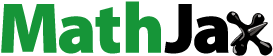
Abstract
Dengue virus (DENV) is a globally significant human pathogen that annually infects more than ∼300 million of the Earth’s population. While the clinically approved anti-dengue vaccine, Dengvaxia is available for treating such infection, its treatment is limited to those infected with DENV of serotypes 3 and 4 only. However, the vaccine is no longer available to treat dengue infections following the suspended use of Dengvaxia after several discoveries on the side effects of the vaccine on humans. In view of this recent development, a collection of plant extracts in the MyNature50000 library showing potential anti-dengue activities were explored. In this work, the DENV2 NS2B-NS3 bioassay was setup and optimized to evaluate the potential inhibitory activity of plant extracts stored in the MyNature50000 natural product collections. A total of 150 plants were examined using the high-throughput screening assay, among which four candidate plants showed potential as inhibitors of the DENV2 NS2B-NS3 protease, with exceptional inhibition activity >95% with corresponding IC50 values ranging within 2 − 5 µg/mL. Two local plants, Syzygium campanulatum (‘Kelat Paya’) and Syzygium grande (‘Keriang Batu’), were phytochemically analyzed and the data revealed existence of cyclododecane, n-hexadecanoic acid and caryophyllene, all of which are compounds well-known for their inhibitory activity against DENV2 NS2B-NS3. Additionally, MTT assays showed that both plants were mildly cytotoxic on mammalian cell lines, with IC50 values >20 µg/mL, indicating their prospective use as drugs to combat DENV.
Introduction
According to the World Health Organization, dengue virus (DENV) infection is a worldwide problem. DENV is deemed a public health risk to an estimated 2.5 billion people inhabiting areas where dengue is prevalent, infecting between 50 and 100 million people each year [Citation1]. Most dengue cases appear asymptomatic, particularly in children as well as in adults following a first infection. Sometimes the infection seems self-limited and manifests as undifferentiated fever or classic dengue fever [Citation2]. However, dengue haemorrhagic fever, if left untreated, has a mortality rate as high as 10 − 20%. Ramifications such as morbidity rates and economic impact due to dengue appear to have surpassed that of malaria [Citation3]. Hence, there is a crucial need for continuous efforts in searching and developing effective anti-dengue therapeutics to combat this epidemic infection.
DENV may be endemic in certain countries but the infection may be spread to individuals travelling from non-endemic areas to the dengue-affected areas [Citation2], much to the concern of international public health authorities. Although large investments continue to pour into anti-DENV research, a clinically approved antiviral therapeutic agent or vaccine to treat humans infected with the disease has yet to be found [Citation1,Citation4]. This is of particular importance, considering the suspension of the newly approved dengue tetravalent vaccine, Dengvaxia® after several reports of systemic adverse events such as erythema and swelling in patients.
So far, attempts into development of a dengue antiviral agent have been very challenging. This is because the four dengue serotypes, DENV1, DENV2, DENV3 and DENV4, are highly prone to mutation due to the error-prone nature of their RNA polymerase that forms quasispecies [Citation2]. In the host cell, DENV RNA is translated into a single polyprotein which is then cleaved by the host proteases and the virus-encoded two-component protease (NS2B-NS3pro). The cleaving process produces three other structural proteins viz. the capsid, membrane and envelope, and seven other non-structural proteins viz. NS1, NS2A, NS2B, NS3, NS4A, NS4B and NS5 [Citation5,Citation6]. The serine protease of the N-terminal domain in the non-structural NS3 then hydrolyses the polyprotein, whereas the C-terminal domain exhibits ATPase/helicase enzymatic activity. A 40-residue segment of the NS2B protein acts as a cofactor for NS3 to stabilize the protein folding [Citation7,Citation8]. In this work, the DENV2 NS2B-NS3 protease is the prime drug target due to its essential role in the replication of DENV.
Exploration works towards discovery of new potential lead compounds from animals, microorganisms and plants have continued for the past few years via screening approaches [Citation9]. Survey of literature revealed that crude polar (ethanol, methanol or water) plant extracts show higher quantities of flavonoids and alkaloids, as well as cyclohexenyl chalcone, all of which are reported to inhibit several types of flaviviruses, for instance, DENV, yellow fever virus and West Nile virus (WNV). However, according to a report by Szymanski et al. [Citation10], methanol plant extracts showed higher inhibitory effects on the DENV protease than ethanol extracts. Interestingly, traditional folk medicine practiced among the Malaysians uses the juice from of Carica papaya for the treatment of dengue. In the case of C. papaya, the potency of the juice of its leaf extracts may be directly due to the presence of a wide variety compounds known for their antioxidant, anti-inflammatory and hypoglycaemic effect [Citation11].
Likewise, other natural inhibitors have also demonstrated anti-viral activity, such as polyphenols which include delphinidin, epigallocatechin [Citation12] and indole derivatives [Citation13]. Driven by this idea, we performed a search for prospective DENV2 NS2B-NS3 inhibitors among the crude plant extracts in the MyNature50000 library (Malaysian Plant Natural Product Repository and Database) at the Malaysian Institute of Pharmaceuticals and Nutraceuticals, located on the northern region of Peninsular Malaysia. The repository contains over 500 Malaysian plants collected around the northern region of Peninsular Malaysia. Researchers have so far isolated a total of 552 extracts, 2064 partitions and 3479 fractions as well as identified 150 natural compounds from the repository. Consequently, the methods to identify potential NS2B-NS3 protease inhibitors from a variety of sources have typically used rationally designed substrate-based peptidomimetics and screening natural products, structure-based virtual screening as well as high-throughput screening (HTS) [Citation6]. Nonetheless, HTS has become the standard technique to identify potential drugs discovery [Citation14], specifically in the identification of potential DENV and WNV inhibitors [Citation15,Citation16].
In this study, HTS was focused on the identification of plant extracts in the MyNature50000 library showing DENV2 NS2B-NS3 inhibitor activity. First, we screened 150 plant crude extracts, and only plants that exhibited exceptional anti-DENV2 NS2B-NS3 activity were subjected to further phytochemical and cytotoxicity tests. Next, MTT assay using human uroepethelial carcinoma cell line (HTB-4) and normal human uroepethelial cell line (SV-HUC-1) was employed to assess the safety of the selected crude extracts. The data presented here may prove useful in providing an alternative avenue in the search of inhibitors that target the NS2B-NS3 dengue viral protease.
Materials and methods
Plant material
Parts of plants were collected around the Northern Region of Peninsular Malaysia, in the states of Kedah, Perak and Pulau Pinang. Identification of plants was done by Dr. Rahmad Zakaria at the Herbarium Unit, School of Biological Sciences, Universiti Sains Malaysia. Voucher specimens are deposited at the Herbarium Unit, Natural Product Drug Discovery Centre (NPDC), Malaysian Institute of Pharmaceuticals and Nutraceuticals (IPharm).
Expression and purification of NS2B-NS3 in Escherichia coli
The recombinant pET14-NS2B-NS3 was expressed in Escherichia coli BL21 (DE3) according to a previous work by Heh et al. [Citation13]. E. coli BL21 (DE3) containing a pET14-NS2B-NS3 expression vector was inoculated into Luria–Bertani (LB) medium (containing 100 µg/mL ampicillin) and was incubated overnight at 37 °C. A 10 mL aliquot of the culture was transferred into LB broth (1 L) and incubated at 37 °C with agitation at 200 rpm until the optical density (OD600) of the culture reached a value of 0.8. The cells were induced with 0.5 mmol/L IPTG (isopropyl β-d-1-thiogalactopyranoside), incubated overnight and harvested by centrifugation (10,000×g, 10 min, 4 °C). Cells were sonicated, ultracentrifuged (10,000×g, 10 min, 4 °C) and the protein suspension was filtrated through a membrane filter (0.22 µm). The crude His-tagged fusion protein was purified using a Ni-NTA (nitrilotriacetic acid) agarose column at 4 °C. The column-retained protein was initially washed with buffer A, Tris–HCl (pH 8.5, 0.5% glycerol, 20 mmol/L imidazole) and the pure protein was eluted with buffer B, Tris–HCl (pH 8.5, 0.5% glycerol, 250 mmol/L imidazole).
Preparation of plant extracts
Macerated plant material was dissolved in 500 mL and 5 L of methanol, respectively [ratio of 1:5 (w/v)] for 72 h. Each plant extract was filtered sequentially through a Büchner funnel and a Whatman No. 1 filter paper, and the filtrates were concentrated at 40 °C under reduced pressure Kawamura et al. [Citation14] using a Rotary evaporator EYELA to give a dark-green residue of 200 and 8 g, respectively.
DENV2 NS2B-NS3 protease inhibition assay
The inhibition assay was performed according to the protocol proposed by Rothan et al. [Citation8]. A reaction mixture of 100 µL aliquots each was prepared by dissolving fluorogenic peptide (Boc-Gly-Arg-Arg-MCA) (10 mmol/L) and recombinant DENV2 NS2B-NS3 (0.5 µmol/L) in Tris–HCl buffer (200 mmol/L, pH 8.5). For the assay, three parallel reactions were performed viz. buffer only, buffer with enzyme and buffer with the enzyme, using 200 µg/mL of plant extract. Drug inhibitors were pre-incubated with the NS2B-NS3pro at 37 °C for 10 min prior to the addition of the fluorogenic peptide substrate.
The assay mixture was incubated at the same temperature (37 °C) for another 1 h. The assay conditions were validated by simultaneously running a blank control (100 µL of 200 mmol/L Tris–HCl only) and a positive control (100 µmol/L substrate BOC-GRR-MCA and 0.5 µmol/L NS2B-NS3pro in 200 mmol/L Tris–HCl) and the experiment was carried out in quadruplicate (n = 4). The percentage of DENV2 NS2B-NS3 protease inhibition by the peptide inhibitor was measured by fluorescence emission spectroscopy using a Tecan M1000 PRO 96-well microplate reader for emissions between 410 and 460 nm upon excitation at 365 nm. The inhibitory activity was calculated according to EquationEquation (1)(1)
(1) :
(1)
(1)
(2)
(2)
where RFU is the relative fluorescence units.
High-throughput screening (HTS)
To increase the efficiency and throughput, the HTS assay for screening DENV2 NS2B-NS3 inhibitors was conducted by converting the fluorescence-based assay to a miniaturized 96-well plate format.
Preparation of source plate and 96-well destination plate
The source plate was comprised of recombinant DENV2 NS2B-NS3 (0.5 µmol/L), 100 µL Tris–HCl (200 mmol/L, pH 8.5), fluorogenic substrate BOC-GRR-MCA (100 µmol/L) and plant extracts (200 µg/mL) dissolved in dimethyl sulphoxide (DMSO). Subsequently, 1 µL of the plant extract was transferred from the source plate into a 96-well destination plate using the automated TECAN Freedom EVO 100 liquid handling system. Then, DENV2 NS2B-NS3 was added to the plant extract (200 µg/mL) and pre-incubated for 10 min 37 °C, before the fluorogenic peptide substrate was added to initiate the reaction. The assay mixture was further incubated with shaking at 100 rpm for 1 h at 37 °C before measurement. The resulting fluorescence was measured at λexcitation = 365 nm and λemission = 410–460 nm using Tecan M1000 PRO 96-well advanced microplate reader.
Determination of IC50
Crude methanol plant extracts exhibiting inhibitory activity >95% were then selected for determination of the half maximal inhibitory concentration (IC50). Serial dilutions of 200, 100, 50, 25, 12.5, 6.25 and 3.125 µg/mL, for each type of crude methanol plant extract were prepared. Preliminary screening of various plant extracts showed that the IC50 values of Syzygium campanulatum (‘Kelat Paya’) and Syzygium grande (‘Keriang Batu’) indicate promising DENV2 NS2B-NS3 protease inhibitory activity. Hence these extracts were further assessed for phytochemical and cytotoxicity analysis. Both plants were subjected to extraction with different solvents, methanol (MeOH), ethyl acetate (EtOAc) and ethyl acetate-methanol (EtOAc-MeOH) (1:1 ratio) followed by HTS. Selected plant extracts were then subjected to DENV2 NS2B-NS3 enzyme assay and phytochemical analysis via gas chromatography–mass spectrometry (GC-MS).
Compound identification of different plant extracts using GC-MS
Carbohydrates and protein contaminants were removed from the MeOH, EtOAc, and EtOAc-MeOH leaves extract of S. campanulatum and S. grande as described by Bligh and Dyer [Citation17]. Plant extract (50 mg) was resuspended in methanol (0.5 mL) (LCMS grade) and vortexed for 1 min at 25 °C before the addition of LCMS grade water (0.2 mL). The mixture was vortexed for 1 min, followed by the addition of dichloromethane (0.1 mL) and vortexed further for an additional 2 min. The sample was incubated with shaking at 800 rpm at 20 °C for 1 h. The samples were collected by centrifugation at 10,000×g for 3 min at 20 °C, and the lower layer (organic phase) was transferred into a new tube and vacuum-dried at temperature below 30 °C. The dried sample was suspended in ethanol (0.05–0.1 mL) and filtered through a 0.45 µm filter for GC-MS analysis.
The leaf extracts of S. campanulatum and S. grande were subjected to GC-MS in solid-phase micro-extraction (SPME) mode using an Agilent 7890A gas chromatograph equipped with an Agilent 5975C MSD. The injector temperature was set at 250 °C and set using split mode (1:50). The volatile compounds were separated in a HP-5MS capillary column (30 m × 250 µm inner diameter × 0.25 µm film) with helium as the carrier gas at a flow rate of 1 mL/min. The oven temperature was initially maintained at 40 °C for 2 min, followed by a two-step temperature increased to 175 °C at a rate of 5 °C/min, then to 250 °C at 90 °C/min. The temperature of the ion source and transfer line were set at 220 and 280 °C, respectively and the electron impact mass spectra was recorded at 70 eV ionisation energy. Volatile compounds were identified by mass spectra comparison using MSD Chemstation Enhanced Data Analysis Software (E.02.02.1431 version, Agilent Technologies) and compared against the National Institute of Standards and Technology library database (NIST 14).
Cytotoxicity test of plant extracts using MTT assay
The cytotoxicity of each plant extract was tested in vitro using SV-HUC-1 and HTB-4 by 3-(4,5-dimethylthiazol-2-yl)-2, 5-diphenyltetrazolium bromide (MTT) assay as described by Mosmann [Citation15]. When the cultured cells reached 100% confluence, the cells were harvested with 2 mL of Trypsin EDTA (GIBCO) and seeded at 5 × 104 cells/mL in each well of the 96-well plate containing the complete medium. After 24 and 48 h incubation for SV-HUC-1 and HTB-4 cells attachment, respectively, each medium was replaced with 150 µL/well synchronising medium containing 0.5% serum. After 24 h, the synchronising medium was removed and replaced with 150 µL/well fresh complete growth media (10% serum) and incubated for another 24 h. Next, the cells were treated with 100 µL of different concentrations (1.65, 3.125, 6.25, 12.5, 25, 50, 100 and 200 µg/mL) of each plant extract (S. grande and S. campanulatum methanol extract) that was serially diluted in the growth medium and 100 µL plain medium was used as the blank. Chloroquine (100 µL) was dissolved in the medium as the positive control, whereas DMSO (100 µL) diluted in the medium as the second positive control; medium containing cells only was the negative control. The plates were incubated in a CO2 incubator for 72 h. As a biological control, three plates were prepared for each cell line and plant extract, and a mechanical control triplicate for each well was also carried out.
MTT assay was conducted to determine the viability of cells as indicated by cellular NADP-H dependent cellular-oxidoreductase enzyme activity. The plant extract was removed, MTT reagent (5 mg/mL) was loaded into each well containing cells and incubated in darkness for 4 h. The MTT reagent was then removed followed by the addition of DMSO (100 µL). The 96-well plate was analyzed under fluorescence wavelength of 560 nm using a Tecan Spectrophotometry reader. All test concentrations were run in triplicate to check for technical errors, whereas three plates for each cell line were used to account for any biological error. The result was expressed as a log cell viability percentage to log treatment concentration, and then calculated using EquationEquation (2)(2)
(2) :
where Asample is the absorbance of the treated cells at a certain concentration of the plant extract, Ablank is the absorbance of the blank, and Acontrol is the absorbance of the cells treated with chloroquine. The IC50 value was determined by plotting the values; log of cell viability percentage versus the log of respective concentration of the plant extract.
Data analysis
Data in this study are presented as mean values with standard deviation (±SD).
Results and discussion
DENV-2 NS2B-NS3 protease inhibitory assay
In the present study, recombinant NS2B-NS3 protease for the in vitro HTS assay system was expressed and purified. Based on HTS screening data tested on plant extracts at a concentration of 200 µg/mL, 10 out of 150 plant samples exhibited over 90% inhibitory activity against NS2B-NS3 protease (). Further tests revealed that four out of the ten plant extracts demonstrated the highest NS2B-NS3 protease inhibitory activity, with percentage inhibition of more than 95%. The four plant extracts were then chosen for the IC50 test.
Table 1. Top 10 plant species with high inhibitory activity towards NS2B/NS3 protease.
IC50 of selected plant extracts
The results revealed that extracts from plants designated as IPNAT 45a/M01 and IPNAT 11b/M01, locally known as ‘Keriang Batu’ (S. grande) and ‘Kelat Paya’ (S. campanulatum), respectively, of the family Myrataceae and genus Syzyqium, showed promising IC50 values of 3.7673 and 2.4397 µg/mL, respectively (). Moreover, the ‘Keriang Batu’ leaf extract showed a lower IC50 value than the stem extracts of 3.7673 and 5.2275 µg/mL, respectively. This indicated differences in the composition and concentration of compounds having potential to inhibit DENV2 NS2B-NS3 protease from different parts of the plant. Further extraction and phytochemical analysis were conducted to find positive correlation between the potential compounds content and the inhibitory activity.
To determine the extraction conditions for potential compounds form both plants, S. grande and S. campanulatum, with optimum DENV2 NS2B-NS3 inhibitory activity, we performed extraction with three different solvents, methanol (MeOH), ethyl acetate (EtOAc) and ethyl acetate-methanol (EtOAc-MeOH). Interestingly, the three different solvent extracts from S. grande showed no significant differences in their inhibitory activity, whereas the EtOAC extracts from S. campanulatum showed a higher potential to inhibit DENV2 NS2B-NS3 protease activity as compared to the other two different solvents ().
Table 2. Inhibition of dengue virus NS2B/NS3 protease by different extracts of Syzygium grande (IPNAT 45a/M01) and Syzygium campanulatum (IPNAT 11a/M01).
The obtained data, hence, imply that extracts of S. grande and S. campanulatum could have potential use as DENV2 NS2B-NS3 inhibitors. The findings also corroborate an earlier observation by Mohamed et al. [Citation16] on plants of the genus Syzyqium (family Myrataceae) having therapeutic properties, likely attributable to the plant being rich in tannins, alkaloids, steroids, flavonoids and terpenoids. A minimal inhibition concentration against DENV-2 of 100 µg/mL was observed for the plant Piper ractrofractum of the Piperaceae family. Unlike plant extracts from the genus Syzyqium, hyperoside is the crucial active compound in the extracts of P. ractrofracum which imparted the inhibitory effect against DENV-2 [Citation18]. Other species from the same genus have been similarly reported to contain high concentrations of flavonoids, known for their ability to scavenge free radicals [Citation19].
Phytochemical analysis of potential anti-dengue plant extracts
The presence of various phytochemicals with anti-dengue activity, such as flavonoids, in many plants has been vastly studied. To investigate the composition of chemical constituents present in the three different extracts, the MeOH, EtOAc and EtOAc-MeOH extracts of S. grande and S. campanulatum samples in a ratio of 1:1 were subjected to GC-MS ( and ).
Figure 1. GC-MS chromatogram of MeOH (a), EtOAc (b) and EtOAc-MeOH (c) leaf extracts of Syzygium grande.
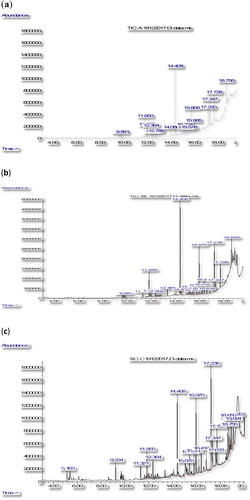
Figure 2. GC-MS chromatogram of MeOH (a), EtOAc (b) and EtOAc-MeOH (c) leaf extracts of Syzygium campanulatum.
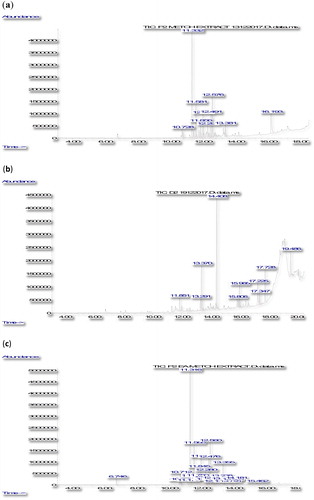
As shown in the chromatograms of the S. grande extracts (), the highest chromatogram peak was recorded at 14.4 min in the MeOH and the EtOAc extracts () and at 17.2 min in the EtOAc-MeOH extract (). When compared with the compound library, the peaks were identified as cyclododecane (RT = 14.408) and n-hexadecanoic acid (RT = 17.236), respectively. The corresponding GC-MS chromatograms of the MeOH, EtOAc and EtOAc-MeOH extracts of S. campanulatum, revealed two major peaks at retention time 11.3 min in the MeOH and the EtOAc-MeOH extract () and at 14.4 min in the EtOAc extract (). The peaks were identified as caryophyllene (RT = 11.332, 11.316) and cyclododecane (RT = 14.408). Most importantly, the findings indicated that cyclododecane, n-hexadecanoic acid and caryophylline were the common bioactive agent found in all extracts of S. grande and S. campanulatum showing promising DENV2 NS2B-NS3 protease inhibition.
Furthermore, the obtained data are in good agreement with Santoyo et al. [Citation20], who reported that cyclododecane and n-hexadecanoic acid were present in good quantities in extracts of Himanthalia elongate, an edible seaweed, which showed antiviral activity against herpes simplex virus. Cyclododecane was also discovered in the leaf extract of Morus rotunbiloba Koidz that exhibited anti-viral properties [Citation21].
Various other compounds were also identified in the extract of S. campanulatum leaves ( and ). MeOH extracts gave five compounds, while the EtOAc and EtOAc-MEOH extracts gave five and seven types of compounds, respectively. All three S. campanulatum leaves extracts appeared to share a common compound, i.e. caryophyllene, which is a terpenoid belonging to the sub-class of sesquiterpenes [Citation21]. Although extracts of S. campanulatum leaves were expected to contain different isoforms of caryophyllene, their biological and chemical properties should remain relatively similar. Hence, based on the observed data, caryophyllene could be classified as a potential lead-compound in S. campanulatum leaf extracts which imparted the inhibitory effect on DENV-NS2B-NS3 protease. However, Alesic and Knezevic [Citation22] conjectured that caryophyllene may react in synergy with other chemical compounds within the plant to impart the observed inhibitory effect on DENV-NS2B-NS3 protease. As a matter of fact, Djilani and Dicko [Citation23] provided proof of the synergistic effect between caryophyllene and plant compounds that resulted in higher antiviral activity; rather than this compound alone.
Table 3. Biologically active chemical compounds in MeOH, EtOAc and EtOAc-MeOH leaf extracts from Syzygium grande.
Table 4. Biologically active chemical compounds in MeOH, EtOAc and EtOAc-MeOH leaf extracts from Syzygium campanulatum.
Correspondingly, several high polarity volatile compounds have been isolated from other plants belonging to the same genus Syzygium, including triterpene glycoside, myricetin 4-O-methylether-3-O-α-l-rhamnopyranoside, myricetin 3-O-β-d-glucopyranoside, ursolic acid, quadranoside IV, friedelin, β-sitosterol, botulin, phenethyl d-rutinoside, officinoside B, crotalionoside C, and n-butyl-b-d-fructopyranoside [Citation24]. In another study, the leaf extract of S. campanulatum Korth was shown to contain two types of flavanones which were later identified as (2S)-7-hydroxy-5-methoxy-6,8-dimethyl flavanone and (S)-5,7-dihydroxy-6,8-dimethyl-flavanone, along with the known chalcone, (E)-2′,4′-dihydroxy-6′-methoxy-3′,5′-dimethylchalcone and two triterpenoids, namely, betulinic and ursolic acids which reportedly impart anti-proliferative activity to the isolated flavanone compounds [Citation25]. Volatile compounds are also found in the Syzygium genus and therapeutically used such as S. aromatic. Eugeniin is a common component found in the volatiles and has been identified as a potential inhibitor against viral DNA replication of herpes simplex virus [Citation26] Other interesting findings showed the Psidium guajava plant from the family of Syzygium (Myrtaceae) to inhibit the growth of DENV [Citation27].
Cell cytotoxicity assay of plant extracts with potential anti-dengue activity
The MTT assay results are interpreted by the observation of changes in colour intensity, due to the enzymatic conversation reaction. MTT assay was chosen as the standard for cytotoxicity test as the technique has been acknowledged as the ‘gold standard’ assay as compared to others. It is worth noting here that the data presented here are the first detailing of the cytotoxic effect of S. grande and S. campanulatum plant extracts on human cell lines, i.e. SV-HUC-1 (human uroepithelial cell line) and HTB-4 (human uroepithelial cancer cell line).
shows the survival curves of SV-HUC-1 and HTB-4 cell lines treated with S. grande and S. campanulatum methanol leaf extracts. Both the SV-HUC-1 and HTB-4 cell lines treated with S. grande leaf extracts showed higher percentages of cell survival as compared to the cell lines treated with S. campanulatum leaf extracts. These data appear to suggest that the compounds in the S. grande leaf extracts were more potent in inhibiting DENV-NS2B-NS3 protease. However, upon comparison, a higher percentage of cell survival was observed for SV-HUC-1 cells as compared to the cancer cell line HTB-4. This in fact, indicates that the tested plant extracts exhibited anti-mitotic effect on the cancer cells, implying that the plant extracts may contain appreciable quantities of antimitotic compounds, possibly atorvastatin and trans-caryophyllene [Citation28].
Figure 3. Survival curves of SV-HUC-1 and HTB-4 cell lines treated with various concentrations of Syzium grande and Syzium campanulatum methanol leaf extracts.
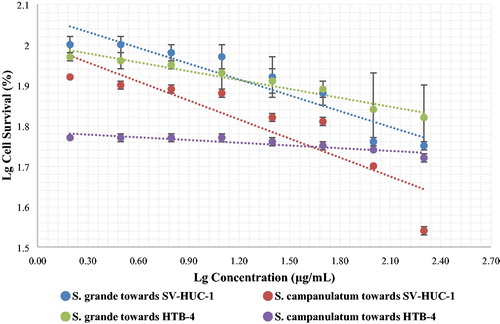
The IC50 values for both cell lines treated with the selected methanol extracts are presented in . For reference, the guidelines set by the National Cancer Institute (NCI) describe that plant extracts or compounds with IC50 values of less than 20 µg/mL are deemed as cytotoxic and, noncytotoxic, if otherwise [Citation28]. Hence, the high IC50 values corresponding to values 182.57 µg/mL and 295.55 µg/mL as well as 122.21 and 256.59 µg/mL, for SV-HUC-1 and HTB-4 cell lines treated with methanol leaf extracts of S. grande and S. campanulatum, directly indicated that the extracts were non-toxic, and could be safely used as a potential DENV-NS2B-NS3 protease inhibiting agent. Although the cancer cell lines did exhibit a lower than expected cell survival percentage, this effect may not necessarily be a direct representation of the cytotoxic effect of the methanol plant extracts. In short, it appears that the polar extracts of leaves of S. grande and S. campanulatum exhibited promising anti-dengue protease properties at concentrations that are likely innocuous for humans, as represented by minute cytotoxicity effect on the tested human cell lines.
Table 5. IC50 values of S. grande and S. campanulatum methanol leaf extracts in SV-HUC-1 and HTB-4 cell lines.
Conclusions
In summary, the extraction, identification and characterization of several compounds in the polar leaf extracts of S. grande and S. campanulatum revealed potential new sources of anti-dengue agents which specifically act on inhibiting activity of DENV2 NS2B-NS3 protease. The findings revealed that cyclododecane and n-hexadecanoic acid found in the leaf extracts of S. grande and caryophyllene in extracts of S. campanulatum were likely the lead-compound that imparted the observed inhibitory effect on the DENV2 NS2B-NS3 protease. However, further purification on extracts of both plants may be necessary to elucidate the actual mechanisms inflicted by each compound that contributed to inhibition of the dengue protease. Future studies should also assess the structure–activity relationship between the DENV2 NS2B-NS3 protease and the abovementioned compounds, to further substantiate the present findings.
Disclosure statement
No potential conflict of interest was reported by the authors.
Funding
This study was funded by supported by the Ministry of Science, Technology and Innovation (MOSTI) under grant number PKA0514B006.
References
- Yang CC, Hu HS, Wu RH, et al. A novel dengue virus inhibitor, BP13944, discovered by high-throughput screening with dengue virus replicon cells selects for resistance in the viral NS2B/NS3 protease. Antimicrob Agent Chemother. 2014;58:110–119.
- Ng L-C, Chem Y, Koo C, et al. Dengue outbreaks in Singapore and Malaysia caused by different viral strains. Am J Trop Med Hyg. 2015;92:1150–1155.
- Sarala N, Paknikar SS. Papaya extract to treat dengue: a novel therapeutic option. Ann Med Health Sci Res. 2014;4:320–324.
- Simmons CP, Farrar JJ, Nguyen VV, et al. Dengue. N Engl J Med. 2012;366:1423–1432.
- Chambers TJ, Hahn CS, Galler R, et al. Flavivirus genome organization, expression, and replication. Annu Rev Microbiol. 1990;44:649–688.
- Yang CC, Hsieh YC, Lee SJ, et al. Novel dengue virus-specific NS2B/NS3 protease inhibitor, BP2109, discovered by a high-throughput screening assay. Antimicrob Agent Chemother. 2011;55:231–233.
- Niyomrattanakit P, Winoyanuwattikun P, Chanprapaph S, et al. Identification of residues in the dengue virus type 2 NS2B cofactor that are critical for NS3 protease activation. J Virol. 2004;78:13708–13716.
- Rothan HA, Abdulrahman AY, Sasikumer PG, et al. Protegrin-1 inhibits dengue NS2B-NS3 serine protease and viral replication in MK2 cells. J Biomed Biotechnol. 2012;2012:1251482.
- Teixeira RR, Pereira WL, Oliveira AF, et al. Natural products as source of potential dengue antivirals. Molecules. 2014;19:8151–8176.
- Szymanski P, Markowicz M, Mikiciuk-Olasik E. Adaptation of high-throughput screening in drug discovery toxicological screening test. Int Mol Sci. 2012;13:427–452.
- Qing M, Liu W, Yuan Z, et al. A high-throughput assay using dengue-1 virus-like particles for drug discovery. Antivir Res. 2010;86:163–171.
- Johnston PA, Phillips J, Shun TY, et al. HTS identifies novel and specific uncompetitive inhibitors of the two-component NS2B-NS3 proteinase of West Nile virus. Assay Drug Dev Technol. 2007;5:737–750.
- Heh CH, Othman R, Buckle MJC, et al. 2013. Rational discovery of dengue type 2 non-competitive inhibitors. Chem Biol Drug Des. 2013;82:1–11.
- Kawamura F, Ramle SFM, Sulaiman O, et al. Antioxidant and antifungal activities of extracts selected hardwood species of Malaysian timber. Eur J Wood Prod. 2011;69:207–212.
- Mosmann T. Rapid colorimetric assay for cellular growth and survival: application to proliferation and cytotoxicity assays. J Immunol Methods. 1983;65:55–63.
- Mohamed AA, Ali SI, El-Baz FK. Antioxidant and antibacterial activities of crude extracts and essential oils of Syzygium cumini leaves. PLoS ONE. 2013;8:60269.
- Bligh EG, Dyer WJ. A rapid method for total lipid extraction and purification. J Biochem Physiol. 1959;37:911–917.
- Kadir SLA, Yaakob YH. Potential anti-dengue medicinal plants: a review: J Nat Med. 2013;67:677–689.
- Ravi K, Ramachandran B, Subramanian S. Protective effect of Eugenia jambolana seed kernel on tissue antioxidants in streptozotocin induced diabetic rats. Biol Pharm Bull. 2004;27:1212–1217.
- Santoyo S, Plaza M, Jaime L, et al. Pressurized liquids as an alternative green process to extract antiviral agents from Himanthalia elongate. J Appl Phycol. 2011;23:909–917.
- Patharakorn T, Arpornsuwan T, Wetprasit N, et al. Antibacterial activity and cytotoxicity of the leaf of Morus rotunbiloba Koidz. J Med Plants Res. 2010;4:837–843.
- Aleksic V, Knezevic P. Antimicrobial and antioxidative activity of extracts and essential oils of Myrtus communis L. J Microb Res. 2014;169:247–251.
- Djilani A, Dicko A. Nutrition, well-being and health. Croatia: InTech; 2012. Bouayed J, editor. The therapeutic benefits of essential oils; p. 155–178.
- Samy MN, Sugimoto S, Matsunami K, et al. One new flavonoid xyloside and one new natural triterpene rhamnoside from the leaves of Syzygium grande. J Phytochem Lett. 2014;10:86–90.
- Memon AH, Ismail Z, Al-Suede FS, et al. Isolation, characterization, crystal structure elucidation of two flavanones and simultaneous RP-HPLC determination of five major compounds from Syzygium campanulatum Korth. Molecules. 2015;20:14212–14233.
- Jassim SAA, Naji MA. Novel antiviral agents: a medicinal plant perspective. J Appl Microbiol. 2003;95:412–427.
- Qadir I, Abbas K, Tahir M, et al. Review: dengue fever: natural management. Pak J Pharm Sci. 2015;28:647–655.
- Campos MI, Vieira WDA, Campos CN, et al. Atorvastatin and trans-caryophyllene for the prevention of leukopenia in an experimental chemotherapy model in Wistar rats. Mol Clin Oncol. 2015;3:825–828.