Abstract
Ramie fibres are harvested from the stem bark of the plant, and thus two stem traits—the stem length (SL) and the stem diameter (SD)—are important components of fibre yield in this crop. A recently developed high-density genetic map using an F2 agamous line population derived from two parents, Qingdaye (QDY) and Zhongzhu 1 (ZZ1) laid the basis for dissection of the genetic basis of fibre yield-related traits. However, the genetic basis of the two fibre yield-related stem traits presented here is still uncharacterized in this population. In this study, quantitative trait locus (QTL) analysis for SL and SD traits was performed using the population of QDY/ZZ1, resulting in the identification of four SL and six SD QTLs. Among these 10 QTLs, one was detected in both environments, and six demonstrated a significant environmental correlation. Three DELLA genes were identified to cluster in the region of the stem length QTL qSL11b, and their encoding proteins displayed either amino acid-substitutions or amino acid-insertion/deletion in the functional GRAS domain between two parents. Because the DELLA protein is a negative regulator of the gibberellin signalling pathway and plays a central role in regulating the stem elongation, the mutations in the GRAS domain-encoding region of these three genes potentially influence stem growth. The identification of the QTLs for two stem traits will help improve the fibre yield in ramie breeding programmes by marker-assisted selection. The three identified DELLA genes are candidate genes for cloning the qSL11b locus in future studies.
Keywords:
Introduction
Ramie (Boehmeria nivea L. Gaud), a perennial and diploid (2n = 28) herbaceous plant, is the second most important natural fibre crop in China, and the growth acreage and production are only a little lower than cotton. Ramie fibres have many excellent traits, such as a smooth texture and high tensile strength [Citation1], and a long fibre length that can reach up to 55 cm [Citation2]. Ramie fibres are harvested from the stem; therefore, two stem traits—stem length (SL) and stem diameter (SD)—have a critical influence on fibre yield [Citation3,Citation4].
The pursuit of high fibre yield is a major goal in ramie breeding, so fibre yield-related traits, including stem length and diameter, have received much attention in previous studies. Liu et al. [Citation5,Citation6] have developed simple sequence repeat markers (SSRs) from the expressed sequence tags on a large scale, and constructed the first genetic linkage map using these SSRs. Based on this genetic map, the genetic basis of fibre yield traits and its four components have been characterized first, resulting in a total of 33 identified quantitative trait loci (QTLs) [Citation6]. There are 24 QTLs exhibiting overdominance, which are possibly responsible for the heterosis of these yield-related traits in ramie [Citation6]. Therefore, this study provides the first insights into the genetic basis of fibre yield-related traits in ramie. A recent genome-wide study also identified 16 loci associated with fibre yield-related traits, based on these SSRs [Citation7]. The identification of these QTLs provides an essential basis for the genetic improvement of fibre yield traits through marker-assisted selection in ramie.
In the past 10 years, next generation sequencing has rapidly developed, and provides a powerful and cost-effective tool for the high-throughput screening of single nucleotide polymorphisms (SNP) and the construction of high-density linkage maps [Citation8,Citation9]. For ramie, a genetic map with 4338 SNPs has been developed using the segregating population derived from two parents, Qingdaye (QDY) and Zhongzhu 1 (ZZ1), which is the first high-density genetic map of ramie [Citation10]. Four QTLs for fibre yield traits and eleven QTLs involved in fibre yield-related stem bark traits were detected [Citation10]. There was a gene with a 760-bp insertion that caused premature termination, thereby producing a protein that lacked part of the MYB domain, in the region of bark thickness QTL qBT4a in the parent ZZ1. Because of the vital role, MYB genes play in the regulation of secondary cellular walls biosynthesis in fibre [Citation11], these MYB genes were considered candidate genes for qBT4a [Citation10]. Liu et al. [Citation10] not only expand our knowledge of the genetic basis of fibre yield but also provide a first insight into the molecular mechanism of fibre formation in ramie. However, in this population, the genetic basis of two stem traits remains uncharacterized, although these traits are the main components for fibre yield in ramie.
In this study, the genetic dissection for two stem traits (SL and SD) was performed using the population reported by Liu et al. [Citation10]. The identification of QTLs involved in SL and SD traits will not only provide basic genetic understanding of these two traits but also present new genetic loci for the improvement of fibre yield in the molecular breeding of ramie.
Materials and methods
Experimental population and phenotypic measurements
A ramie F2 agamous line (FAL) population that consisted of 134 lines derived from two parents, QDY and ZZ1, has been developed by Liu et al. [Citation10]. The field experiment for this population and the two parents have been described in the previous study [Citation10]. In June 2015 and 2016, when more than two-thirds of the plant leaves had shattered, the phenotype of stem traits was investigated in the population. The SL was measured from the stem bottom to the shoot, and the SD was measured in the middle of the stem using a Vernier caliper.
Data analyses
A high-density genetic map with a total length of 1942.9 cM has been developed, consisting of 4338 high-quality SNPs [Citation10]. QTLs of the two traits were detected in 2015 and 2016 using composite interval mapping with Widows QTLs CARTOGRAPHER 2.5. The Window size was set at a 10 cM threshold significance level and was used to identify significant makers as cofactors. The experimental likelihood of odd (LOD) threshold significant level was determined by computing 1000 permutations (p < 0.05) using a permutation test programme in MapQTLs [Citation12]. The interaction of QTL with the environment was analyzed using QTLMapper 1.6 [Citation13], and the significant interaction was identified at the p-value of less than 0.05. Correlation analyses and variance of phenotypic traits were conducted using Origin 2018.
DNA extraction, sequencing and comparison
The DNA was extracted from the fresh leaves of two parents (ZZ1 and QDY) using a DNA extraction kit (Tiangen, China). Three DELLA protein-encoding genes were amplified from these two parents using a standard PCR protocol with gene-specific primers (Supplementary material Table S1). For sequencing, 8 μL PCR products corresponding to each parent were digested using 5 U ExoI (NEB) and 0.13 U shrimp alkaline phosphatase (Fermentas) and sequenced using a 3730xl DNA Analyzer (ABI, USA). Sequence contigs were assembled using SEQUENCHER 4.1.2 (Gene Codes Co.), and the assembled three gene cDNA sequences of the two parents were submitted to GenBank with the accession numbers MK696552, MK696553, MK696554, MK696555, MK696556 and MK696557. The sequence alignment of DNA and protein for these three genes was performed between ZZ1 and QDY using Clustal Omega and Muscle [Citation14]. The conserved protein-encoding domain was identified using the NCBI conserved domain database [Citation15].
Phylogenetic analysis
Of the species whose genomes have been sequenced, Morus notabilis has the closest genetic relationship with ramie. Therefore, all eight DELLA proteins of M. notabilis and five DELLA proteins of Arabidopsis sp. were collected from the PlantTFDB database (http://planttfdb.cbi.pku.edu.cn/). Additionally, one drought stress-responsive DELLA gene (Unigene19721) has been reported in ramie [Citation16]. Phylogenetic analysis was performed on these 14 DELLA proteins, with the three DELLA proteins identified in this study. Multiple sequence alignments of the full-length protein sequences were performed by the Clustal X (version 1.83) programme [Citation17]. An unrooted phylogenetic tree was constructed with MEGA 4.0 using the Neighbour-Joining (NJ) method and the bootstrap test carried out with 1000 replicates [Citation18].
Results and discussion
Population variation in stem traits
The two parents displayed a significant difference in the stem traits, and the stems of ZZ1 were longer and wider than those of QDY (). There was a large variation between both years in the population. A significant positive correlation was observed between SL and SD (p < 0.05). In addition, both stem traits showed a significant positive correlation with fibre yield (p < 0.05, r > 0.76; ), suggesting that these two traits have a significant influence on fibre yield.
Table 1. Descriptive statistics of the traits for parents and the FAL population.
QTL mapping of SL and SD traits
SL and SD are the two main components of fibre yield in ramie. A significant positive correlation between these two traits and fibre yield observed in this study indicate that fibre yield is highly determined by the SL and SD. Both the SL and SD are quantitative traits, suggesting that they are typically defined by many major and minor QTLs. Linkage mapping is a common tool for the genetic dissection of quantitative traits and a large number of QTLs involved in quantitative traits have been identified by this approach [Citation19–22]. However, only nine SL QTLs and six SD QTLs were identified in ramie [Citation6]. Additionally, based on the association mapping, there were respectively six and five loci detected to associate with SL and SD [Citation7]. All these QTLs were identified based on limited mapping markers, resulting in QTLs with a low-resolution interval. Recently, a high-density genetic map with 4338 SNPs was developed, and a total of 15 fibre yield-related QTLs were identified, one of which, qBT4a, confirmed its candidate genes [Citation10]. However, two stem traits remain uncharacterized using this high-density genetic map.
Therefore, the genetic basis of SL and SD was dissected in the present study using this high-density genetic map. LOD threshold values of the two traits ranged from 4.8 to 5.2, which were determined by computing 1000 permutations (p < 0.05) (). Based on these LOD threshold values, four and six QTLs, respectively, were identified for SL and SD trait (, ). Among these 10 QTLs, only one SL QTL (qSL11b) and one SD QTL (qSD1a) increased the trait value for the QDY allele and the other eight QTLs for the ZZ1 allele; one QTL, qSD2b, was detected in both years (). Two QTLs, qSL2 and qSD2b were identified in a pleiotropic region of the linkage group 2 (, ). The identification of these QTLs provide a basis for the improvement of stem traits by marker-assisted selection in ramie high yield-breeding programmes.
Figure 1. Quantitative trait loci (QTLs) for ramie SL and SD traits. The red line indicates the genome-wide significance LOD threshold, and the red arrow indicates the LOD peak of the QTLs.
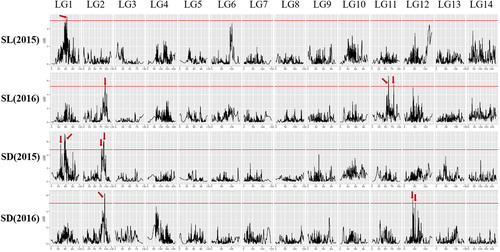
Table 2. QTLs identified for SL and SD from the population in 2015 and 2016.
The stem growth and fibre yield are easily impacted by climatic and environmental factors including precipitation, sunshine duration and the relative humidity [Citation23]. Short and thin stems are frequently observed when the ramie is subjected to environmental stress [Citation16,Citation24]. Therefore, we also examined the interaction of SL and SD QTLs with the environment (G × E), and the result revealed that six out of 10 QTLs were significantly correlated (p < 0.05; ). This result provides an insight into the genetic basis of the strong correlation between environmental conditions and the stem growth of ramie.
Identification and characterization of three DELLA genes in qSL11b
The SL QTL qSL11b was mapped into a 1.6 cM-region flanked by two markers, bin3128 and bin2862. After aligning these two markers into the ramie genome [Citation25,Citation26], the markers bin3128 and bin2862 were respectively mapped into the 424 kb and 1040 kb position in the scaffold PHNS01011683.1, suggesting that a 616-kb genome region is included in qSL11b. There were three genes annotated as DELLA protein-encoding genes in this QTL region, including whole_GLEAN_10018840, whole_GLEAN_10018848 and whole_GLEAN_10018850. Phylogenetic analysis revealed that these three genes were highly homologous with the Morus notabilis XP_024022283.1, XP_010097792.1, XP_010097793.1 and XP_010097794.1, especially the gene whole_GLEAN_10018850 ().
Figure 2. Phylogenetic tree of 17 DELLA proteins from ramie, Morus notabilis and Arabidopsis sp. The unrooted tree was generated using the neighbour-joining method from the MEGA 4 programme. Bootstrap values from 1000 replicates are indicated at each node.
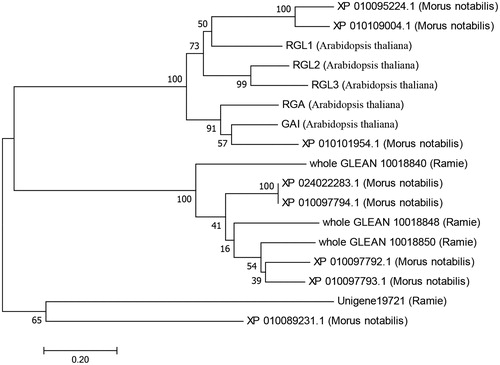
It is notable that gibberellins (GAs) are pleiotropic phytohormones, regulating various growth processes throughout the plant life cycle such as seed germination, leaf expansion, pollen maturation, the induction of flowering and especially stem elongation [Citation27,Citation28]. DELLA protein is a negative regulator of GA signalling pathways and can inhibit the growth of plants; the GAs signal can induce the destruction of DELLA protein and cancel the repressive function of DELLA [Citation29,Citation30]. DELLA proteins belong to a subfamily of GRAS, and their mutant forms result in a dwarf phenotype [Citation31]. In some crop breeding programmes, the dwarf phenotype caused by the mutation of DELLA genes was favoured because the dwarf lines can produce a higher yield and are more resistant to lodging. The application of these dwarfing alleles has resulted in the well-known ‘Green Revolution’ of crops. The DELLA genes that contributed to the ‘Green Revolution’ include wheat Rht, maize D8, rice slr1, barley sln1-d, Brassica rapa dwf2, and grape Vvgai1 [Citation32–36]. In ramie, GAs have been identified to dramatically promote the stem length and fibre yield [Citation37]. Transcriptome comparison between wild ramie and the cultivar found that GAs biosynthesis-related genes undergo significant positive selection [Citation38]. Also, a DELLA-encoding transcript unigene19721 with an up-regulated expression caused by a 335-fold under drought stress is, possibly responsible for the inhibition of the stem growth of ramie under drought stress [Citation16]. These studies indicate that GAs play an essential role in promoting growth of ramie.
Because of the vital role of DELLA proteins in stem growth, the three DELLA genes were further implemented for the sequence comparison between the two parents. Finally, 11, 42 and 11 SNPs causing respectively 7, 12 and 3 amino acid-substitutions were identified in whole_GLEAN_10018840e, whole_GLEAN_10018848 and whole_GLEAN_10018850e (). In addition, 3-bp and 9-bp insertion/deletion mutations were respectively identified in whole_GLEAN_10018848 and whole_GLEAN_10018850 (). Among these mutants, five, five and four amino acid differences appeared in the GRAS domain in the protein encoded by respectively whole_GLEAN_10018840, whole_GLEAN_10018848 and whole_GLEAN_10018850. Because the GRAS domain is a key functional domain involved in the transcriptional regulation, these mutants potentially have roles in regulating the stem elongation of ramie. Therefore, this study provides candidate genes of qSL11b for further validation in future studies.
Figure 3. Sequence difference of the three DELLA genes between the two parents. The white line in the black block indicates the SNP, the triangle represents the nucleotide insertion/deletion in the CDS gene of QDY by comparing that of ZZ1. The pink block indicates the gene region that encodes the functional GRAS domain. Two-row letters represent the amino acid change caused by sequence difference, and the red and blue letter indicates the amino acid sequence of respectively QDY and ZZ1. ‘-’ indicates the deletion of amino acid.
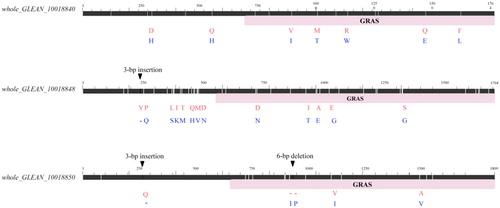
Conclusions
In this study, a total of four SL and six SD QTLs were detected using a high-density genetic map. Among these 10 QTLs, one was detected in both environments, and six showed a significant correlation with the environment. Additionally, three DELLA genes were identified to cluster in the region of stem length QTL qSL11b, and their encoding proteins displayed either amino acid-substitutions or amino acid-insertion/deletion in the functional GRAS domain in the two parents. Because the DELLA protein is a negative regulator of the gibberellin signalling pathway and plays a central role in regulating the stem elongation, the mutations in the GRAS domain-encoding region of these three genes potentially influence stem growth. The identification of the 10 stem trait QTLs and three DELLA genes will help improve the fibre yield in ramie breeding programmes by marker-assisted selection.
Supplemental Material
Download PDF (479.4 KB)Acknowledgements
We kindly acknowledge Dr. Touming Liu (Department of Southern Forage Crop and Utilization, Institute of Bast Fiber Crops, Chinese Academy of Agricultural Sciences) for providing the population for trait investigation and the genotypes used in this study.
Disclosure statement
No potential conflict of interest was reported by the authors.
Additional information
Funding
References
- Xiong H. Bast-fiber crops breeding. Beijing (China): China Agricultural Science and Technology Press; 2008.
- Aldaba VC. The structure and development of the cell wall in plants I. bast fibers of Boehmeria and Linum. Am J Bot. 1927;14:16–24.
- Liu LJ, Chen HQ, Dai X, et al. Effect of planting density and fertilizer application on fiber yield of ramie (Boehmeria nivea). J Integr Agric. 2012;11:1199–1206.
- Xiong H, Jiang J, Yu C, et al. Relations between yield-related traits and yield in ramie. Acta Agronomica Sinica. 1998;24:155–160.
- Liu T, Zhu S, Fu L, et al. Development and characterization of 1,827 expressed sequence tag-derived simple sequence repeat markers for ramie (Boehmeria nivea L. Gaud). PLoS One. 2013;8:e60346. [cited 2019 Apr 13]
- Liu T, Zhu S, Tang Q, et al. QTL mapping for fiber yield-related traits by constructing the first genetic linkage map in ramie (Boehmeria nivea L. Gaud). Mol Breeding. 2014;34:883–892.
- Luan MB, Liu CC, Wang XF, et al. SSR markers associated with fiber yield traits in ramie (Boehmeria nivea L. Gaudich). Ind Crops Prod. 2017;107:439–445.
- Verma S, Gupta S, Bandhiwal N, et al. High-density linkage map construction and mapping of seed trait QTLs in chickpea (Cicer arietinum L.) using genotyping-by-sequencing (GBS). Sci Rep. 2015;5:17512.
- Branham S, Levi A, Farnham M, et al. A GBS-SNP-based linkage map and quantitative trait loci (QTL) associated with resistance to Fusarium oxysporumf. sp. Niveum race 2 identified in Citrullus lanatusvar. citroides. Theor Appl Genet. 2017;130:319–330.
- Liu C, Zhu S, Tang S, et al. QTL analysis of four main stem bark traits using a GBS-SNP-based high-density genetic map in ramie. Sci Rep. 2017;7:13458.
- Zhong R, Ye Z. Secondary cell walls: biosynthesis, patterned deposition and transcriptional regulation. Plant Cell Physiol. 2015;56:195–214.
- Van OJ, Boer M, Jansen R, et al. MapQTL 4.0: software for the calculation of QTL positions on genetic maps [User manual]. Wageningen (Netherlands): Plant Research International; 2000.
- Wang D, Zhu J, Li Z, et al. Mapping QTLs with epistatic effects and QTL × environment interactions by mixed linear model approaches. Theor Appl Genet. 1999;99:1255–1264.
- Li W, Cowley A, Uludag M, et al. The EMBL-EBI bioinformatics web and programmatic tools framework. Nucleic Acids Res. 2015;43:580–584.
- Marchler BA, Lu S, Anderson JB, et al. CDD: a conserved domain database for the functional annotation of proteins. Nucleic Acids Res. 2011;39:D225–D229.
- Liu T, Zhu S, Tang Q, et al. Identification of drought stress-responsive transcription factor in ramie (Boehmeria nivea L. Gaud). BMC Plant Biol. 2013;13:130. 10.1186/1471-2229-13-130
- Thompson JD, Gibson TJ, Plewniak F, et al. The CLUSTAL_X windows interface: flexible strategies for multiple sequence alignment aided by quality analysis tools. Nucleic Acids Res. 1997;25:4876–4882.
- Tamura K, Dudley J, Nei M, et al. MEGA4: molecular evolutionary genetics analysis (MEGA) software version 4.0. Mol Biol Evol. 2007;24:1596–1599.
- Liu T, Zhang Y, Xue W, et al. Comparison of quantitative trait loci for 1,000-grain weight and spikelets per panicle across three connected rice populations. Euphytica. 2010;175:383–394.
- Liu T, Li L, Zhang Y, et al. Comparison of quantitative trait loci for rice yield, panicle length and spikelet density across three connected populations. J Genet. 2011;90:377–382.
- Mao D, Liu T, Xu C, et al. Epistasis and complementary gene action adequately account for the genetic bases of transgressive segregation of kilo-grain weight in rice. Euphytica. 2011;180:261–271.
- Zhu S, Zheng X, Dai Q, et al. Identification of quantitative trait loci for flowering time traits in ramie (Boehmeria nivea L. Gaud). Euphytica. 2016;210:367–374.
- Liu T, Tang Q, Zhu S, et al. Analysis of climatic factors causing yield difference in ramie among different eco-regions of Yalley valley. Agri Sci Technol. 2011;12:745–750.
- Liu T, Zhu S, Tang Q, et al. Genome-wide transcriptomic profiling of ramie (Boehmeria nivea L. Gaud) in response to cadmium stress. Gene. 2015;558:131–137.
- Liu C, Zeng L, Zhu S, et al. Draft genome analysis provides insights into the fiber yield, crude protein biosynthesis, and vegetative growth of domesticated ramie (Boehmerianivea L. Gaud). DNA Res. 2018;25:173–181.
- Luan M, Jian J, Chen P, et al. Draft genome sequence of ramie, Boehmeria nivea (L.) Gaudich. Mol Ecol Resour. 2018;18:639–645.
- Lange MP, Lange T. Gibberellin biosynthesis and the regulation of plant development. Plant Biol. 2006;8:281–290.
- Fleet CM, Sun TP. A DELLAcate balance: the role of gibberellin in plant morphogenesis. Curr Opin Plant Biol. 2005;8:77–85.
- Sun TP. The molecular mechanism and evolution of the GA-GID1-DELLA signaling module in plants. Curr Biol. 2011;21:R338–345.
- Hauvermale A, Ariizumi T, Steber C. Gibberellin signaling: a theme and variations on DELLA repression. Plant Physiol. 2012;160:83–92.
- Thomas S, Sun T. Update on gibberellin signaling. A tale of the tall and the short. Plant Physiol. 2004;135:668–676.
- Peng J, Richards DE, Hartley NM, et al. ‘Green revolution’ genes encode mutant gibberellin response modulators. Nature. 1999;400:256–261.
- Ikeda A, Ueguchi TM, Sonoda Y, et al. Slender rice, a constitutive gibberellins response mutant, is caused by a null mutation of the SLR1 gene, an ortholog of the height-regulating gene GAI/RGA/RHT/D8. Plant Cell. 2001;13:999–1010.
- Boss PK, Thomas MR. Association of dwarfism and floral induction with a grape ‘green revolution’ mutation. Nature. 2002;416:847–850.
- Chandler PM, Marion PA, Ellis M, et al. Mutants at the slender1 locus of barley cv Himalaya: molecular and physiological characterization. Plant Physiol. 2002;129:181–190.
- Muangprom A, Osborn TC. Characterization of a dwarf gene in Brassica rapa, including the identification of a candidate gene. Theor Appl Genet. 2004;108:1378–1384.
- Liu T, Zhu S, Fu L, et al. Morphological and physiological changes of ramie (Boehmeria nivea L. Gaud) in response to drought stress and GA3 treatment. Russ J Plant Physiol. 2013;60:749–755.
- Liu T, Tang S, Zhu S, et al. Transcriptome comparison reveals the patterns of selection in domesticated and wild ramie (Boehmeria nivea L. Gaud)). Plant Mol Biol. 2014;86:85–92.