wild F, et al. Role of autophagy, SQSTM1, SH3GLB1, and TRIM63 in the turnover of nicotinic acetylcholine receptors. Autophagy. 2014;10:123–136.
- Runkle KB, Meyerkord CL, Desai NV, et al. Bif-1 suppresses breast cancer cell migration by promoting EGFR endocytic degradation. Cancer Biol Ther. 2012;13:956–966.
- Coppola D, Helm J, Ghayouri M, et al. Down-regulation of Bax-interacting factor 1 in human pancreatic ductal adenocarcinoma. Pancreas. 2011;40:433–437.
- Naponelli V, Modernelli A, Bettuzzi S, et al. Roles of autophagy induced by natural compounds in prostate cancer. Biomed Res Int. 2015;2015:1–14.
- Lee JW, Jeong EG, Soung YH, et al. Decreased expression of tumour suppressor Bax-interacting factor-1 (Bif-1), a Bax activator, in gastric carcinomas. Pathology. 2006;38:312–315.
- Ning P, Hu C, Li X, et al. Classical swine fever virus Shimen infection increases p53 signaling to promote cell cycle arrest in porcine alveolar macrophages. Oncotarget. 2017;8:55938. [12 p.] DOI: 10.18632/oncotarget.18997
- Ning P, Gao L, Zhou Y, et al. Caveolin-1-mediated endocytic pathway is involved in classical swine fever virus Shimen infection of porcine alveolar macrophages. Vet Microbiol. 2016;195:81–86.
- Szklarczyk D, Franceschini A, Wyder S, et al. STRING v10: protein–protein interaction networks, integrated over the tree of life. Nucleic Acids Res. 2015;43:D447–D452.
- Huang DW, Sherman BT, Lempicki RA. Systematic and integrative analysis of large gene lists using DAVID Bioinformatics Resources. Nat Protoc.. 2009;4:44–57.
- Xie C, Mao X, Huang J, et al. KOBAS 2.0: a web server for annotation and identification of enriched pathways and diseases. Nucleic Acids Res. 2011;39:W316–W322.
- Wu J, Mao X, Cai T, et al. KOBAS server: a web-based platform for automated annotation and pathway identification. Nucleic Acids Res. 2006;34:W720–W724.
- Ning P, Zhou Y, Liang W, et al. Different RNA splicing mechanisms contribute to diverse infective outcome of classical swine fever viruses of differing virulence: insights from the deep sequencing data in swine umbilical vein endothelial cells. Peerj. 2016;4:e2113. p.] DOI: 10.7717/peerj.2113
- Varin A, Gordon S. Alternative activation of macrophages: immune function and cellular biology. Immunobiology. 2009;214:630–641.
- Lamb CA, Dooley HC, Tooze SA. Endocytosis and autophagy: Shared machinery for degradation. Bioessays. 2013;35:34–45.
- Levine B, Kroemer G. Autophagy in the pathogenesis of disease. Cell. 2008;132:27–42.
- Klionsky DJ, Emr SD. Autophagy as a regulated pathway of cellular degradation. Science. 2000;290:1717–1721.
- Luo Q, Zhang L, Wei F, et al. mTORC1 Negatively regulates the replication of classical swine fever virus through autophagy and IRES-dependent translation. iScience. 2018;3:87–101.
- Gou H, Zhao M, Fan S, et al. Autophagy induces apoptosis and death of T lymphocytes in the spleen of pigs infected with CSFV. Sci Rep. 2017;7:13577[19 p.] DOI:10.1038/s41598–017–14082-9
- Pei J, Deng J, Ye Z, et al. Absence of autophagy promotes apoptosis by modulating the ROS-dependent RLR signaling pathway in classical swine fever virus-infected cells. Autophagy. 2016;12:1738–1758.
- Pei J, Zhao M, Ye Z, et al. Autophagy enhances the replication of classical swine fever virus in vitro. Autophagy. 2014;10:93–110.