Abstract
Today people focus on healthy lifestyle. It is well known that probiotics have a number of beneficial health effects in humans. They play an important role in protecting the host against harmful microorganisms, reduce metabolic disorders and support immune functions. In the present study, we investigated 26 probiotic products: 16 commercially available ones and 10 from a local manufacturer. None of the commercial products contained all labelled LAB and some of them contained unacceptable microorganisms. Of 890 isolated strains only 420 met the criteria for LAB. Ninety-seven strains were investigated by phenotypic and genotypic methods. 16S–23S rDNA amplification was performed for all putative LAB isolates. Fifty-seven rod-shaped bacteria referred to genus Lactobacillus and 16 coccus bacteria, to genus Weissela. Two-step multiplex PCR identified the rod-shaped strains to belong to four species: Lactobacillus delbrueckii ssp. bulgaricus (three strains), Lactobacillus acidophilus (four strains), Lactobacillus casei (three strains), Lactobacillus rhamnosus (two strains), Lactobacillus reuteri (four strains) and Lactobacillus plantarum (one strain). The transit tolerance of the isolated bacteria to in vitro simulated gastric juice (pH 2) was examined. Only four strains survived after 90 min of incubation. The antimicrobial activity of the native supernatants of the isolates was tested and 10 of them showed slight activity against three pathogenic bacteria. Our results demonstrated that only freshly produced products possessed the expected number of viable cells. The data revealed that one of the most common problems is the lower concentration of viable cells, misidentification and the presence of undesired microflora.
Introduction
Environmental deterioration, stress and eating disorders led to the development of the concept ‘probiotics and food products’ in the 1990s [Citation1]. Initially, the term ‘probiotic’ used to refer to a product based on lactic acid bacteria belonging to the intestinal commensals which are capable of implementing biological control in the body and have regulatory properties [Citation2]. According to the current international FAO/WHO definition, the human origin of the bacterial strain is not a criterion for selection of probiotics and, instead, importance is put on the type of effect caused [Citation3]. According to recommendations, there should be minimum 106 CFU mL−1 of live probiotic bacteria at the time of consumption [Citation4]. Research has since shown that probiotics (108–109 CFU mL−1) can regulate gut microbiota, improve immune system, reduce symptoms of lactose intolerance as well as prevent and/or treat gastrointestinal infections. Other health claims include the prevention of mammary cancer, the reduction of viral-associated pulmonary damage and the decrease in cholesterol level, which reduces the risk of cardiovascular diseases [Citation5]. The biological effect of probiotics is primarily due to antagonistic activity associated with the synthesis of lactic acid and acetic acid, bacteriocins, the bacterial fermentation of some drugs, and the synthesis of signal and surface molecules [Citation6]. The probiotic dietary supplements represent the second largest segment of the probiotics market and it is growing by the year. However, consumers are interested whether the labels claiming that the products contain a large number of viable and functionally effective probiotic bacteria are correct. The relevance of correct identification and typing of strains used in probiotic products is increasing for two main reasons. First, the use of probiotic strains is no longer strictly restricted to food applications. In recent years they are also applicable as biotherapeutic and pharmaceutical formulations. These formulations are intended for specific patient groups with different clinical profiles and in this regard it is essential to ensure reliable identification at species and strain level. Second, а new aspect in the use of probiotics involves the introduction of several mixed-strain formulations in dietary supplements as well as in therapeutic applications with health-promoting claims. Thus, every individual strain within such mixtures needs accurate characterization both on a taxonomical and functional level [Citation7]. In recent years, there is growing scientific evidence that the number and identification of recovered species in some probiotic products not always correspond to the species composition on the labels [Citation8–14].
At present, such incorrect species designations of probiotic strains [Citation15] and mislabelling of probiotic products [Citation16,Citation17] are mainly attributed to the inappropriate use of identification methods. Any inconsistencies in the microbial identification of commercial probiotic products will affect their potential efficacy and safety record, and are likely to have a negative impact on consumer trust [Citation7]. Another problem could be the extremely variable tolerance to gastric acid and bile salts depending on the species and strain composition of the formulations [Citation18]. A recent position paper by the ESPGHAN working group for probiotics and prebiotics [Citation19] reviewed that decreased acid or bile tolerance, impaired abilities to colonize and to adhere to intestinal cells, and inability to inhibit or exclude a pathogen can all be found within the same species, this being influenced by the manufacturing processes and the food matrix used [Citation20]. The position paper further underlines that few studies have yielded satisfactory results but the majority report labelling inconsistency in most of the tested products [Citation21,Citation22]. A number of attempts have been made to improve the viability of probiotics in different food products during their production until the time of consumption. The survival of probiotic microorganisms in food products during production, processing and storage depends on multiple factors. These include food parameters, e.g. pH value, water activity, presence of salt, sugar, hydrogen peroxide, bacteriocins, flavours and colourants. Another set of factors are associated with the type of processing, e.g. heat treatment, incubation temperature, cooling rate of the product, packaging materials and storage methods, and the scale of production. There are also microbiological factors that affect the viability of the probiotic cells: the technological properties of the strains and the inoculation rate and proportion [Citation23,Citation24]. Last but not least, it has now become clear that probiotic administration in at-risk populations could become a problem, causing several diseases like sepsis, endocarditis, localized and opportunistic infections etc. [Citation25,Citation26]. Lack of specific and stringent regulation of the registration process of probiotics, leads to no appropriate protection of producers and customers and finally results in reduction of quality and health benefits of dietary supplements [Citation27].
In this regard, the purpose of the present work was to evaluate the microbiological and functional qualities of imported and local probiotic supplements in the Bulgarian market and to propose a molecular test procedure for quality control of probiotic dietary supplements.
Materials and methods
Probiotic products
Twenty-six probiotic products (16 imported commercially available ones and 10 powdered products provided from a local manufacturer) were evaluated in the present study (). All products were stored according to the manufacturers’ requirements and were analysed at least 6 months before the expiration date.
Table 1. Expected and actual counts of LAB in some commercially available probiotic supplements and in probiotic products provided by a local manufacturer.
Isolation and quantification of viable bacteria
Isolation and quantification of viable bacteria were performed according the standardized procedure described in ISO 15214 and ISO 6887 by incubation for at least 48 h in anaerobic conditions (Anaerocult A, Merck Millipore, Germany). Each probiotic sample was rehydrated in 9 mL tryptone water for 24 h at 37 °C. The resultant suspensions were diluted 10-fold and aliquots of 100 µL were grown in anaerobic conditions (Anaerocult A, Merck Millipore, Germany) on MRS agar (Merck Millipore, Germany) and NA (Nutrient agar, Merck Millipore, Germany) plates in three replicates per dilution. The number of viable cells was scored after incubation by standard microbiological counting methods. Depending on the number of morphological types of colonies on each agar plate, 2–3 colonies of each type were randomly selected and transferred onto a new plate. Distinct colonies were sub-cultured in MRS broth (HiMedia Laboratories, India) and were identified phenotypically.
Molecular identification of isolates
Genomic DNA was extracted from overnight MRS broth cultures of all isolates by using a Tissue and Bacterial DNA Purification kit (EURx Ltd., Poland). The extracted DNA samples were stored at −20 °C. Molecular differentiation of isolated LAB at the genus level was carried out by polymerase chain reaction (PCR) amplification of 16S–23S ITS rDNA with the universal primers 16S/4(5-GCTGGATCACCTCCTTTCT-3) and 23S/7 (5-GGTACTTAGATGTTTCAGTTC-3) as previously described [Citation28]. The genus affiliation of the strains obtained by the above methods was confirmed by PCR amplification with genus-specific primer pairs (LbLMA1/R16-1) [Citation29]. Species grouping and species identification of Lactobacillus isolates were performed according to Song et al. [Citation30].
In vitro resistance of LAB in simulated gastrointestinal conditions
The gastrointestinal tolerance of the probiotic strains was determined using simulated gastric and pancreatic juices. They were prepared just before use by diluting pepsin (3 mg mL−1) and pancreatin USP (1 mg mL−1) in sterile saline (0.5% w/v), respectively. The pH of the resultant solutions were adjusted to pH 2 for pepsin and pH 8 for pancreatic juice using 37% HCl and 5 mol L−1 NaOH, respectively [Citation31]. Washed cell suspension (1.0 mL) obtained from each overnight culture was added to 9.0 mL of simulated pepsin and pancreatin juices, and was vortexed for 5 s for complete dispersion of cells. The samples for analysis were taken at 1, 90 and 180 min for pepsin resistance and at 1 and 240 min for pancreatin tolerance. To detect the tolerant cells, the samples were transferred onto MRS agar and incubated at 37 °C for 48 h and the viable counts were determined using a pour-plate technique.
Antibacterial assay
The antimicrobial activity of the isolated LAB was determined by the agar overlay method [Citation32]. The antibacterial activity of native and neutralized cell-free supernatants (CFS) obtained after cultivation of the LAB was evaluated by the agar well diffusion method [Citation33]. The following test microorganisms were used: Listeria innocua F 4078, Bacillus subtilis NBIMCC 2353, Escherichia coli NBIMCC 3548, Salmonella enterica serotype Enteritis NBIMCC 8691, and Candida glabrata.
Results and discussion
Microbiological quality of probiotic supplements
The microbiological quality of the probiotic supplements was determined on selective media according to standardized ISO procedures. According to the requirements, viable probiotic bacteria must be at least 106–107 CFU g−1. The present investigation determined that the content of living bacteria in none of the 16 samples bought from the Bulgarian market fully met the quantity indicated on the label. Viable bacteria were not detected in 11.53% of the tested samples and 7.69% contained a minimal amount (102 CFU g−1). Approximately 38.46% of dietary supplements contained 105–106 CFU g−1 viable bacteria. Only the 10 samples from a local manufacturer contained 108–1010 CFU g−1 probiotic bacteria in accordance with the product specifications ().
Next, we determined the cultural and morphological properties of about 890 isolated colonies. The results showed that 420 small, round and opaque, cream-coloured colonies with fine-grained structure had characteristics of LAB: Gram-positive, non-sporeforming rods or cocci, catalase and oxydase negative, and aerotolerant. Ninety-seven presumptive LAB strains were purely isolated and were phenotypically characterized. Ninety per cent of the isolates were rod-shaped and 10% were spherical in short and medium-length chains. Other types of colonies isolated on nutrient agar did not have the characteristics of lactic acid bacteria or yeast. The analysis of the microbiological quality showеd that more than 42% of the tested probiotic supplements contained unacceptable microbial contamination. These results are in agreement with those obtained in other investigations on dietary supplements. Coeuret et al. [Citation13] found that 50% of 10 commercial dairy probiotic products contained fewer lactobacilli than claimed on the labels and no viable lactobacilli were recovered from the food supplements tested. Another study reported that the viable bacterial counts were generally lower in food supplements than in dairy products, there being no viable bacteria in 37% of food supplements [Citation12]. A microbiological analysis of the quality of 25 probiotic products (medicinal products, dietary supplements and food for special medical purposes (FSMP)) available on the Polish market showed that not all of these preparations possess a suitable number of bacteria [Citation14]. Only one medicinal product, two dietary supplements and two FSMP of all tested 25 different products showed good quality with respect to the number of bacterial cells [Citation14]. This may be due to disruption of the cold chain, strain-dependent loss of viability or a decrease in numbers during storage [Citation11]. As the probiotic effect is dependent on the number of viable bacteria, any decrease results in loss of probiotic quality.
Molecular identification of LAB isolates
According to the product specifications, the tested dietary supplements had to contain different probiotic species. We investigated the actual species diversity by a diagnostic molecular algorithm designed in this study (). All putative lactic acid strains were subjected to preliminary molecular characterization using the 16S–23S ITS PCR approach. Fifty-seven rod-shaped bacteria formed amplification patterns with two fragments (450 bp and 650 bp) and 16 coccus bacteria formed three fragments (450 bp, 550 bp and 650 bp) (). By comparing our results with the available literature data [Citation28], we could conclude that 57 isolates belonged to genus Lactobacillus and 16 ones could be attributed to genus Weissella. Species of the genus Weissella were not described on any product label, so it is possible that the producers had used incorrectly taxonomically determined strains of Streptococcus thermophilus or Lactococcus lactis. The genus affiliation of Lactobacillus strains was confirmed by genus-specific primers. Fifty-two strains generated an expected fragment of about 250 bp. Five strains were not confirmed on the genus level.
Figure 2. PCR amplification and identification of selected strains at genus level via primer pair 16S/p4–23S/p7: M, molecular marker (Fermentas, 100 bp); strains number 3, 5–9, 15, 28–32 formed amplification patterns with two fragments (450 bp and 650 bp); strains number 1–2, 4, 10, 11–14, 16–21, 22–26 formed amplification patterns with three fragments (450 bp, 550 bp and 650 bp).
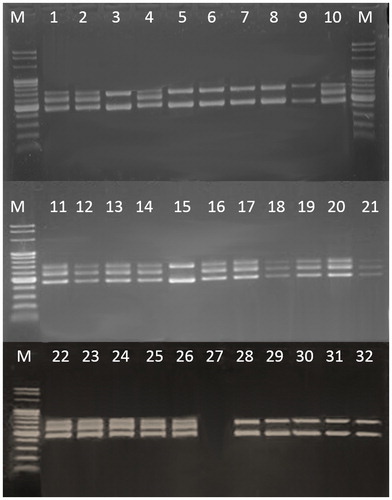
Species grouping and species identification of Lactobacillus isolates were performed by a two-step multiplex PCR using group- and species-specific primers. The first multiplex with primers Ldel-7/Lac 2, Lu1/Lac2, Lu-5/Lac2 and Lu-3/Lac2, separated the Lactobacillus isolates into four groups according to Song et al. [Citation30]: Group I (Lactobacillus delbrueckii ssp. bulgaricus/lactis) – 10 isolates; Group II (Lactobacillus acidophilus, Lactobacillus helveticus, Lactobacillus amylovorus, Lactobacillus crispatus, Lactobacillus gasseri, Lactobacillus johnsonii) – 7 strains; Group III (Lactobacillus paracasei, Lactobacillus casei and Lactobacillus rhamnosus) – 18 isolates; and Group IV (Lactobacillus plantarum, Lactobacillus fermentum, Lactobacillus salivarius and Lactobacillus reuteri) – 9 ones. All strains were subjected to a second multiplex PCR with species-specific primers. The PCR results revealed that, out of 44 isolates, only 17 strains were identical to the expected species: L. bulgaricus (3 strains), L. acidophilus (4 strains), L. casei (3 strains), L. rhamnosus (2 strains), L. reuteri (4 strains) and L. plantarum (1 strain). According to the specifications, most probiotic products were supposed to contain the species Lactobacillus delbrueckii ssp. bulgaricus. Our studies confirmed its presence in only three probiotic products. Similar results were obtained for L. acidophilus. Surprisingly, in most of the dietary supplements included in our study, we were able to identify species that are not included on the label. Similarly, other authors investigating the quality of probiotic supplements have reported that none of the products tested in their studies contained all of the types described on the label and most of the products contained species other than those listed [Citation19,Citation34]. Our studies also showed that some strains were not identified or many were misidentified in a number of products.
Functional properties of isolated LAB bacteria
The presence of living bacteria in dietary supplements does not guarantee their efficiency. In vitro, gastric juice was simulated by incubating the strains in pepsin-containing medium, pH 2.0. The viable cell population was determined before and after incubation (1, 90 and 180 min) on MRS agar plates by the spread plate method (). The acidic tolerance of all the species tested in the present study was variable. The cell counts of the tested strains decreased by one or two orders of magnitude immediately after the cells were introduced into the pepsin solution. Further incubation at pH 2 caused cell death in most of the strains. Only the strains Lactobacillus delbrueckii ssp. bulgaricus 52, L. acidophilus N2, L. acidophilus 64 and L. plantarum 84 were able to survive for 90 min in pepsin solution, but the cell counts decreased by 5 or 6 orders of magnitude compared to the control. None of the strains survived following 180 min of incubation.
Charteris et al. [Citation31] indicated that the resistance of L. delbrueckii ssp. bulgaricus to pepsin is very weak (2–5% of cells remain viable). The strains of Lactobacillus delbrueckii ssp. bulgaricus tested in our study had significaly higher pepsin resistance (25% and 17% viable cells) compared to the data reported by Charteris et al. [Citation31]. According to Jensen et al. [Citation22], the Lactobacillus species retain vitality up to 180 minutes, reducing their viability by about 1- to 4-fold. Similar results have been described for the species L. acidophilus, L. rhamnosus and L. plantarum. The survival rate of the mentioned strains was about 50% after 120 min of contact with simulated gastric juice at pH 2 [Citation35]. The same authors reported that at pH 3, the survival rate was significantly higher even after 120 min. Probably, in the technological process, probiotic strains partially lose their resistance to acidic environment or Lactobacillus showed a high level of strain specificity. Therefore, according to our data, it may be assumed that some of the tested strains belonging to L. bulgaricus, L. acidophilus and L. plantarum have the potential to survive in real gastric conditions.
Simulation of conditions corresponding to those in the small intestine was done by incubating the test strains in pancreatin solution, pH 8.0. The number of surviving cells was determined after 1 and 240 min. The strains were less sensitive in these conditions than in the simulated gastric juice conditions. At the first contact with pancreatin, the bacterial cells showed better resistance and decreased by about 2–5% of their initial count. At the end of the studied period, the percentage of surviving cells decreased to about 30%. Jensen et al. [Citation22] conducted a similar experiment, also demonstrating that the strains used by them are significantly more resistant to pancreatin than to pepsin. According to them, some of the strains of L. plantarum, L. sakei and L. reuteri even remain unaffected by pancreatin throughout the 240-min period of contact with the simulated intestinal juice or show a decrease in the viable counts at 240 min by 10–20%.
Our results showed that the strains tested were more sensitive to the simulated gastric juice conditions, many of them not surviving after 90 min. However, the tested strains were more tolerant to simulated small intestine conditions.
Antibacterial assessment
Only acid native CFSs of the 10 Lactobacillus strains isolated from dietary supplements showed inhibitory effect against Escherichia coli NBIMCC 3548, Salmonella enterica serotype Enteritis NBIMCC 8691 and Bacillus subtilis NBIMCC 2353, forming relatively small inhibition zones (12–14 mm). The results showed that the tested probiotic isolates did not synthesize bacteriocins or bactericidal substances. Inhibition of E. coli NBIMCC 3548, Salmonella enteritica serotype Enteritidis NBIMCC 8691 and B. subtilis NBIMCC 2353 was due to lactic acid production. None of the strains of lactic acid bacteria exhibited inhibitory properties against Candida glabrata and Listeria innocua F4078.
Conclusions
The results of our study showed that almost none of the tested probiotic products had satisfactory quality. There were deviations from the information provided on the labels about the strain composition of the products. One of the most common problems was the lower number of total viable counts in the products. There was also detected undesired microflora in some of the products. The strains used in the tested probiotic supplements had poor functional properties that did not meet the criteria for the products to be considered probiotic. The current use of probiotic bacteria in dietary supplements could be accompanied by new knowledge on how these bacteria may be influenced by different factors inherent to the technological processes, the physicochemical environment of food and the gastrointestinal transit. Thus, it could be recommended that proof of the in vivo functionality of supplements be requested as a procedure that allows commercialising ‘proven’ probiotic products, not potential ones. The molecular identification strategy proposed in this study demonstrates a rapid, useful and powerful tool to identify lactic acid bacteria in dietary supplements at the species level. On the basis of the obtained results, it can be concluded that all probiotic products available on the Bulgarian market should be subjected to routine and thorough inspection by relevant authorities. The reported work could be of help to elaborate guidelines for the control of probiotic lactobacillus strains in human foods.
ORCID details
Yoana Krasimirova Kizheva https://orcid.org/0000-0002-1140-4671
Disclosure statement
No potential conflict of interest was reported by the authors.
Additional information
Funding
References
- Vandenplas Y, Huys G, Daube G. Probiotics: an update. J Pediatr (Rio J). 2015;91:6–21.
- Astashkina AP, Khudyakova LI, Kolbysheva YV. Microbiological quality control of probiotic products. Procedia Chem. 2014;10:74–79.
- World Health Organization (WHO), Food and Agriculture Organization of the United Nations (FAO). Probiotic in foods. Health and nutritional properties and guidelines for evaluation. Rome, Italy; 2006.
- Boylston TD, Vinderola CG, Ghoddusi HB. Incorporation of Bifidobacteria into cheeses: challenges and rewards. Int Dairy J. 2004;14:375–387.
- Zheng M, Zhang R, Tian X, et al. Assessing the risk of probiotic dietary supplements in the context of antibiotic resistance. Front Microbiol. 2017;8:908.
- Bondarenko VM, Vorobyev аа. Dysbiosis and medications with probiotic function. ZHurn Mikrobiol. 2004;1:84–92.
- Huys G, Botteldoorn N, Delvigne F, et al. Microbial characterization of probiotics – advisory report of the working group “8651 Probiotics” of the Belgian Superior Health Council (SHC). Mol Nutr Food Res. 2013;57:1479–1504.
- Klein G, Pack A, Bonaparte C, et al. Taxonomy and physiology of probiotic lactic acid bacteria. Int J Food Microbiol. 1998;41:103–125.
- Hamilton-Miller JMT, Shah S. Deficiencies in microbiological quality and labelling of probiotic supplements. Int J Food Microbiol. 2002;72:175–176.
- Hamilton-Miller JMT, Shah S, Winkler JT. Public health issues arising from microbiological and labelling quality of foods and supplements containing probiotic microorganims. In: Public health nutrition. 2nd ed. Chichester, West Sussex (UK); Ames, Iowa (USA): John Wiley & Sons; 1999.
- Shah NP. Probiotic bacteria: selective enumeration and survival in dairy foods. J Dairy Sci. 2000;83:894–907.
- Temmerman R, Pot B, Huys G, et al. Identification and antibiotic susceptibility of bacterial isolates from probiotic products. Int J Food Microbiol. 2003;81:1–10.
- Coeuret V, Gueguen M, Vernoux JP. Numbers and strains of lactobacilli in some probiotic products. Int J Food Microbiol. 2004;97:147–156.
- Zawistowska-Rojek A, Zareba T, Mrówka A, et al. Assessment of the microbiological status of probiotic products. Pol J Microbiol. 2016;65:97–104.
- Huys G, Vancanneyt M, D'Haene K, et al. Accuracy of species identity of commercial bacterial cultures intended for probiotic or nutritional use. Res Microbiol. 2006;157:803–810.
- Masco L, Huys G, De Brandt E, et al. Culture-dependent and culture-independent qualitative analysis of probiotic products claimed to contain bifidobacteria. Int J Food Microbiol. 2005;102:221–230.
- Temmerman R, Scheirlinck I, Huys G, et al. Culture-independent analysis of probiotic products by denaturing gradient gel electrophoresis. Appl Environ Microbiol. 2003;69:220–226.
- Vecchione A, Celandroni F, Mazzantini D, et al. Compositional quality and potential gastrointestinal behavior of probiotic products commercialized in Italy. Front Med (Lausanne). 2018;7:59.
- Kolaček S, Hojsak I, Canani R, et al. Commercial probiotic products: a call for improved quality control. A position paper by the ESPGHAN working group for probiotics and prebiotics. J Pediatr Gastroenterol Nutr. 2017;65:117–124.
- Grzeskowiak L, Isolauri E, Salminen S, et al. Manufacturing process influences properties of probiotic bacteria. Br J Nutr. 2011;105:887–894.
- De Vecchi E, Nicola L, Zanini S, et al. In vitro screening of probiotic characteristics of some Italian products. J Chemother. 2008;20:341–347.
- Jensen H, Grimmer S, Naterstad K, et al. In vitro testing of commercial and potential probiotic lactic acid bacteria. Int J Food Microbiol. 2012;153:216–222.
- Tripathi MK, Giri SK. Probiotic functional foods: survival of probiotics during processing and storage. J Funct Foods. 2014;9:225–241.
- Călinoiu LF, Vodnar DC, Precup G. The probiotic bacteria viability under different conditions. Bull UASVM Food Sci Technol. 2016;73:55–60.
- Vallabhaneni S, Walker TA, Lockhart SR, et al. Notes from the field: fatal gastrointestinal mucormycosis in a premature infant associated with a contaminated dietary supplement – Connecticut, 2014. Centers for Disease Control and Prevention (CDC). MMWR Morb Mortal Wkly Rep. 2015;64:155–156.
- Kothari D, Patel S, Kim SK. Probiotic supplements might not be universally-effective and safe: a review. Biomed Pharmacother. 2019;111:537–547.
- de Simone C. The unregulated probiotic market. Clin Gastroenterol Hepatol. 2019;17:809–817.
- Kabadjova P, Dousset X, Le Cam V, et al. Differentiation of closely related carnobacterium food isolates based on 16S–23S ribosomal DNA intergenic spacer region polymorphism. Appl Environ Microbiol. 2002;68:5358–5366.
- Dubernet S, Desmasures N, Gueguen M. A PCR-based method for identification of lactobacilli at the genus level. FEMS Microbiol Lett. 2002;214:271–275.
- Song Y, Kato N, Liu C, et al. Rapid identification of 11 human intestinal Lactobacillus species by multiplex PCR assays using group- and species-specific primers derived from the 16S–23S rRNA intergenic spacer region and its flanking 23S rRNA. FEMS Microbiol Lett. 2000;187:167–173.
- Charteris WP, Kelly P, Morelli L, et al. Development and application of an in vitro methodology to determine the transit tolerance of potentially probiotic Lactobacillus and Bifidobacterium species in the upper human gastrointestinal tract. J Appl Microbiol. 1998;84:759–768.
- Chowdhury A, Malaker R, Nur Hossain M, et al. Bacteriocin profiling of probiotic Lactobacillus spp. isolated from yoghurt. Int J Pharm Chem. 2013;3:50–56.
- Tagg JR, McGiven AR. Assay system for bacteriocins. Appl Microbiol. 1971;21:943.
- Farahmand N. Characterization of probiotic Lactobacillus spp. isolates from commercial fermented milks [dissertation]. London (UK): London Metropolitan University; 2015.
- Ashraf R, Smith SC. Commercial lactic acid bacteria and probiotic strains – tolerance to bile, pepsin and antibiotics. Int Food Res J. 2016;23:777–789.