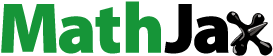
Abstract
Waste biomass from Bacillus cereus immobilized in sodium alginate and co-immobilized with activated carbon or with bentonite into alginate gel was studied for Pb(II), Cd(II) and Hg(II) removal from aqueous solutions. The composite biosorbent consisting of waste B. cereus biomass co-immobilized with activated carbon into alginate beads was selected as the most prospective for heavy metals removal. Immobilization increased both the removal capacity and the mechanical strength of the biosorbent. Major process parameters were optimized and maximum removal efficiency of 92.13% was reached for Pb(II) ions at pH 5.0, biosorbent dosage 2 g/L, temperature 25 °C, agitation speed 120 rpm for 120 min.
Introduction
Current stage of development of various industries causes constantly increasing amounts of pollutants annually discharged into the environment. It has been proved that large volumes of industrial effluents, in particular, waste waters contaminated by heavy metals, such as copper, lead, chromium, cadmium, mercury and others, affect mainly aquatic ecosystems. For their toxicity and possibilities for bioaccumulation through the food chain, heavy metals have become one of the major risks for human health. Probably heavy metal pollution is one of the most serious ecological problems nowadays.
Various methods have been proposed for removal of metal ions from aqueous solutions, such as chemical precipitation [Citation1–3], electrodeposition [Citation4], reverse osmosis [Citation5, Citation6], ion exchange [Citation7, Citation8], evaporation and others [Citation9, Citation10]. Conventional methods characterized by several disadvantages such as incomplete metal removal especially at low concentrations, high reagent or energy requirements, generation of large amounts of toxic sludge and other hazardous by-products that must be additionally treated or eliminated. In general, these methods are not economically effective.
For this reason, many scientific researches are focused on the possibilities for development and application of cost-effective and environment friendly technologies for heavy metals removal from aqueous solutions. Among the biotechnological methods, bioaccumulation and biosorption demonstrate promising potential for replacing conventional methods [Citation11]. Bioaccumulation is a process that occurs only in living organisms through transport of contaminants and their accumulation into the cell, mainly microbial cells. The major restriction of this method is the lethal effect of heavy metal on cells and for this reason it is appropriate for application only at relatively lower metal concentration.
Biosorption is defined as a metabolically independent method for pollutants removal from aqueous solutions by adsorption of the pollutant onto biomass (mainly from plant or microbial origin), called biosorbent [Citation12–15]. The use of non-living cells offers the following advantages comparing to living biomaterials: no requirement for growth media and nutrients, no requirements for aseptic conditions, high efficiency, independence of pollutant toxicity, minimisation of chemical sludge, regeneration of biosorbent and possibility for metal recovery, higher speed of adsorption and low cost [Citation11, Citation16]. Currently biosorption studies are concentrated into several major directions as: (a) screening of low cost biosorbents with high biosorption capacity and mechanical stability, (b) optimization of process parameters and (c) modelling and scale of-up laboratory processes to industrial scale.
Various waste biomaterials from food, fermentation, pharmaceutical industries and agriculture were intensively studied for heavy metals removal from aqueous solutions [Citation14, Citation17, Citation18]. In their efforts to increase the sorption capacity of the biomass, authors usually focused on the application of different chemical methods for pretreatment and modification of chemical groups on the surface of the biosorbent. Despite that microbial biomass accounts for the dominant group of biosorbents, one of the major problems concerning microbial biosorbents is their mechanical instability and also the difficulty in separating the biosorbent from the aqueous solution at the end of the batch biosorption process [Citation19, Citation20].
To overcome these drawbacks, a lot of studies are directed to application of immobilized bacterial, yeast or fungal biosorbents for heavy metals removal [Citation19, Citation21]. Based on the results obtained, many authors conclude that microbial biomass can remove heavy metals from aqueous solutions and waste waters both in free and immobilized form and immobilization increases the mechanical strength of the biosorbent [Citation22–30]. There is no general conclusion unambiguously explaining the effect of immobilization on the heavy metals removal capacity of microbial sorbents. It is clear that the biosorption capacity of immobilized biosorbents depends on the type of microbial biomass, the carrier and the immobilization technique applied, and it is impossible to formulate general rules for selection of the most effective immobilization procedure. For this reason the most appropriate immobilization method and optimal process conditions for reaching the maximum removal of heavy metals by immobilized microbial biosorbents must be determined individually for each pair of biosorbent–heavy metal.
The aim of the present study was to construct a novel effective biocomposite sorbent for Pb(II), Cd(II) and Hg(II) removal from aqueous solutions based on the combination of waste bacterial biomass co-immobilized with inorganic carriers into a polymer gel. The major parameters influencing the batch biosorption process, such as pH, biosorbent dosage and initial metal ion concentrations, were also optimized.
Materials and methods
Preparation of free bisorbent of Bacillus cereus
Waste biomass of B. cereus used for biotechnological production of microbial phospholipase in laboratory conditions was separated from culture broth by filtration and was washed three times with distilled water. The washed biomass was dried at 80 °C for 12 h. The heat inactivated biomass was ground in a laboratory grinder and sieved until particles of uniform size were reached and in this form it was used as control sample free bisorbent called B.c/FB. The biosorbent was stored at 4 °C until biosorption.
Preparation of composite biosorbents of B. cereus by immobilization
Immobilization of B. cereus in calcium alginate beads
Sodium alginate was dissolved in hot distilled water at concentration of 2% (w/v). Cell suspension of heat inactivated biomass of B. cereus was mixed with sodium alginate solution at ratio 1:1 on a magnetic stirrer until complete homogenisation. Then using the syringe-method, the suspension was extruded dropwise to a gelling solution of 0.1 mol/L CaCl2, previously cooled to 4 °C. The beads were left to polymerise for 30 min and then were separated from the solution by filtration using Bűchner funnel and washed twice with distilled H2O. The beads called biosorbent type B.c/ALG were stored in distilled H2O at 4 °C until biosorption.
Co-immobilization of B. cereus in calcium alginate beads with activated carbon
Activated carbon was added to sodium alginate solution to reach final concentration of 3% (w/v). Then the cell suspension of heat inactivated biomass of B. cereus was mixed with sodium alginate–activated carbon suspension and the above listed procedure for beads formation by syringe-method was followed. The beads of co-immobilized B. cereus with activated carbon in calcium alginate, called biosorbent type B.c/AC/ALG, were stored in distilled H2O at 4 °C until biosorption.
Co-immobilization of B. cereus in calcium alginate beads with bentonite
About 6% (w/v) bentonite clay was added to sodium alginate solution to reach final concentration of 3% (w/v). Then the cell suspension of heat inactivated biomass of B. cereus was mixed with sodium alginate–bentonite suspension and the above listed procedure for beads formation by syringe-method was followed. The beads of co-immobilized B. cereus with bentonite in calcium alginate called biosorbent type B.c/B/ALG were stored in distilled H2O at 4 °C until biosorption.
Preparation of heavy metals stock solutions
Stock solutions of studied metal ions Pb(II), Cd(II) and Hg(II) at initial concentration 1000 mg/L were prepared by dissolving a weighed quantity of Pb(NO3)2 (Aldrich), Hg(NO3)2·H2O (Aldrich) and Cd(NO3)2·4H2O (Aldrich) in deionized water. Working solutions of different concentrations 10, 25, 50, 75 and 100 mg/L of each heavy metal were prepared as single solutions by adequate dilution of the initial stock solution.
Biosorption studies
To select an appropriate composite sorbent with high removal capacity, we carried out batch biosorption experiments in 250 mL Erlenmeyer flasks containing 100 mL single working solution of a desired metal ion, at 25 °C on a rotary shaker (120 rpm) for 120 min at pH 5.0 and biosorbent dosage of 2 g/L. At the end of the process, the biosorbent was separated by filtration, and the residual metal ion concentration in the solution was determined by atomic absorption spectroscopy (AAS) using an atomic absorption spectrometer PinAAcle 900 T (THGA/FLAME) Perkin Elmer.
The effect of pH on the removal of heavy metals was studied in the range of 3.0–6.0. The desired pH value was adjusted with 0.1 mol/L HNO3 or 0.1 mol/L NaOH. The effect of biosorbent dosage on the heavy metals removal was studied in the range of 0.5–3.0 g/L. The effect of initial metal ion concentration on the heavy metals removal was studied at 10, 25, 50, 75 and 100 mg/L.
Desorption experiments
Desorption experiments were carried out by 0.1 mol/L NaCl in five consecutive adsorption–desorption cycles and the heavy metal removal capacity of the biosorbent was monitored after each adsorption–desorption cycle. All glassware used in the biosorption experiments were washed with 10% (v/v) HNO3 and subsequently rinsed with deionized water to avoid binding of the metal ions to it. All the experiments were carried out in triplicate.
Calculation of metal uptake and removal efficiency
The heavy metal uptake was calculated by the simple difference method using Equation (1):
(1)
(1)
(1),where q is metal uptake, mg/g; Ci and Cf are the initial and final concentrations of metal ion, mg/L; V is the volume of working solutions, L; W is the biosorbent dosage, g.
The removal of metal ions was calculated using Equation (2):
(2)
(2)
where R is the removal efficiency, %; Ci and Cf are the initial and final concentrations of metal ion, mg/L
Equilibrium modelling
The Langmuir and Freundlich models were used to describe the biosorption equilibrium [Citation14, Citation31]. The linearized Langmuir isotherm allows the calculation of the maximum adsorption capacity and Langmuir constant and was presented by the following Equation (3):
(3)
(3)
where qe and qm are the metal uptake at equilibrium and the maximum metal uptake respectively, mg/g; Ce is metal concentration at equilibrium, mg/L, and b is the constant related to the affinity of the binding sites, L/mg.
The Freundlich isotherm was linearized as follows and Freundlich constants were determined:
(4)
(4)
where KF and n (dimensionless) are Freundlich adsorbent constant and exponent characterising the system.
Results and discussion
Selection of prospective composite biosorbent for Pb(II), Cd(II) and Hg(II) removal from aqueous solutions
As it was mentioned above, one of the major disadvantages of microbial biosorbents is their mechanical instability and also it is difficult to separate the biosorbent from aqueous solution at the end of the biosorption process. To overcome these drawbacks we focused our efforts on the possibilities for construction of novel type of immobilized microbial biosorbent for heavy metals removal from aqueous solutions with high removal capacity and sufficient mechanical stability. Calcium alginate is the dominant natural carrier for immobilization by entrapment due to its high biocompatibility and simple gelatinisation [Citation32–35]. Immobilization with natural polymeric carriers leads to the formation of gel beads, a major drawback being diffusion restrictions [Citation19, Citation36]. To solve this problem we tried to combine waste bacterial biomass of B. cereus as a cheap biosorbent material with another more rigid and stable inorganic sorbent as activated carbon or bentonite co-immobilized into polymer gel. In this way we hope to increase removal capacity of the microbial biosorbent and to enhance its mechanical stability and to benefit the sorbent separation after the biosorption. Three types of immobilized biosorbents were constructed B.c/ALG, B.c/AC/ALG and B.c/B/ALG and were tested for Pb(II), Cd(II) and Hg(II) removal from aqueous solutions. The results are shown in .
Figure 1. Removal of Pb(II), Cd(II) and Hg(II) from aqueous solutions by different composite biosorbents.
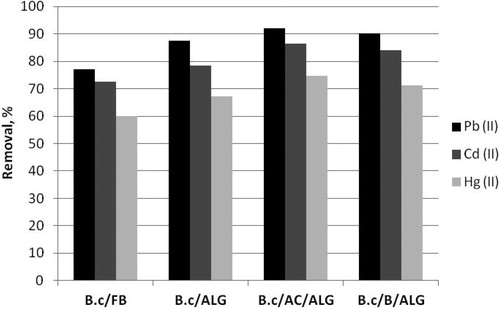
As seen, the three types of composite biosorbents B.c/ALG, B.c/AC/ALG and B.c/B/ALG demonstrated increased removal capacity in comparison with free biosorbent B.c/FB. Microbial biomass, co-immobilization with carriers and a combination of inorganic and organic materials, whose joint performance is complementary, renders composite biosorbent with superior biosorption properties. The co-immobilization of heat inactivated biomass of B. cereus with activated carbon in calcium alginate causes the highest removal increasing of 19% in comparison with free biosorbent, followed by co-immobilization with bentonite which causes about 16% increasing. The constructed biosorbents are the most appropriate for Pb(II) removal from aqueous solutions and maximum removal efficiency of 92.13% was reached, followed by Cd(II) removal 83.15% and Hg(II) removal 74.6%. The highest biosorption affinity of Pb(II) probably is due to the biggest ionic radius and the smallest hydration capacity in comparison with Cd(II) and Hg(II) resulting in the weaker binding between Pb(II) and water phase.
Based on the obtained results, we selected the composite biosorbent B.c/AC/ALG as the most prospective among the three studied types of biosorbents and all subsequent experiments were carried out with B.c/AC/ALG. Our results are in accordance with Chatterjee and Ray [Citation37] and Paul et al. [Citation38], who also achieved increased biosorption capacity of B. cereus for Cu(II) and Pb(II) removal by immobilization in calcium alginate.
Optimization of process parameters for Pb(II), Cd(II) and Hg(II) removal from aqueous solutions by composite biosorbent B.c/AC/ALG
Effect of pH on Pb(II), Cd(II) and Hg(II) removal from aqueous solutions by composite biosorbent B.c/AC/ALG
The pH of aqueous solution is one of the most important parameters influencing the biosorption processes. It affects the behaviour and speciation of metal ions; the dissociation of functional groups of the biosorbent and the metal ion-sorbent interactions [Citation14, Citation39–41]. The effect of initial pH on the Cd(II) and Hg(II) removal by selected composite biosorbent B.c/AC/ALG was evaluated in the range from 3.0 to 6.0. The effect of pH on Pb(II) removal by the selected composite biosorbent B.c/AC/ALG was evaluated in the pH range from 3.0 to 5.0 to avoid Pb(OH)2 precipitation, because at pH values higher than 5.0 several low soluble hydroxide species were observed [Citation42]. The results are shown in .
Figure 2. Effect of pH on the Pb(II), Cd(II) and Hg(II) removal from aqueous solutions by composite biosorbent B.c/AC/ALG.
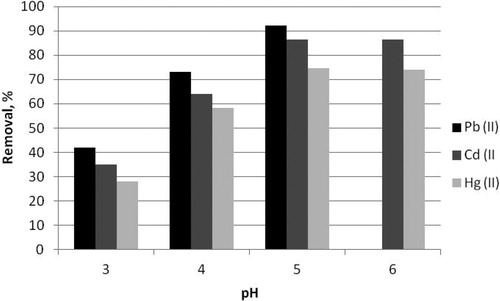
As seen, the removal efficiency of composite biosorbent B.c/AC/ALG increased from 41.81% to 92.13% for Pb(II), from 35.02% to 86.33% for Cd(II) and from 28.03% to 74.6% for Hg(II) with the increasing of pH from 3.0 to 5.0. The greatest metal uptake, 11.52 mg/g for Pb(II), 10.79 mg/g for Cd(II) and 9.33 mg/g for Hg(II), was reached at pH 5.0. Therefore, pH 5.0 was selected as the most appropriate pH value for all further biosorption experiments.
The increase in pH causes deprotonation of functional groups on the biosorbent and thus, formed negatively charged sites for electrostatic attraction of positively charged metal ions. The low removal efficiency at low pH was due to the presence of higher concentrations of hydrogen ions in the solution, which competed with heavy metal ions for the adsorption sites on the biosorbent surface. These results were in a good agreement with data for the effect of pH on Pb(II) biosorption published by other authors [Citation24–26, Citation38].
Effect of biosorbent dosage on Pb(II), Cd(II) and Hg(II) removal from aqueous solutions by composite biosorbent B.c/AC/ALG
Another key factor influencing the effectiveness of biosorption is the adsorbent dosage. Usually at low biosorbent dosages higher biosorption capacity and low heavy metal removal are detected but at higher sorbent amount some diffusion limitations are possible, which also affects biosorption effectiveness [Citation43, Citation44]. To clarify these effects different dosages of the selected composite biosorbent B.c/AC/ALG, ranging from 0.5 to 3.0 g/L, was used for Pb(II), Cd(II) and Hg(II) removal. The results are shown in .
Figure 3. Effect of biosorbent dosage on the Pb(II), Cd(II) and Hg(II) removal from aqueous solutions by composite biosorbent B.c/AC/ALG.
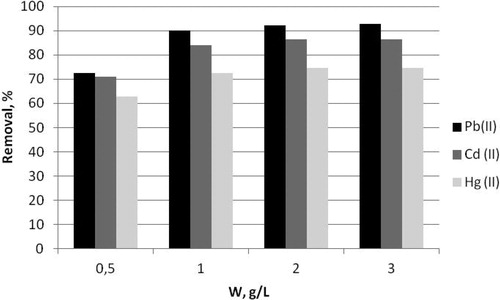
The increase of biosorbent dosage from 0.5 to 3.0 g/L caused a decrease in metal uptake as follows: for Pb(II) from 36.21 mg/g to 7.73 mg/g, for Cd(II) from 35.43 mg/g to 8.04 mg/g and for Hg(II) from 31.36 mg/g to 6.23 mg/g. The decreased metal uptake was associated with increased removal capacity, reaching 92.86% removal for Pb(II), 86.41% removal for Cd(II) and 74.72% removal for Hg(II). Maximum removal capacity was detected at the highest biosorbent dosage and 28.2%, 21.9% and 19% increasing was reached for Pb(II), Cd(II) and Hg(II) removal, respectively. Initial increase of biosorbent dosage from 0.5 g/L to 1 g/L resulted in a well expressed increase in the removal effectiveness by 24%, which was due to the increased number of active sites for metals ions binding on the biosorbent surface. Additional amounts of the bisorbent reaching 2 g/L resulted in a slight increase in Pb(II) removal by only 2% and additional increase with 1 g/L reaching biosorbent dosage 3 g/L caused less than 1% increase in Pb(II) removal. The same trends were detected for Cd(II) and Hg(II) removal. Probably at large amounts of the biosorbent, it is almost impossible for heavy metal ions to occupy all of the binding sites located on the biosorbent surface; and some diffusion limitations are possible, which decreases the biosorption effectiveness. These results are in accordance with literature data [Citation24]. Based on the results obtained, we selected biosorbent dosage of 2 g/L as the most appropriate for all further biosorption experiments.
Effect of initial metal ion concentration on Pb(II), Cd(II) and Hg(II) removal from aqueous solutions by composite biosorbent B.c/AC/ALG
The effectiveness of biosorption depends not only on the type and dosage of the biosorbent and pH of the solution but also on the initial metal ion concentration. This process parameter is the driving force for overcoming the transmission resistances between solution and sorbate phases. The effect of initial metal ion concentrations ranging from 10 g/L to 100 g/L on Pb(II), Cd(II) and Hg(II) removal from aqueous solutions by composite biosorbent B.c/AC/ALG were studied. The results are shown in (a)–(c).
Figure 4. Effect of initial metal ion concentration on the Pb(II) removal (a), Cd(II) removal (b) and Hg(II) removal (c) from aqueous solutions by composite biosorbent B.c/AC/ALG.
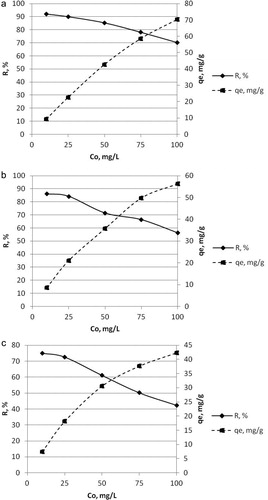
As the initial metal concentration increased, the metal ion uptake by the biosorbent increased but the removal efficiency decreased. This could be attributed to the gradual blocking of the free active sites for metal ion binding on the surface of the biosorbent.
Desorption experiments
The general effectiveness of heavy metal removal from aqueous solutions depends not only on the sorption capacity of the biosorbent and process parameters of biosorption stage but also on the desorption process and the possibilities for biosorbent regeneration. Usually solutions of diluted mineral acids (HCl, H2SO4 and HNO3), organic acids (citric, acetic and lactic acids) and EDTA are used for desorption of metal ions.
To evaluate the mechanical stability and the possibilities for re-usage of the composite biosorbent B.c/AC/ALG, five consecutive adsorption–desorption cycles were carried out. The residual capacity for heavy metal removal by the biosorbent after each working cycle was monitored. The results are shown in .
Figure 5. Effect of desorption on the Pb(II), Cd(II) and Hg(II) removal from aqueous solutions by composite biosorbent B.c/AC/ALG (a) and control sample free biosorbent B.c/FB (b).
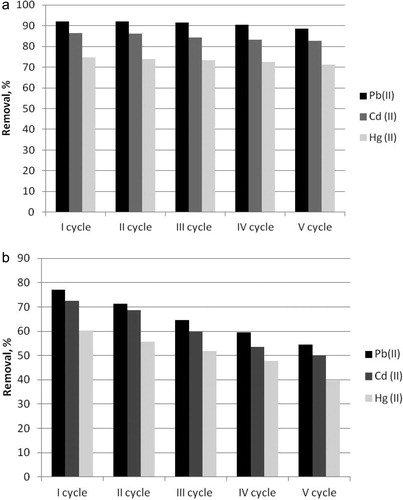
After five working cycles, the composite biosorbent B.c/AC/ALG retained its removal capacity almost unchanged (). The removal effectiveness of the biosorbent B.c/AC/ALG for the studied heavy metal removal decreased less than 5% (3.8% for Pb(II) removal, 4.2% for Cd(II) removal and 4.5% for Hg(II) removal). The removal capacity of the free biosorbent B.c/FB used as control sample decreased about 15% () even after the third adsorption–desorption cycle and about 29.3% for Pb(II) removal, 31.2% for Cd(II) removal and 34.1% for Hg(II) removal after the fifth cycle, indicating that co-immobilization with activated carbon in alginate gel increased the mechanical stability of the free biosorbent.
Equilibrium modelling of biosorption process for Pb(II), cd(II) and Hg(II) removal from aqueous solutions by composite biosorbent B.c/AC/ALG
Equilibrium modelling is an obligate stage in biosorption studies because it allows characterisation and comparisons among different biosorbents at different process conditions and also is a prerequisite for designing and scale-up of the removal processes into industrial scale. Usually the Langmuir and Freundlich isotherm equations are used for modelling of biosorption from single ion solution. The major advantages of these equations are the limited number of experimentally determined constants and their easier interpretation. The Langmuir model describes the adsorption process as a reversible equilibrium process, carried out on the independent active binding sites, which are homogeneously distributed on the surface of the sorbate and only one molecule binds into each site. In general, the Langmuir model describes homogeneous adsorption, whereas, the Freundlich model describes a process which is carried out on the heterogeneous sorbate. The maximum biosorption capacity and constant values for Pb(II), Cd(II) and Hg(II) removal from aqueous solutions by composite biosorbent B.c/AC/ALG were calculated by the linearized isotherms of Langmuir and Freundlich and the results are shown in .
Table 1. Langmuir and Freundlich constants.
The Langmuir model better fitted the experimental data for Pb(II), Cd(II) and Hg(II) removal from aqueous solutions by composite biosorbent B.c/AC/ALG. Based on the results obtained it became clear that the constructed biosorbent B.c/AC/ALG is appropriate predominantly for removal of Pb(II) from aqueous solutions. The maximum biosorption capacity of B.c/AC/ALG calculated by Langmuir equation was compared with the maximum biosorption capacity of other biosorbents based on bacteria belonging to genus Bacillus. The comparisons are shown in .
Table 2. Biosorption of Pb(II) onto bacterial sorbents belonging to genus Bacillus.
As seen, the calculated maximum biosorption capacity dramatically changed in a large scale, ranging from 0.33 mg/g to 131.84 mg/g, depending on the bacterial species, the applied methods for preliminary pretreatment of biomass, the type of cells, living or inactivated, and the process conditions. It is almost impossible to compare different biosorbents only based on the maximum biosorption capacity. Nevertheless, the novel composite biosorbent B.c/AC/ALG is competitive, because it demonstrated the highest removal capacity, except that of the biosorbent of Bacillus licheniformis. However, the procedure for preparation of the B. licheniformis biosorbent is more complicated in comparison with heat inactivation and direct immobilization in alginate gel, which was applied in a present study.
Conclusions
A novel biocomposite consisting of waste biomass from B. cereus co-immobilized with activated carbon into alginate was successfully applied for biosorption removal of Pb(II), Cd(II) and Hg(II) from aqueous solutions. The biocomposite was characterized with increased heavy metals removal capacity in comparison with free microbial biosorbent. Major process parameters were optimized and a maximum removal efficiency of 92.13% was reached for Pb(II) ions at pH 5.0, biosorbent dosage 2 g/L, temperature 25 °C and agitation speed 120 rpm for 120 min. The biocomposite demonstrated the highest affinity to Pb(II) ions followed by Cd(II) and Hg(II). Langmuir equilibrium model better fitted the biosorption process. Further experiments will be focused on the possibilities for application of the constructed biocomposite for removal of heavy metals in a column.
Disclosure statement
No potential conflict of interest was reported by the authors.
Funding
Dr. Kostadinka Todorova was supported by National Scientific Programme for Young Scientists and Postdoctoral Fellows, Bulgarian Ministry of Education and Science. This work was also supported by “P. Hilendarski” University of Plovdiv under grant number FP17/FK002.
References
- Ajmal M, Khan A, Nomani AA, et al. Heavy metals: leaching from glazed surfaces of tea mugs. Sci Total Environ. 1997;207:49–54.
- González-Muñoz MJ, Rodríguez MA, Luque S, et al. Recovery of heavy metals from metal industry waste waters by chemical precipitation and nanofiltration. Desalination. 2006;200:742–744.
- Macchi G, Marani D, Pagano M, et al. A bench study on lead removal from battery manufacturing wastewaters by carbonate precipitation. Water Res. 1996;30:3032–3036.
- Hunsom M, Pruksathorn K, Damronglerd S, et al. Electrochemical treatment of heavy metals (Cu2+, Cr6+, Ni2+) from industrial effluent and modeling of copper reduction. Water Res. 2005;39:610–616.
- Atkinson S. World’s largest desalination plant begins operating in Israel. Membr Technol. 2005;2005:9–10.
- Gholani MM, Mokhtari MA, Aameri A, et al. Application of reverse osmosis technology for arsenic removal from drinking water. Desalination. 2006;200:725–727.
- Ćurković L, Cerjan-Stefanović Š, Filipan T. Metal ion exchange by natural and modified zeolites. Water Res. 1997;31:1379–1382.
- Eom TH, Lee CH, Kim JH, et al. Development of an ion exchange system for plating wastewater treatment. Desalination. 2005;180:163–172.
- Djukić A, Jovanović U, Tuvić T, et al. The potential of ball-milled Serbian natural clay for removal of heavy metal contaminants from wastewaters: simultaneous sorption of Ni, Cr, Cd and Pb ions. Ceram Int. 2013;39:7173–7178.
- Shavandi MA, Haddadian Z, Ismail MHS, et al. Removal of Fe(III), Mn(II) and Zn(II) from palm oil mill effluent (POME) by natural zeolite. J Taiwan Inst Chem Eng. 2012;43:750–759.
- Vijayaraghavan K, Yun YS. Bacterial biosorbents and biosorption. Biotechnol Adv. 2008;26:266–291.
- Gadd GM. Biosorption: critical review of scientific rationale, environmental importance and significance for pollution treatment. J Chem Technol Biotechnol. 2009;84:13–28.
- Hansda A, Kumar V. Anshumali A comparative review towards potential of microbial cells for heavy metal removal with emphasis on biosorption and bioaccumulation. World J Microbiol Biotechnol. 2016;32:170.
- Volesky B. Sorption and biosorption. Montreal (QC): BV-Sorbex Inc.; 2003. p. 97–100.
- Fomina M, Gadd GM. Biosorption: current perspectives on concept, definition and application. Bioresour Technol. 2014;160:3–14.
- Modak JM, Natarajan KA. Biosorption of metals using nonliving biomass – a review. Miner Metall Proc. 1995;12:189–196.
- Wang J, Chen C. Biosorbents for heavy metals removal and their future. Biotechnol Adv. 2009;27:195–226.
- Park D, Yun AS, Park JM. The past, present, and future trends in biosorption. Biotechnol Bioproc Eng. 2010;15:86–102.
- Ramrakhiani L, Ghosh S, Majumdar S. Surface modification of naturally available biomass for enhancement of heavy metal removal efficiency, upscaling prospects, and management aspects of spent biosorbents: a review. Appl Biochem Biotechnol. 2016;180:41–78.
- Veglio F, Beolchini F. Removal of metals by biosorption: a review. Hydrometallurgy. 1997;44:301–316.
- Andrès Y, Texier AC, Le Cloirec P. Rare earth elements removal by microbial biosorption: a review. Environ Technol. 2003;24:1367–1375.
- Li X, Wang Y, Li Y, et al. Biosorption behaviors of biosorbents based on microorganisms immobilized by Ca-alginate for removing lead(II) from aqueous solution. Biotechnol Bioproc Eng. 2011;16:808.
- Çabuk A, Akar T, Tunali S, et al. Biosorption characteristics of Bacillus sp. ATS-2 immobilized in silica gel for removal of Pb(II). J Hazard Mater. 2006;163:317–323.
- Wen X, Du C, Zeng G, et al. A novel biosorbent prepared by immobilized Bacillus lichemiformis for lead removal from wastewaters. Chemosphere. 2018;200:173–179.
- Xiangling P, Jianlong W, Daoyong Z. Biosorption of Pb(II) by Pleurotus ostreatus immobilized in calcium alginate gel. Process Biochem. 2005;40:2799–2803.
- Lin C, Lai Y-T. Adsorption and recovery of lead(II) from aqueous solutions by immobilized Pseudomonas aeruginosa PU21 beads. J Hazard Mater. 2006;137:99–105.
- Mata YN, Blazquez ML, Ballester A, et al. Biosorption of cadmium, lead and copper with calcium alginate xerogels and immobilized Fucus vesiculosus. J Hazard Mater. 2009;163:555–562.
- Tsekova K, Todorova D, Dencheva V, et al. Biosorption of copper(II) and cadmium(II) from aqueous solutions by free and immobilized biomass of Aspergillus niger. Bioresour Technol. 2010;101:1727–1731.
- Yan G, Viraraghavan T. Heavy metal removal in a biosorpetion column by immobilized Mucor rouxii biomass. Bioresour Technol. 2001;78:243–249.
- Arica YM, Bayramoglu G, Yilmaz M, et al. Biosorption of Hg2+, Cd2+ and Zn2+ by calcium alginate and immobilized wood-rotting fungus Funalia trogii. J Hazard Mater. 2004;109:191–199.
- Loukidou M, Zouboilis A, Karapantsios T, et al. Equilibrium and kinetic modeling of Cr(VI) biosorption by Aeromonas caviae. Colloids Surf A: Physicochem Eng Aspects. 2004;242:90–104.
- Bishnoi NR, Kumar R, Bishnoi K. Biosorption of Cr (VI) with Trichoderma viride immobilized fungal biomass and cell free Ca-alginate beads. Indian J Exp Biol. 2007;45:657–664.
- Tan WS, Ting A. Efficacy and reusability of alginate-immobilized live and heat-inactivated Trichoderma asperellum cells for Cu(II) removal from aqueous solution. Bioresour Technol. 2012;123:290–295.
- Ivánová D, Horváthová H, Kaduková J, et al. Stability of immobilized biosorbents and its influence on biosorption of copper. Nova Biotechnol. 2010;10:45–51.
- Es I, Vieira J, Amaral AC. Principles, techniques, and applications of biocatalyst immobilization for industrial application. Appl Microbiol Biotechnol. 2015;99:2065–2082.
- Górecka E, Jastrzębska M. Immobilization techniques and biopolymer carriers. Biotechnol Food Sci. 2011;75:65–86.
- Chatterjee A, Ray L. Biosorption of Cu(II) by immobilized biomass of Bacillus cereus M116 from aqueous solution. J Sci Ind Res. 2008;67:629–634.
- Paul S, Bera D, Chattopadhyay P, et al. Biosorption of Pb(II) by Bacillus cereus M116 Immobilized in Calcium Alginate Gel. J Hazard Subst Res. 2006;5:2.
- Esposito A, Pagnanelli F, Lodi A, et al. Biosorption of heavy metals by Sphaerotilus natans: an equilibrium study at different pH and biomass concentrations. Hydrometallurgy. 2001;60:129–141.
- Alkan H, Gul-Guven R, Guven K, et al. Biosorption of Cd2+, Cu2+, and Ni2+ ions by a thermophilic haloalkalitolerant bacterial strain (KG9) immobilized on amberlite XAD-4. Pol J Environ Stud. 2015;24:1903–1910.
- Alavi SA, Zilouei H, Asadinezhad A. Otostegia persica biomass as a new biosorbent for the removal of lead from aqueous solutions. Int J Environ Sci Technol. 2015;12:489–498.
- Ozdes D, Gundogdu A, Kemer B, et al. Removal of Pb(II) ions from aqueous solution by a waste mud from copper mine industry: equilibrium, kinetic and thermodynamic study. J Hazard Mater. 2009;166:1480–1487.
- Boschi C, Maldonado H, Ly M, et al. Cd(II) biosorption using Lessonia kelps. J Colloid Interface Sci. 2011;357:487–496.
- O’Connell DW, Birkinshaw C, O’Dwyer TF. Heavy metal adsorbents prepared from the modification of cellulose: a review. Bioresour Technol. 2008;99:6709–6724.
- Pan J, Liu R, Tang H. Surface reaction of Bacillus cereus biomass and its biosorption for lead and copper ions. J Environ Sci. 2007;19:403–408.
- Seo H, Lee M, Wang S. Equilibrium and kinetic studies of the biosorption of dissolved metals on Bacillus drentensis immobilized in biocarrier beads. Environ Eng Res. 2013;18:45–53.
- Oves M, Khan MS, Zaidi A. Biosorption of heavy metals by Bacillus thuringiensis strain OSM29 originating from industrial effluent contaminated north Indian soil. Saudi J Biol Sci. 2013;20:121–129.