Abstract
Anthocyanins are among the most important factors affecting the leaf colour of kale, and MYB proteins are key transcription factors regulating their synthesis in plants. In this study, purple-leaf kale ‘D07’ was used as a test material, and the complete coding sequence of BoMYB was determined by homologous cloning. Bioinformatics analysis showed that the cDNA sequence of the BoMYB coding region was 753 base pairs in length and encoded a predicted 250-amino acid protein with a mass of 25 kDa and an isoelectric point of 9.08. The BoMYB protein was predicted to contain two MYB conserved domains and to be localized in the cell nucleus. A phylogenetic tree indicated that the amino acid sequence encoded by BoMYB in kale was most closely related to the MYB protein sequence of Brassica napus. The expression patterns of BoMYB were evaluated in different tissues of ‘D07’ by quantitative real-time polymerase chain reaction, and the results showed that BoMYB was expressed in kale roots, stems, inner leaves, and outer leaves. However, the expression levels were tissue specific and correlated with the anthocyanin content in each tissue. To confirm the effect of BoMYB on anthocyanin synthesis and accumulation, ectopic expression in Arabidopsis was performed. Morphological observation showed that overexpression of BoMYB increased the accumulation of anthocyanidins in transgenic Arabidopsis. Thus, BoMYB may encode an MYB transcription factor, which positively regulates the synthesis of anthocyanins in kale.
Introduction
Kale (Brassica oleracea L. var. acephala, 2n = 18), native to the Mediterranean and Asia Minor, is a biennial herb variant of B. oleracea (Brassicaceae), which belongs to the same group as wild cabbage (Brassica oleracea L. var. oleracea), purple cabbage (Brassica oleracea L. var. capitata), savoy cabbage (Brassica oleracea L. var. subauda), kohlan (Brassica oleracea var. caulorapa) and brussels sprouts (Brassica oleracea L. var. gemmifera), and grows in the coastal areas of north-western Europe [Citation1]. These plants can be used for urban greening purposes and as food [Citation2]. There are three types of kale based on leaf shape: crinkle leaf, round leaf, and leather leaf kale. The leaf colour can be pure white, yellow-white, yellow-green, pink, rose red, purple, etc. The pink, rose red, purple, and other colours are closely associated with the anthocyanin content in leaves [Citation3].
Anthocyanins are one of the most important metabolites in flavonoid biosynthesis pathways and are water-soluble natural flavonoid pigments conferring colours such as red, pink, blue, purple and even black to flowers, fruits, stems, and leaves of plants [Citation4]. Anthocyanidins found in plants are unstable and are typically combined with sugars to form glycosides, anthocyanins [Citation5].
The biosynthesis of anthocyanins is controlled by two different types of genes. One is structural genes, which encode catalytic enzymes of anthocyanin biosynthesis, and the other is transcription factors, which participate in the formation of the plant colour at the transcriptional level by regulating the expression of structural genes [Citation6]. Transcriptional factors bind to recognized cis-acting elements of structural gene promoters and regulate structural genes in anthocyanin biosynthetic pathways, either alone or in concert, thereby effectively controlling anthocyanin biosynthesis in plants.
The MYB family of proteins is large, functionally diverse, and is represented in all eukaryotes. Most MYB proteins function as transcription factors with varying numbers of MYB domain repeats conferring their ability to bind DNA [Citation7]. A variety of plant studies have shown that MYB transcription factors play an important regulatory role in anthocyanin biosynthesis pathways. Some key MYB transcription factors can significantly increase the anthocyanin content when overexpressed in plants, such as AtMYB75/PAP1 in Arabidopsis, SlANT1 in tomato (Solanum lycopersicum L.), MdMYB10 and MYB110a in apple (Malus ×domestica), BoMYB2 in cauliflower (Brassica oleracea var. botrytis), and PmMYB1 in plum blossom Prunus mume, OjMYB1 in water dropwort (Oenanthe javanica) [Citation8–14], and their loss of function or suppression of expression prevents anthocyanins from normally accumulating in plants [Citation15–17]. In contrast, expression of other MYB genes inhibits the biosynthesis of anthocyanins, but when their activity is lost, expression of structural genes is upregulated and the anthocyanin content increases [Citation18].
Studies of anthocyanin biosynthesis in cruciferous crops have mainly focused on plants such as Arabidopsis thaliana, purple cabbage (Brassica oleracea L. var. capitata), purple heading Chinese cabbage (Brassica campestris L. ssp. pekinensis), and flowering Chinese cabbage (Brassica campestris L. var. purpurea) [Citation7, Citation19–22]. In these studies, the effects of environmental factors such as light and temperature on the expression of genes related to anthocyanin biosynthesis were examined, and a model of MYB, basic helix–loop–helix, and interaction with WD40 to control anthocyanin synthesis were determined. Few studies have examined the synthesis of anthocyanins in kale [Citation3, Citation23], and no transgenic method has been reported for functional verification of related genes. In this study, a gene (BoMYB) encoding an R2R3-MYB transcription factor, related to anthocyanin regulation, was cloned from purple-leaf kale, and its expression was analysed in different organs of the plant. Agrobacterium tumefaciens-mediated transformation of A. thaliana was carried out to explore the effect of heterologous expression of BoMYB on the transgenic Arabidopsis phenotype to further verify the function of the gene.
Materials and methods
Plant material
The pure line ‘D07’ of purple-leaf kale was preserved in our laboratory. Plants were grown in a temperature- and light-controlled greenhouse at a temperature of 25 °C/10 °C (day/night) and light on for 16 h. In mid-September, seeds were sown into a bowl containing nutritional soil and then transplanted into pots (diameter: 15 cm) at the four-leaf stage. Growth continued to the optimal ornamental period until the end of October, after which samples were collected and stored at −80 °C.
Determination of the anthocyanin content, total RNA extraction and cDNA synthesis
The anthocyanin content in the roots, stem, inner leaves, and outer leaves of kale and in the entire Arabidopsis plant was determined following anthocyanin extraction as described previously [Citation24]. Total RNA was extracted from leaves using an RNA extraction kit (Invitrogen, Carlsbad, CA, USA) according to the manufacturer’s instructions. The concentration of RNA was determined using a NanoDrop 2000 spectrophotometer (Thermo Fisher Scientific, Waltham, MA, USA), and RNA integrity was determined by agarose gel electrophoresis. First-strand cDNA was synthesized using an AMV reverse transcriptase kit (Sangon Biotech, Shanghai, China).
BoMYB cloning and sequence analysis
The conserved sequence of the A. thaliana MYB90 gene (NP_176813.1) from GenBank was analysed and used to identify homologous genes in the Brassica genome database (http://brassicadb.org/brad/), as well as for BLAST comparisons. Specific primers, forward (5′-ATGGAGGATTCGTCCAAAGGGTTGAC-3′) and reverse (5′-ATCAAGTTCTACAGTCTCTCCATCCAAC-3′), were designed for cDNA amplification using the Primer 5.0 software. The amplification reactions (25 µL) were prepared, each containing H2O 9.5 µL, PCR SuperMix 12.5 µL, primers (10 µmol L−1) of 1 µL each, template 1 µL. The amplification conditions were as follows: 98 °C for 10 s; 63 °C for 30 s; 72 °C for 60 s; 30 cycles, and lastly 72 °C for 10 min (VeritiTM 96-Well Thermal Cycler, Applied Biosystems, USA). The polymerase chain reaction (PCR) products were cloned into the pGWC vector and confirmed by sequencing. Physical and chemical properties (amino acid composition, molecular weight and isoelectric point) of the encoded protein were analysed using the ExPASy database (http://www.expasy.org/). The nuclear localization signal was predicted using WoLF PSORT (http://www.genscript.com/wolf-psort.html), and conserved protein structural domains were analysed with InterPro (http://www.ebi.ac.uk/interpro/). The secondary protein structure was predicted using SOPMA (http://www.sopma.org/), and amino acid sequence alignment was performed using DNAMAN. A phylogenetic tree of MYB genes was generated using the neighbour-joining method with bootstrap support (1,000 replicates), and the branch lengths are proportional to the phylogenetic distances.
Expression analysis
Specific primers, BoMYB-RT-F (5′-AGGTGTAGGAAGAGTTGTAGAC-3′) and BoMYB-RT-R (5′-AGAAGATCAACTTCATCAGAGC-3′) were designed to detect the expression of BoMYB in the root, stem, inner leaf, and outer leaf by quantitative real-time PCR. The 18S rRNA gene was used as a reference gene for normalization and was amplified using the 18S-RT-F (5′-CCAGGTCCAGACATAGTAAG-3′) and 18S-RT-R (5′-GTACAAAGGGCAGGGACGTA-3′) primers. The reaction mixture contained 5 μL of 2× QuantiFast® SYBR® Green PCR master mix (Qiagen, Hilden, Germany), 0.2 μL of each forward and reverse primer, 1 μL of cDNA template (1:10 dilution), and nuclease-free water added to a total volume of 10 μL. The reactions were performed at 95 °C for 5 min, followed by 40 cycles at 95 °C for 10 s and 60 °C for 30 s (VeritiTM 96-Well Thermal Cycler, Applied Biosystems, USA). Each sample was run in triplicate. At the end of PCR, melting curve analysis was performed to validate the specificity of the expected PCR product. The data were analysed using the 2−ΔΔCt method [Citation25]. The expression level of the BoMYB gene in the leaves of transgenic Arabidopsis plants was detected using the same method as described above. The actin-12 gene of Arabidopsis is a reference gene, and the primer is actin-RT-F (5′-ACACTTTCTACAATGAGCTGC-3′) and actin-RT-R (5′-TCTGTGTCATCTTCTCACGG-3′).
Vector construction and transformation of Arabidopsis
The plasmids of entry vectors (pGWC-BoMYB) were extracted and recombined using the Gateway® LR Clonase® II Enzyme Mix kit (Invitrogen). The following reagents were added to the centrifuge tube: recombinant plasmid pGWC-BoMYB (50–100 ng µL−1) 1 µL, pEarleyGate 103 (50–100 ng µL−1) 1 µL, LR Clonase Enzyme Mix II l µL, ddH2O 2 µL. The reaction was left overnight at 25 °C, and a 2.5-µL reaction solution was transformed into Escherichia coli DH5α (Tiangen Biotech, Beijing, China). Positive clones were selected on Luria–Bertani solid medium containing 50 μg mL−1 kanamycin and 50 μg mL−1 hygromycin B to confirm that the correct vector was produced (). The constructed expression vector pEarleyGate-BoMYB was transformed into Agrobacterium by a freeze-thaw method, followed by transformation into wild-type A. thaliana Col-0 by dipping. The anthocyanin content and expression levels of BoMYB were analysed in transgenic Arabidopsis as described above. Wild-type and transgenic Arabidopsis plants were grown on Murashige and Skoog medium in a growth chamber at 22 °C, 70% relative humidity and a 16-h photoperiod (120–150 μmol m−2 s−1).
Results and discussion
BoMYB cDNA cloning and analysis
Transcription factors, also known as trans-acting factors, are DNA-binding proteins that interact specifically with cis-acting elements of eukaryotic genes and activate or inhibit their transcription [Citation26]. Studies have shown that most key genes in plant anthocyanin synthesis are co-expressed, which may occur through coordinated activation of expression of structural genes by transcription factors from one or more gene families. As the understanding of anthocyanin biosynthesis pathways has increased, transcription factor-encoding genes, regulating the expression of structural genes in biosynthetic pathways, have gradually become the focus of research. Previous studies have shown that MYB transcription factors are key transcription factors involved in anthocyanin biosynthesis [Citation27].
In the present study, the cDNA sequence was obtained by reverse transcription of total RNA from leaves of purple kale, and the target gene of approximately 750 base pairs (bp) was amplified. The sequencing results showed that the target gene was 753 bp in length, and it was named BoMYB (GenBank accession number: MG827397). Analysis of the BoMYB protein revealed that it had a molecular weight of 28.5 kDa, isoelectric point of 9.08, molecular formula C1255H1996N360O368S15, theoretical half-life of 30 h, instability index of 39.56, and aliphatic index of 75.64, indicating that it was a stable protein. The protein sequence consisted of 250 amino acids and was compared to MYB sequences of other plants by constructing a phylogenetic tree (). The amino acid sequences of BoMYB and the MYB protein of B. napus were grouped into one cluster, showing the closest genetic relationship. BoMYB was also closely related to the MYB proteins of Brassica oleracea, Raphanus sativus, Arabidopsis thaliana, and Camelina sativa and was farthest from those of Nicotiana tomentosiformis and Gossypium hirsutum.
Figure 2. Phylogenetic tree showing the evolutionary relationship and the homology degrees among the MYB amino acid sequences. The estimated genetic distance between sequences is in proportional to the lengths of the horizontal lines connecting one sequence to another. The sequences are of the following MYB transcription factors: Brassica napus (XP_013681390.1), Raphanus sativus (XP_0184687473.1), Gossypium hirsutum (XP_016694638.1), Nicotiana tomentosiformis (NP_001306786), Tarenaya hassleriana (XP_010551826.1), Camelina sativa (XP_010513542.1), Arabidopsis thaliana (NP_176813.1), Arabidopsis lyrata subsp. (XP_002887034.1), and Brassica oleracea (XP_013590812.1).
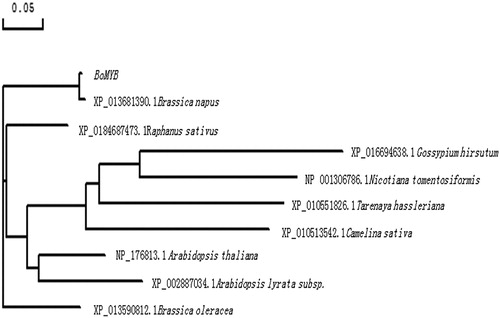
MYB proteins have a relatively conserved domain structure and are classified into single domain-containing R1/2-MYBs, two repeat domain-containing R2R3-MYBs and three repeat domain-containing R1R2R3-MYBs. Most MYB proteins in plants are type II proteins with a two-repeat domain (R2R3), and many studies have shown that some members of the R2R3-MYB gene family regulate anthocyanin biosynthesis [Citation28, Citation29]. The VvMyb5b transcription factor in grape enhances the expression of the ANS, CHI, and LAR promoters and induces the expression of flavonoid- and anthocyanin-related structural genes [Citation30]. The LhMYB12 and LhMYB6 transcription factors in lily control the perianth colouration and plaque formation, which directly affects the spatiotemporal accumulation of anthocyanins in the flowers [Citation31]. VmMYB2 in bilberry is involved in metabolic regulation of anthocyanins, and its function is directly or indirectly regulated by the MADS-box gene [Citation32]. The SmMYB gene that positively regulates anthocyanins has also been isolated from eggplant [Citation33]. When the Rosea 1 and Delila genes of snapdragon were simultaneously transformed into tomato, a large amount of anthocyanins were accumulated in the peel of transgenic tomato, and the fruit became purple, with the trait stably transferred to the offspring [Citation34].
The results obtained using the InterPro database showed that BoMYB had two typical DNA-binding domains () and was a typical R2R3-MYB transcription factor. The protein sequence contained a conserved tryptophan (W) at positions 13, 33, 53, 85 and 104. The SOPMA online tool predicted that the secondary structure of the BoMYB protein consisted of alpha-helices (35.2%), irregular coils (40.4%), beta-turns (12.4%) and extended strands (12.0%). Subcellular localization prediction showed that the BoMYB protein was localized in the nucleus. Based on these results, BoMYB has the characteristics of a transcription factor and is a MYB family member.
Figure 3. Alignment of BoMYB protein sequense of Brassica oleracea L.var.acephalawith Brassica napus MYB (GenBank Ac. No. XP_013681390.1), Raphanus sativus MYB (GenBank Ac. No.XP_0184687473.1), Gossypium hirsutum MYB (GenBank Ac. No.XP_016694638.1), Nicotiana tomentosiformis MYB (GenBank Ac. No.NP_001306786), Tarenaya hassleriana MYB (XP_010551826.1), Camelina sativa MYB (XP_010513542.1), Arabidopsis thaliana MYB (NP_176813.1), Arabidopsis lyrata subsp. MYB (XP_002887034.1), and Brassica oleracea MYB(XP_013590812.1). The identical amino acids are shown as white letters on a black background. Alignment within solid line shows R2 and R3 domains. Conservative 13th, 33rd, 53rd, 85th, and 104th amino acids in R2 and R3 domains.
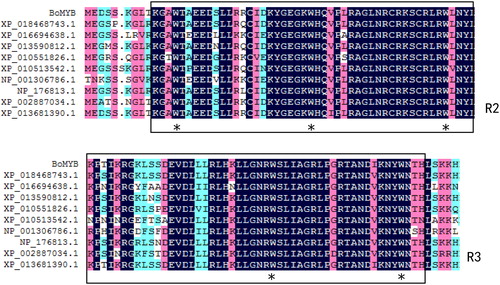
Anthocyanin content and expression of BoMYB in kale
Kale exhibits a wide variety of colours, with the pigments in pink, red, and purple lines mainly represented by anthocyanins. To understand the anthocyanin biosynthesis mechanism in purple-leaf kale, we focused on MYB transcription factors and cloned a gene (BoMYB) encoding an R2R3-MYB protein. We also showed that BoMYB was expressed in plant tissues in a tissue-specific manner. The gene was mainly expressed in purple stems and leaves but only slightly in roots, and thus we predicted that the expression of BoMYB was necessary for the synthesis of anthocyanins in kale.
Field observations showed that the stems and inner leaves of ‘D07’ were purple, outer leaves were green with purple veins, and roots were milky white (). The anthocyanin content was determined in each tissue, and the results showed () that the content was the highest in the stem epidermis; it was significantly higher in inner leaves than in outer leaves and was very low in the roots. To evaluate the expression levels of BoMYB in different organs of kale, gene expression analysis was performed by real-time PCR, and the data showed that BoMYB was expressed in ‘D07’ roots, stem epidermis, inner leaves, and outer leaves in a tissue-specific manner (). The gene showed the highest level of expression in the purple stem epidermis, followed by the leaf of the heart and old leaves, and showed the lowest level of expression in the root. Thus, biosynthesis of anthocyanin in kale may be related to the expression of BoMYB, and BoMYB may be involved in regulation of anthocyanin synthesis in kale.
Figure 4. Exterior colour (A), anthocyanin content (B), and BoMYB expression (C) in different tissues of kale.
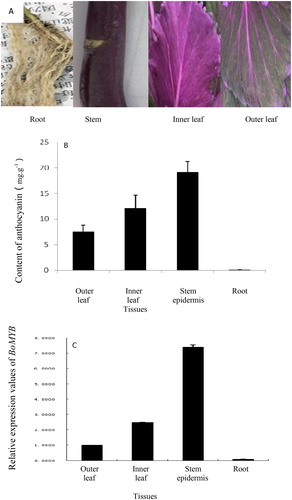
In addition to MYB transcription factors that positively regulate the synthesis of anthocyanins, some MYB transcription factors are involved in the inhibition of anthocyanin synthesis in plants. For example, the A. thaliana AtMYB4, MYBL2, and ICX1 transcription factors inhibit the expression of key genes involved in anthocyanin synthesis [Citation18, Citation35, Citation36]. When these transcription factors are not activated, the expression of anthocyanin genes is high, and the anthocyanin content significantly increases. Two continuously downregulated MYB genes (MnMYBJ and MnMYB4) were identified during development of mulberry fruits, and their expression was found to be negatively correlated with anthocyanin accumulation in mulberry [Citation37]. Overexpression of the SIMYB-ATV gene in tomato also inhibited anthocyanin production in tomato seedlings and plants, which can negatively interfere with the activation of the anthocyanin biosynthetic pathway mediated by the endogenous MYB–bHLH–WDR complexes [Citation38].
Genetic transformation of the pEarleyGate-BoMYB vector into Arabidopsis and detection of BoMYB expression
Arabidopsis, a model organism with physiological activities similar to those of kale, was used to further verify the function of BoMYB and demonstrate its ectopic expression. The pEarleyGate-BoMYB vector was transformed into Arabidopsis using the A. tumefaciens-mediated method. After T1 seeds were selected, they were screened on a medium containing 50 mg L−1 kanamycin, and then seedlings were transplanted to a greenhouse. The T2 generation was obtained by self-crossing. After self-crossing and screening, pure lines were obtained. Total RNA was extracted from eight transgenic A. thaliana seedlings, and fluorescence quantitative PCR was used to determine the relative expression levels of BoMYB in these transgenic lines (). BoMYB expression was detected in all eight strains, which were ranked in the following descending order of expression levels: cn7 > cn3 > cn5 > cn6 > cn1 > cn8 > cn2 > cn4. The transgenic plants were further cultivated to flowering and seed setting.
Morphological observation and anthocyanin content in transgenic plants
The morphological characteristics of wild-type plants and transgenic plants with low (cn4), medium (cn6 and cn1) and high (cn7) expression levels of BoMYB were evaluated. The transgenic plants showed differences in colour corresponding to the expression levels of BoMYB (). Plants from the line showing a high level of expression of BoMYB had dark purple leaves, stems, and roots; those from the lines showing medium expression had purple-coloured stem base and roots, and plants from the line with low expression of BoMYB showed a colour change only in the roots. The anthocyanin content was determined in the above four transgenic and wild-type plants. Wild-type Arabidopsis showed the lowest anthocyanin content of 1.03 mg g−1. The anthocyanin content in the transgenic plants was found to be in the following descending order: cn7 > cn6 > cn1 > cn4. The content of anthocyanin in cn7 was 23.33 mg g−1, i.e. 20-fold higher than that in wild-type Arabidopsis ().
Figure 6. Morphological characteristics (A) and anthocyanin content (B) in different Arabidopsis plants. Cn1, cn4, cn6, and cn7: different transgenic Arabidopsis plants; WT: Wild-type Arabidopsis plant.
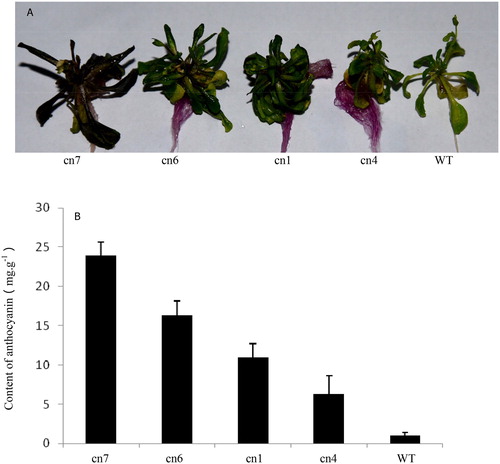
The results showed that BoMYB overexpression affected transgenic plants (cn7) of Arabidopsis, and that these transgenic plants could produce fertile seeds. Thus, BoMYB was predicted to be a MYB family transcription factor. Interestingly, the roots of kale are white, but when BoMYB was overexpressed in Arabidopsis, the roots showed a varying degree of purple, suggesting that the BoMYB gene was also expressed in Arabidopsis roots. The gene may activate the expression of certain key enzyme genes from the anthocyanin biosynthetic pathway, thus initiating anthocyanin synthesis and accumulation. The cloning of BoMYB and verification of its function using transgenic plants provide a foundation for related studies of anthocyanin biosynthesis in kale and technical support for changing the plant colour and breeding new colour varieties through a molecular breeding technology.
Conclusions
In this study, an R2R3-MYB transcription factor gene (BoMYB) from kale was obtained by homologous cloning. The quantitative analysis showed tissue specificity of BoMYB expression levels, which were correlated with the anthocyanin content in each tissue. The effects of the gene on anthocyanin synthesis and accumulation were verified by ectopic expression, and the results indicated that overexpression of the BoMYB gene increased the accumulation of anthocyanidins in transgenic Arabidopsis, which resulted in colour changes in the leaves, stem, and roots of transgenic Arabidopsis plants, showing various degrees of purple. Since BoMYB gene expression was related to the anthocyanin content in Arabidopsis, it is speculated that BoMYB is a gene for a MYB transcription factor that positively regulates the synthesis of anthocyanins in kale.
Disclosure statement
No potential conflict of interest was reported by the authors.
Additional information
Funding
References
- Wang YS, Tong Y, Li YF. High frequency plant regeneration from microspore-derived embryos of ornamental kale (Brassica oleracea L. var. acephala). Sci Hort. 2011;130:296–302.
- Zhao XS, Li MY, Zhang WL, et al. Establishment of high adventitious shoot regeneration system of ornamental Kale. Genomics Appl Biol. 2009;28:141–148.
- Zhang B, Yin MQ, Wen YY, et al. Expression features of the anthocyanin biosynthetic structural genes in kale. J Shanxi Agric Univ. 2014;42:313–316.
- Shen JZ, Zou ZW, Zhang XZ, et al. Metabolic analyses reveal different mechanisms of leaf color change in two purple-leaf tea plant (Camellia sinensis L.) cultivars. Hortic Res. 2018;5:7.
- Khoo HE, Azlan A, Tang ST, et al. Anthocyanidins and anthocyanins: colored pigments as food, pharmaceutical ingredients, and the potential health benefits. Food Nutr Res. 2017;61:1361779.
- Niu SS. Regulation of anthocyanin biosynthesis by MYB in Chinese Bayberry fruit and the mechanisms [Doctoral thesis]. Hangzhou (China): Zhejiang University; 2011.
- Dubos C, Stracke R, Grotewold E, et al. MYB transcription factors in Arabidopsis. Trends Plant Sci. 2010; 15:573–581.
- Shin DH, Choi M, Kim K, et al. HY5 regulates anthocyanin biosynthesis by inducing the transcriptional activation of the MYB75/PAP1 transcription factor in Arabidopsis. FEBS Lett. 2013;587:1543–1547.
- Kiferle C, Fantini E, Bassolino L, et al. Tomato R2R3-MYB proteins SlANT1 and SlAN2: same protein activity, different roles. PLoS ONE. 2015;10:e0136365. [cited 2019 Apr 05];
- Espley RV, Hellens RP, Putterill J, et al. Red colouration in apple fruit is due to the activity of the MYB transcription factor, MdMYB10. Plant J. 2007;49:414–427.
- Chagné D, Lin-Wang K, Espley RV, et al. An ancient duplication of apple MYB transcription factors is responsible for novel red fruit-flesh phenotypes. Plant Physiol. 2013;161:225–239.
- Chiu LW, Zhou XJ, Burke S, et al. The purple cauliflower arises from activation of a MYB transcription factor. Plant Physiol. 2010;154:1470–1480.
- Zhang Q, Xu ZD, Zhao K, et al. Isolation and biological function analysis of Aanthocyanin regulatory gene PmMYB1 from Prunus mume. Sci Silvae Sin. 2018; 54:67–75.
- Feng K, Xu ZS, Que F, et al. An R2R3-MYB transcription factor, OjMYB1, functions in anthocyanin biosynthesis in Oenanthe javanica. Planta. 2018;247:301–315.
- El-Sharkawy I, Liang D, Xu K. Transcriptome analysis of an apple (Malus × domestica) yellow fruit somatic mutation identifies a gene network module highly associated with anthocyanin and epigenetic regulation. J Exp Bot. 2015;66:7359–7376.
- Singh R, Low ET, Ooi LC, et al. The oil palm VIRESCENS gene controls fruit colour and encodes a R2R3-MYB. Nat Commun. 2014:30:5.
- Wang Z, Meng D, Wang A, et al. The methylation of the PcMYB10 promoter is associated with green-skinned sport in Max Red Bartlett pear . Plant Physiol. 2013;162:885–896.
- Dubos C, Le Gourrierec J, Baudry A, et al. MYBL2 is a new regulator of flavonoid biosynthesis in Arabidopsis thaliana. Plant J. 2008;55:940–953.
- Duan YJ, Zhang LG, He Q, et al. Expression of transcriptional factors and structural genes of anthocyanin biosynthesis in purple-heading Chinese cabbage. Acta Hortic Sin. 2012;39: 2159–2167.
- Jiang M, Chen XS, Li JZ. Cloning, expression and sequence analysis of anthocyanidin synthase gene BcANS in Brassica campestris var. purpurea. J Zhejiang Univ. 2011;37:393–398.
- Zhang B, Lu Y, Yin MQ, et al. Cloning and expression pattern analysis of purple cabbage COP1gene. J Shanxi Agric Univ. 2014;34:385–390.
- Ramsay NA, Glover BJ. MYB-Bhlh-WD40 protein complex and the evolution of cellular diversity. Trends Plant Sci. 2005;10:63–70.
- Zhang B, Hu ZL, Zhang YJ, et al. A putative functional MYB transcription factor induced by low temperature regulates anthocyanin biosynthesis in purple kale (Brassica Oleracea var. acephala f. tricolor). Plant Cell Rep. 2012;31:281–289.
- Niu SS, Xu CJ, Zhang WS, et al. Coordinated regulation of anthocyanin biosynthesis in Chinese bayberry (Myrica rubra) fruit by a R2R3 MYB transcription factor. Planta. 2010;231:887–899.
- Livak KJ, Schmittgen TD. Analysis of relative gene expression data using real-time quantitative PCR and the 2(-Delta Delta C(T)) Method. Methods. 2001;25:402–408.
- Jin H, Martin C. Multifunctionality and diversity within the plant MYB gene family. Plant Mol. Biol. 1999;41:577–585.
- Allan AC, Hellens RP, Laing W. MYB transcription factors that colour our fruit. Trends Plant Sci. 2008;13:99–102.
- Mehrtens F, Harald K, Pawel B. The Arabidopsis transcription factor MYB12 is a flavonol-specific regulator of phenylpropanoid biosynthesis. Plant Physiol. 2005; 138:1083–1096.
- Pattanaik S, Kong Q, Zaitlin D, et al. Isolation and functional characterization of a floral tissue-specific R2R3 myb regulator from tobacco. Planta. 2010;231:1061–1076.
- Deluc L, Bogs J, Walker AR, et al. The transcription factor Vv MYB5b contributes to the regulation of anthocyanin and proanthocyanidin biosynthesis in developing grape berries. Plant Physiol. 2008; 147:2041–2053.
- Yamagishi M, Shimoyamada Y, Nakatsuka T, et al. Two R2R3-MYB genes, Homologs of petunia AN2, regulate anthocyanin biosyntheses in flower tepals, tepal spots and leaves of Asiatic hybrid lily. Plant Cell Physiol. 2010; 51:463–474.
- Jaakola L, Poole M, Jones MO, et al. A SQUAMOSA MADS box gene involved in the regulation of anthocyanin accumulation in bilberry fruits. Plant Physiol. 2010;153:1619–1629.
- Shao WT, Liu Y, Han HQ, et al. Cloning and expression analysis of an anthocyanin-related transcription factor gene SmMYB in eggplant. Acta Hortic Sin. 2013; 40:467–478.
- Butelli E, Titta L, Giorgio M, et al. Enrichment of tomato fruit with health-promoting anthocyanins by expression of select transcription factors. Nat Biotechnol. 2008;26:1301–1308.
- Jin H, Cominelli E, Bailey P, et al. Transcriptional repression by AtMYB4 controls production of UV-protecting sunscreens in Arabidopsis. Embo J. 2000;19:6150–6161.
- Wade HK, Sohal AK, Jenkins GI. Arabidopsis ICX1 is a negative regulator of several pathways regulating flavonoid biosynthesis genes. Plant Physiol. 2003;131:707–715.
- Li J, Zhao AC, Liu CY, et al. Identification and function analysis of anthocyanin biosynthesis related MYB genes in Mulberry. Acta Bot Boreal-Occident Sin. 2016;36:1110–1116.
- Sara C, Pierdomenico P, Silvia G. The atroviolacea gene encodes an R3-MYB protein repressing anthocyanin synthesis in tomato plants. Front Plant Sci. 2018;9:830–846.