Abstract
This study was conducted to investigate the effect of quercetin on the triglyceride (TG) content through the peroxisome proliferator-activated receptor α (PPARα) signalling pathway in broiler hepatocytes. The trial included the following five groups: control, DMSO control, 5 mg/L quercetin, 10 mg/L quercetin and 20 mg/L quercetin. Compared with the control group, the PPARα protein content was significantly increased at 24 h in the groups that received quercetin at 5, 10 and 20 mg/L (p < 0.05), whereas the PPARα mRNA expression was significantly increased at 24, 48 and 72 h (p < 0.05). Compared with the control, the content of the long-chain acyl-CoA synthetase (ACSL), apolipoprotein A1 (ApoA1), liver fatty acid–binding protein (FABP) and TG in the 5-, 10- and 20-mg/L quercetin group was significantly increased at 24, 48 and 72 h (p < 0.05). Moreover, the L-FABP and ApoA1 mRNA expression levels were significantly increased at 24, 48 and 72 h (p < 0.05). Compared with the control, the ApoC3 protein content in the 5-mg/L quercetin group was significantly decreased at 72 h (p < 0.05). Furthermore, the very-low-density lipoprotein (VLDL) contents in the 5- and 20-mg/L quercetin groups were significantly decreased at 72 h (p < 0.05). In conclusion, quercetin enhanced lipid transportation and peroxidase β-oxidation regulated by PPARα and promoted lipid decomposition regulated by PPARα, but this did not occur in a dose-dependent or time-dependent manner. Therefore, quercetin decreased the TG content in broiler hepatocytes by promoting PPARα-regulated lipid decomposition, thereby reducing lipid deposition in broilers.
Introduction
In the modern intensive production of broilers, excessive fat deposition—especially in terms of abdominal fat—is a major concern in the poultry industry. Excessive fat deposition affects the quality of the carcass and increases the production cost. To address this issue, different feed additives have been used in broiler production to reduce fat deposition. Flavonoids belong to a group of natural substances that have a variable phenolic structure and are abundantly found in fruit, vegetables, tea and wine [Citation1]. Flavonoids exhibit antioxidative activity, which prevents cells from sustaining oxidative damage [Citation1] and improving immune function [Citation2], while enhancing safety. Grape pomace (GP) supplementation as an antioxidant source at doses of 30 g/kg [Citation3] and 60 g/kg [Citation4] decreases lipid oxidation in the meat of broilers. Moreover, many studies have shown that alfalfa flavonoid improves meat quality and antioxidative activity [Citation5], as well as decreases cholesterol in yolk, meat and serum [Citation6]. These results indicate that flavonoids may regulate lipid metabolism, and thus, affect meat quality.
Quercetin, a well-known flavonoid, possesses a wide array of biological effects that are considered beneficial to health, including anti-inflammatory, free radical scavenging, anticarcinogenic and antiviral activities [Citation1, Citation7–9]. More recently, beneficial effects on blood pressure and heart disease have been described [Citation10–12]. Quercetin has been reported to improve the diabetic status in animal models of both type 1 and type 2 diabetes [Citation13]. Another study conducted by Tang et al. [Citation14] found that quercetin treatment significantly reduced the cholesterol contents of the liver, heart, kidney and small intestine in rats. It is estimated that quercetin and its relevant derivatives account for nearly 60–70% of flavonoid intake in human daily life [Citation15]. The liver is the main site for lipid metabolism in chickens, and only a few studies have been conducted on the potential of quercetin as a feed additive improving the meat quality of broilers and the lipid metabolism mechanism [Citation16]. The purpose of the present experiment was to further study the role of quercetin in regulating the lipid metabolism in the hepatocytes of Arbor Acre (AA) broilers; the results will provide a theoretical basis for the application of quercetin in the production of broilers.
Materials and methods
Animals
AA broilers (16 days old) were purchased from Harbin Dongxu Incubation of Poultry Farms (Harbin, China).
Ethics statement
All procedures were approved by the Northeast Agricultural University Institutional Animal Care and Use Committee.
Materials
Williams’ E medium without phenol red, collagenase IV, insulin and dexamethasone were purchased from Sigma (St. Louis, MO). Penicillin G sodium, streptomycin sulphate, vitamin C, heparin sodium, bovine serum albumin, trypan blue and glutamine were purchased from Amresco (Washington, DC). Trypsin was purchased from Gibco (Los Angeles, CA), and phosphate-buffered saline (PBS) was purchased from Hyclone (Logan, UT). Foetal bovine serum was purchased from Sijiqing (Beijing, China). Albumin, urea nitrogen, alpha fetoprotein (AFP) and lactate dehydrogenase (LDH) kits were obtained from Jiancheng (Nanjing, Wuhan, China). Hepatocyte growth factor was obtained from Harbin High-tech (Harbin, Heilongjiang, China).
Experimental design
Hepatocytes were plated at a density of 5 × 105 cells per well (12-well plates), incubated with 15% foetal calf serum (FCS) Williams’ E medium without phenol sulfonephthalein at 37 °C in a 5%-CO2 incubator for 24 h. They were divided into five groups (), with six replicates in each group, and incubated in 5% FCS Williams’ E medium without phenol sulfonephthalein.
Table 1. Experimental design.
Isolation and culture of primary hepatocytes from AA broilers
Broiler hepatocytes were isolated from three male AA broilers at 16 days of age using a modification of the three-step perfusion method described by Seglen [Citation17], Chen et al. [Citation18] and Liu et al. [Citation19]. The liver was perfused via the hepatic portal vein with buffer A (5 mmol/L Na2EDTA·2H2O, 137 mmol/L NaCl, 3 mmol/L KCl, 3 mmol/L Na2HPO4, 10 mmol/L HEPES) at a flow rate of 20–30 mL/min for 30 min, followed by buffer B (100 mmol/L HEPES, 1.370 mmol/L NaCl, 30 mmol/L KCl, 30 mmol/L Na2HPO4·12H2O) at a flow rate of 20–30 mL/min for 15 min. The livers were picked and placed in a culture dish; they were then perfused with collagenase buffer C (10 mmol/L HEPES, 137 mmol/L NaCl, 3 mmol/L KCl, 3 mmol/L Na2HPO4·12H2O, 0.6 g/L CaCl2, 0.4 g/L collagenase IV; Sigma Inc., USA) at a flow rate of 20 mL/min for 15 min. The livers were dissected in collagenase buffer C and incubated for 10 min at 37 °C. After incubation, 50 mL of cold Williams’ E medium containing 2% FCS (Sijiqing Inc., China) was added, and the cell suspension was filtered through a 40 μm BD Falcon™ cell strainer (BD Biosciences), centrifuged for 2 min at 500 rpm and washed twice (Williams’ E medium and PBS, respectively).
The liver cells were purified by centrifugation through 60% Percoll™ (Amersham Biosciences) in PBS (Hyclone Inc., Logan, UT) at 391 g (Luxingyi Inc., China) for 10 min. Depositing cells were washed twice with PBS for 2 min at 500 rpm and pelleted with Williams’ E medium containing 15% FCS. Trypan (Gibco Inc., Billings, MO) blue excluding primary hepatocytes were counted, and the motility rate was calculated. Cells were seeded at 5 × 105 viable cells/mL in 15% Williams’ E medium (Sigma Inc.). Hepatocytes were plated at a density of 4.5 × 106 cells per dish (60-mm dishes) or 2.5 × 105 cells per well (24-well plates).
Identification of hepatocyte culture from AA broilers
Identification: The morphology of the hepatocytes was observed using an inverted microscope (IX83, OLYMPUS) and transmission electron microscope (JEM-F200, JEOL).
Markers of hepatocytes: The concentrations of albumen and alpha AFP were measured using a commercial chicken-specific enzyme-linked immunosorbent assay (ELISA) kit (Nanjing Jiancheng Bioengineering Institute, Nanjing, Jiangsu, China).
Function of hepatocytes: The concentration of urea nitrogen (BUN) and LDH were measured using a commercial chicken-specific ELISA kit (Nanjing Jiancheng Bioengineering Institute).
ELISA: Very-low-density lipoprotein (VLDL), triglyceride (TG), peroxisome proliferator-activated receptor α (PPARα), liver fatty acid–binding protein (L-FABP), (ApoA1), apolipoprotein A2 (ApoA2), apolipoprotein C3 (ApoC3), long-chain acyl-CoA synthetase (ACSL) and Acyl-CoA oxidase (ACO) were determined using an enzyme-labelled instrument and following the ELISA kit instructions (Nanjing Jiancheng Bioengineering Institute).
Quantitative real-time polymerase chain reaction (PCR): Total RNA in the broiler hepatocytes was extracted using a commercial kit (RNeasy Mini Kit, Qiagen, Dusseldorf, Germany), and the primers for the enzymes were synthesized by Sangon Biotech (Shanghai, China) based on a published sequence (). First-strand cDNA was synthesized from 1 μg of RNA using the Reverse Transcription System (Takara Bio, Inc., Dalian, Liaoning, China) according to the manufacturer’s instructions, and polymerase chain reaction (PCR) was performed using SYBR GREEN MASTERMIX (Takara Bio, Inc.). The PCR procedure was as follows: denaturation at 94 °C for 5 min and 30 cycles at 94 °C for 30 s, 55 °C for 30 s, and 72 °C for 1 min, followed by a 5-min extension at 72 °C.
Table 2. Primer pairs used in this study.
Statistical analysis: The data from this experiment were subjected to one-way analysis of variance (ANOVA) as a completely randomized design with five treatments and six replicates in each treatment. ANOVA was done using SPSS 20.0 software (IBM, 2011). Statistical significance was considered as p < 0.05.
Results and discussion
Isolation, culture and identification of hepatocytes from AA broilers
Under an inverted microscope, hepatocyte adherence was observed at 1 h post-inoculation, while total adherence of hepatocytes was observed at 4 h post-inoculation. There were elongated pseudopodia in the hepatocytes. The hepatocytes were polygonal, with flat and gracile cell bodies exhibiting increased volume. There were island links between the cells at 24 h post-inoculation (). The hepatocytes were integrated with each other in a sheet and there was island attachment at days 3 and 5 post-inoculation (). Aging, darkening and dropping off the wall were observed at day 6 post-inoculation (). Under an electron microscope, clear visualizations of the hepatocytes, nuclei and nuclear chromatin were observed. There were many wheel-shaped mitochondria, big lipid droplets and some glycogen rosettes at 24 h post-inoculation (). The hepatocyte outlines were clear, and their nuclei were located at the center, whereas the mitochondria were complete and visible at day 3 post-inoculation (). There were many lipid droplets in the hepatocytes, and the nuclei were pushed to the edge of the cell; however, the mitochondria were still complete at day 5 post-inoculation (. Hepatocyte disruption and mitochondrial deformation were observed at day 6 post-inoculation ().
Figure 1. Primary culture of broiler hepatocytes at 24 h (A,B), day 3 (C,D), day 5 (E,F) and day 6 (G,H). Magnification 200× (A,C,E,G) and 400× (B,D,F,H).
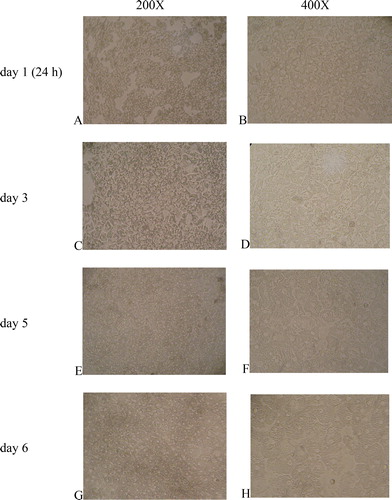
Figure 2. TEM (transmission electron microscopy) of primary broilers hepatocytes: cells (A,D,G,J), nucleoli (B,E,H,K) and mitochondria (C,F,I,L) at 24 h (A,B,C), day 3 (D,E,F), day 5 (G,H,I) and day 6 (J,K,L).
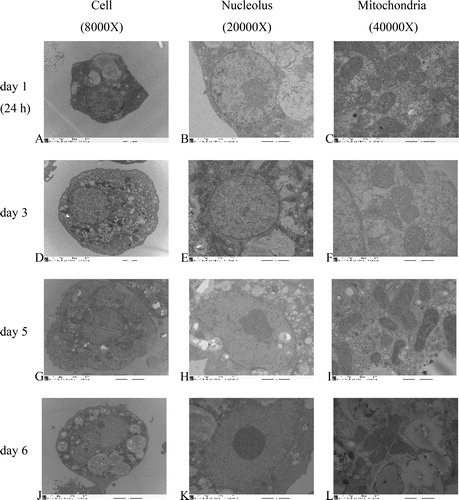
The albumin content in the medium was significantly higher at 36, 48, 60, 72, 84 and 96 h than it was at any other time (p < 0.05). Therefore, hepatocytes from 24 h to 96 h of culturing were strong and suitable for the metabolism study. The AFP content at 12–144 h of culturing was very low, and it was affected by the culture time (p > 0.05; ). The urea nitrogen content in the medium from 24 to 144 h of culturing was significantly higher than that in the 12-h culture (p < 0.05). The urea nitrogen content in the medium from 48 to 96 h of culturing was significantly higher than those at any other culture time (p < 0.05). Therefore, the hepatocytes from 24 to 96 h of culturing were suitable for further study. LDH activity was not detectable from 24 to 108 h; however, it was increased at 12 h and 120–144 h of culturing ().
Table 3. Content of albumin and alpha fetoprotein in hepatocytes medium.
Table 4. Urea nitrogen content and lactic dehydrogenase activity in hepatocytes medium.
Isolation and culture of hepatocytes are essential for the study of hepatocyte function in vitro. Isolation technology is the key step for obtaining sufficient hepatocytes with high vitality. Well-scattered hepatocytes with high purity and viability over 90% were obtained from broilers using the modified three-step perfusion method described by Seglen [Citation17], Chen et al. [Citation18] and Liu et al. [Citation19]. Moreover, the hepatocytes were highly differentiated non-proliferating cells. In fact, it is difficult for hepatocytes to survive for a long time in vitro. Therefore, serum-free Williams’ E medium was used as a culture medium in the present research to guarantee the longevity of the primary hepatocytes.
Cells were identified based on their morphology, biochemistry and histochemistry, among which, morphology is currently the simplest and most effective method. The results showed that the total adherence of hepatocytes occurred at 4 h post-inoculation and island links between cells occurred at 24 h post-inoculation, suggesting that hepatocytes isolated from the broiler liver were fit for further study. In addition, the biochemical functions of the hepatocytes were measured, which were evidenced by the aspects of metabolism. Normal hepatocytes secrete albumin and synthesize urea nitrogen, and AFP was a marker of embryonic hepatocytes; meanwhile, the release of intracellular enzymes is a marker of cellular damage, and LDH is more sensitive than other enzymes are [Citation20]. Thus, albumin, AFP, urea nitrogen and LDH were used for identifying hepatocytes’ biological activity. The present results suggested that hepatocytes from 24 to 96 h of culturing were strong and suitable for metabolism study.
Effects of quercetin on the lipid metabolism in hepatocytes from AA broilers
Lipids are mainly synthesized in the livers of chickens, where the fat mainly becomes TG, phospholipids and cholesterol (CHO), and the content of TG and CHO are key indicators of lipid metabolism. VLDL is responsible for transporting endogenous TG from the liver to other tissues. The results showed that DMSO did not affect the TG content (; p > 0.05); DMSO and quercetin at 5 mg/L did not affect the VLDL content at 24 h (; p > 0.05); and quercetin at 5, 10 and 20 mg/L significantly decreased the TG and VLDL content at 24, 48 and 72 h in hepatocytes compared with the control (p < 0.05). It is well known that high levels of lipids may result in the accumulation of hepatic TG and CHO, thereby causing fatty liver disease [Citation21].
Figure 3. Effects of quercetin on the content of TG (A) and VLDL (B) in broiler hepatocytes. Note:Mean values without a common letter are significantly different, p<0.05. Values are means ± SD (n = 6). The broiler hepatocytes were treated with DSMO and quercetin. Measurements were made by ELISA. Hepatocytes were incubated for 24 h, 48 h, 72 h either with medium as a control or with the addition of 5 mg/L (Group 1), 10 mg/L (Group 2) and 20 mg/L (Group 3) quercetin and DSMO.
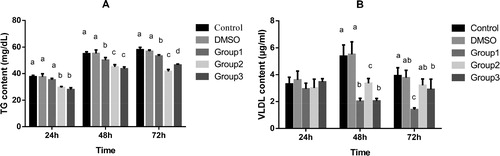
Hesperidin and naringin mainly regulate lipid metabolism by reducing the content of TG and CHO in the human liver [Citation22]. Quesada et al. [Citation21] reported that grapeseed procyanidin extract (GSPE) treatment slightly lowered the content of TG and CHO in rat livers. Moreover, Zhai et al. [Citation23] reported that quercetin decreased the serum TG content in tilapia (freshwater fish), which may be helpful for avoiding pathological changes of fatty liver. Kuipers et al. [Citation24] also reported that quercetin decreased the plasma TG level of 9-week-old mice. Furthermore, hawthorn leaf flavonoid extract significantly reduced serum CHO, TG and VLDL-CHO (VLDL-C) levels in mice [Citation25]. Naringenin supplementation significantly decreased the concentrations of low-density lipoprotein (LDL) and VLDL in ethanol-fed rats [Citation26]. Moreover, the results demonstrated that flavonoids of sea buckthorn fruits (FSBFs) had a quadratic effect on the contents of TG and VLDL in the livers of broilers [Citation11]. The present study suggested that quercetin decreased the blood lipid levels similarly to the effects of the other flavonoids.
Effects of quercetin on the regulation of the lipid metabolism in hepatocytes from AA broilers
PPARα is especially expressed in tissues with high lipid catabolic capacities, such as the liver, skeletal muscle and brown adipose tissue, and it plays a crucial role in lipid metabolism by regulating oxidation and the disintegration of fatty acids (FAs), lipid transportation, and the assembly of lipoproteins through modulating the transcription of its downstream genes [Citation27, Citation28]. PPARα knockout mice gain more adipose mass compared to wild-type animals, which may be due to local or systemic effects of PPARα [Citation29]. Hispidulin, a common flavone, acts as a direct PPARα agonist and exerts a hypolipidemic effect by enhancing the expression of genes involved in FA β-oxidation [Citation30]. Citrus flavonoids inhibit adipogenesis and decrease adiposity by regulating PPARα expression in vivo and in vitro [Citation31, Citation32]. Genistein protects against oleic-acid–induced steatosis with a complex mechanism, including an increase in PPARα expression [Citation33]. These reports indicate that flavonoids significantly increase PPARα expression.
Quercetin is one of the most abundant flavonoids [Citation34], so it probably affects PPARα expression. The results of the present study showed that quercetin significantly increased PPARα content and mRNA expression in a dose-dependent manner (). Our results are in line with those of previous studies, in which quercetin and other flavonoids increased PPARα expression in mice [Citation35], rats [Citation36] and humans [Citation37].
Figure 4. Effects of quercetin on the content (ELIZA) and mRNA expression (RT-PCR) of enzymes associated with lipid metabolism in hepatocytes. Content (A) and mRNA expression (B) of peroxisome proliferator activated receptor (PPARα); content (C) and mRNA expression (D) of liver-type fatty acid binding protein (L-FABP); content (E) and mRNA expression (F) of apolipoprotein-A-1(apoA1). Concentration of (G) apolipoprotein-A2 (ApoA2), (H) apolipoprotein-C3 (ApoC3), (I) long-chain acyl-CoA synthetases (ACLS) and (J) acyl-CoA oxidase (ACO) in broiler hepatocytes treated with DSMO and quercetin. Note: Hepatocytes were incubated for 24 h, 48 h, 72 h either with nothing added as a control or with the addition of 5, 10 and 20 mg/L quercetin and DSMO. The results of the relative quantification were expressed as 2-ΔΔCT and normalized to the control. The 2-ΔΔCT value of the treatment group was a multiple of the control (n = 6). Mean values without a common letter are significantly different, p<0.05. Values are means ± SD (n = 6).
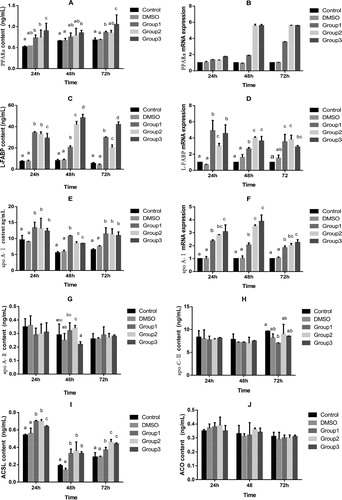
FABPs are found in all animal species, with high evolutionary conservation [Citation38]. FABPs (14–15 kDa) are responsible for transporting and storing FA in mitochondria [Citation39]. L-FABP is the first FABPs member that was isolated [Citation40], and it binds to a broad range of ligands, including FA [Citation41]. L-FABP is highly expressed in enterocytes and hepatocytes, with lower levels of expression in the kidney and colon [Citation42, Citation43]. Newberry et al. [Citation44] reported that L-FABP knockout leads to deprivation of carrying FA in mice. L-FABP promotes the transport of FA from the plasma membrane to the nucleus, which plays a role in regulating FA metabolism in rats [Citation41]. Wolfrum et al. [Citation45] also reported that L-FABP plays a role in FA signalling in hepatocytes. Platycodi radix (PR) supplementation effectively protects against alcohol-induced fatty liver and hepatotoxicity in rats [Citation46]. Our results showed that quercetin increased production of L-FABP in hepatocytes from AA broilers, and 20 mg/L quercetin supplementation worked best (). Meanwhile, together with the results for PPARα in this experiment, our findings were supported by Hostetler et al. [Citation47], who found that L-FABP directly interacted with PPARα.
Apolipoprotein is basically produced in the liver, as well as in the intestine, and it is secreted as a peptide [Citation48–50]. There are five classes of apolipoproteins (A to E); divided according to their structure and function in chickens, they are similar to those in mammals. ApoA1 and ApoA2 influence FA lipid transport by means of regulating transcription and activation, and they maintain the dynamic balance of lipid metabolism in the body. ApoC3 is a small protein on the surface of VLDL and LDL [Citation51]. Compared with the control, hepatic apoA1 mRNA and serum apoA1 in PPARα-deficient mice increased significantly [Citation52]. ApoC3 may lower the TG content by inhibiting the synthesis of TG, reducing the neutral lipid exchange between VLDL and high-density lipoprotein (HDL) and promoting LDL removal [Citation53]. In addition, Ooi et al. [Citation54] reported that apoC3 decreased the plasma TG levels by downregulating the apoC3 expression. Grapeseed procyanidins reduced apoC3 expression in rat liver [Citation55]. Quercetin increased apoA1 mRNA and gene promoter activity in HepG2 cells [Citation56]. The results of the present study showed that quercetin at 5, 10 and 20 mg/L significantly increased the content and mRNA expression of ApoA1 () at 24, 48 and 72 h (p < 0.05) and quercetin at 5 mg/L significantly decreased ApoC3 () content at 72 h in hepatocytes (p < 0.05). Our results further confirmed that quercetin enhanced FA transport in the liver, thereby decreasing the liver’s TG content.
β-Oxidation, a major pathway of FA metabolism, takes place in the mitochondria and peroxisomes through completely different enzymes in the cells [Citation57]. Mashek and Coleman [Citation52] reported that the specific process of FA oxidation is related to short-, medium- and long-chain FA, which undergo oxidation in the liver and muscles. The initial step in long-chain FA oxidation requires conversion of FA to acyl-CoA, a reaction catalyzed by ACSL [Citation58]. Oxidation of long-chain FA in the liver is impaired by ACSL1 deficiency [Citation59]. Patsouris et al. [Citation60] reported that ACSL is significantly decreased in PPARα knockout mice treated with a high-fat diet. Cao et al. [Citation61] showed that ACSL was transcriptionally upregulated by the cytokine oncostatin M (OSM) in HepG2 cells, accompanied by reduced cellular TG content and enhanced β-oxidation. In the present study, quercetin at 5, 10 and 20 mg/L significantly increased the ACLS content () at 24, 48 and 72 h in hepatocytes (p < 0.05). Our results showing that ACLS directly interacted with PPARα, together with the results on PPARα in this experiment, were consistent with previous findings [Citation62, Citation63].
ACO, a key enzyme of the peroxidase FA oxidation process, catalyzes the first step in the oxidation reaction of FA peroxidase. Oxidation of FA is primarily enhanced by increasing the expression of ACO in the mitochondria [Citation64, Citation65]. Supplementation of sea buckthorn extract in feed reduced fat deposition; altered PPARα, ACO and FAS mRNA expressions in fat tissue; and significantly influenced adipose deposition in swine [Citation10]. Costus speciosus ethanolic water (CSEW) significantly increased mRNA expression of ACO in hepatocytes and adipocytes, supporting the role of CSEW in improving FA oxidation [Citation66]. In addition, the mRNA expressions of ACO1 and ACO2 in genistein-treated cells were elevated approximately fivefold at 24 h compared with the control [Citation33]. Saneyasu et al. [Citation67] found that 4 h of fasting increased the mRNA expressions of PPARα, CPT1a and ACO in the liver, suggesting that FA oxidation-related genes were upregulated in the liver. In contrast, the expression of ACO, CD36 and PPARα was not affected by quercetin [Citation68]. Our results showed that quercetin did not affect the ACO content (, p > 0.05), which was consistent with the findings of Arias et al. [Citation68].
Conclusions
Our results showed that quercetin decreased the TG content through increasing the transport and β-oxidation of FA mediated by PPAR in hepatocytes. We hope that this study lays a solid foundation for future lipids metabolism work in vivo.
Disclosure statement
No potential conflict of interest was reported by the authors.
Additional information
Funding
References
- Panche AN, Diwan AD, Chandra SR. Flavonoids: an overview. J Nutr Sci. 2016 [cited 2019 Jun 13];5:e47.
- González-Gallego J, García-Mediavilla MV, Sánchez-Campos S, et al. Fruit polyphenols, immunity and inflammation. Br J Nutr. 2010;104:S15–S27.
- Brenes A, Viveros A, Goni I, et al. Effect of grape pomace concentrate and vitamin e on digestibility of polyphenols and antioxidant activity in chickens. Poult. Sci. 2008;87:307–316.
- Goni I, Brenes A, Centeno C, et al. Effect of dietary grape Pomace and vitamin e on growth performance, nutrient digestibility, and susceptibility to meat lipid oxidation in chickens. Poult. Sci. 2007;86:508–516.
- Ouyang KH, Xu MS, Jiang Y, et al. Effects of alfalfa flavonoids on broiler performance, meat quality, and gene expression. Can J Anim Sci. 2016;96:332–341.
- Wang Y, Cheng QM, Cao WX, et al. The effects of the extract mixture of alfalfa and perilla oil lowering serum lipid, protecting liver and aortic wall in rats. Parenter Enter Nutr. 2005;12:219–222.
- Formica JV, Regelson W. Review of the biology of quercetin and related bioflavonoids. Food Chem. Toxicol. 1995;33:1061–1080.
- Ross JA, Kasum CM. Dietary flavonoids: bioavailability, metabolic effects, and safety. Annu Rev Nutr. 2002;22:19–34.
- Simona B, Mohamed MAD, Delia MT, et al. Health benefits of polyphenols and carotenoids in age-related eye diseases. Oxid Med Cell Longev. 2019;2019:1–22.
- Perez-Vizcaino F, Duarte J, Jimenez R, et al. Antihypertensive effects of the flavonoid quercetin. Pharmacol Rep. 2009;61:67–75.
- Han JJ, Hao J, Kim CH, et al. Quercetin prevents cardiac hypertrophy induced by pressure overload in rats. J Vet Med Sci. 2009;71:737–743.
- Jung CH, Cho I, Ahn J, et al. Quercetin reduces high-fat diet-induced fat accumulation in the liver by regulating lipid metabolism genes. Phytother Res. 2013;27:139–143.
- Aguirre L, Arias N, Macarulla MT, et al. Beneficial effects of quercetin on obesity and diabetes. Open Nutraceuticals J. 2011;4:189–198.
- Tang Z, Gao W, Yang J, et al. Effect of quercetin on cholesterol distribution in rats. Acta Nutr Sin. 2013;35:172–175.
- Liu W, Zhang M, Feng J, et al. The influence of quercetin on maternal immunity, oxidative stress, and inflammation in mice with exposure of fine particulate matter during gestation. Int J Environ Res Public Health. 2017;14:592.
- Goliomytis M, Tsoureki D, Simitzis PE, et al. The effects of quercetin dietary supplementation on broiler growth performance, meat quality, and oxidative stability. Poult Sci. 2014;93:1957–1962.
- Seglen PO. Preparation of isolated rat liver cells. Methods Cell Biol. 1976;13:29–83.
- Chen L, Jiang Q, Zhu X, et al. Isolation and primary culture of adult chicken liver cells. J. Jau. 2008;30:385–389.
- Liu K, Huang XH, Gao H, et al. In situ separation and long-term culture of adult chicken hepatocytes. Chin J Vet Sci. 2009;9:1193–1196.
- Hamamoto I, Nemoto EM, Zhang S, et al. Assessment of hepatic viability during cold ischemic preservation. Transpl Int. 1995;8:6.
- Quesada H, Del-Bas JM, Pajuelo D, et al. Grape seed proanthocyanidins correct dyslipidemia associated with a high-fat diet in rats and repress genes controlling lipogenesis and VLDL assembling in liver. Int J Obes. 2009;33:1007–1012.
- Jung UJ, Kim HJ, Lee JS, et al. Naringin supplementation lowers plasma lipids and enhances erythrocyte antioxidant enzyme activities in hypercholesterolemic subjects. Clin Nutr. 2003;22:561–568.
- Zhai SW, Shi QC, Chen XH. Effects of dietary surfactin supplementation on growth performance, intestinal digestive enzymes activities and some serum biochemical parameters of tilapia (Oreochromis niloticus) fingerlings. Ital J Anim Sci. 2016;15:318–324.
- Kuipers EN, Van Dam AD, et al. Quercetin lowers plasma triglycerides accompanied by white adipose tissue browning in diet-induced obese mice. Int J Mol Sci. 2018;9:1786.
- Dong PZ, Pan LL, Zhang XT, et al. Hawthorn (Crataegus pinnatifida Bunge) leave flavonoids attenuate atherosclerosis development in apo E knock-out mice. J Ethnopharmacol. 2017;198:479–488.
- Jayaraman J, Namasivayam N. Effect of naringenin (Citrus Flavanone) on lipid profile in ethanol-induced toxicity in rats. J Food Biol. 2012;36:502–511.
- Mandard S, Muller M, Kersten S. Peroxisome proliferator activated receptor alpha target genes. Cell Mol. Life Sci. 2004;61:393–416.
- Roepstorff C, Halberg N, Hillig T, et al. Malonyl-CoA and carnitine in regulation of fat oxidation in human skeletal muscle during exercise. Am J Physiol Endocrinol Metab. 2005;288:133–142.
- Costet P, Legendre C, More J, et al. Peroxisome proliferator-activated receptor α- isoform deficiency leads to progressive dyslipidemia with sexually dimorphic obesity and steatosis. J Biol Chem. 1998;273:29577–29585.
- Wu XC, Xu J. New role of hispidulin in lipid metabolism: PPARα activator. Lipids. 2016;51:1249–1257.
- Cho KW, Kim YO, Andrade JE, et al. Dietary naringenin increases hepatic peroxisome proliferators-activated receptor α protein expression and decreases plasma triglyceride and adiposity in rats. Eur J Nutr. 2011;50:81–88.
- Kim GS, Park HJ, Woo JH, et al. Citrus aurantium flavonoids inhibit adipogenesis through the Akt signaling pathway in 3T3-L1 cells. BMC Complement Altern Med. 2012;12:31.
- Kim S, Shin HJ, Kim SY, et al. Genistein enhances expression of genes involved in fatty acid catabolism through activation of PPARα. Mol Cell Endocrinol. 2004;220:51–58.
- Somerset SM, Johannot L. Dietary flavonoid sources in Australian adults. Nutr. Cancer. 2008;60:442–449.
- Ding L, Liang XG, Zhu YD, et al. Icariin promotes expression of PGC-1α, PPARα, andNRF-1during cardiomyocyte differentiation of murine embryonic stem cells in vitro. Acta Pharm Sin. 2007;28:1541–1549.
- Xiong D, Deng Y, Huang B, et al. Icariin attenuates cerebral ischemia-reperfusion injury through inhibition of inflammatory response mediated by NF-κB, PPARα and PPARγ in rats. Int Immunopharmacol. 2016;30:157–162.
- Kobor I. M, Masumoto S, Akimoto Y, et al. Chronic dietary intake of quercetin alleviates hepatic fat accumulation associated with consumption of a Western-style diet in C57/BL6J mice. Mol Nutr Food Res. 2011;55:530–540.
- Zimmerman AW, Veerkamp JH. New insights into the structure and function of fatty acid-binding proteinsl. Cell Mol Life Sci. 2002;59:1096–1116.
- Olivares-Rubio HF, Vega-López A. Fatty acid metabolism in fish species as a biomarker for environmental monitoring. Environ Pollut. 2016;218:297–312.
- Chmurzynska A. The multigene family of fatty acid-binding proteins (FABPs): function, structure and polymorphism. J Appl Gene. 2006;47:39–48.
- Poirier H, Niot I, Monnot MC, et al. Differential involvement of peroxisome-proliferator activated receptors alpha and delta in fibrate and fatty-acid-mediated inductions of the gene encoding liver fatty-acid-binding protein in the liver and the small intestine. Biochem J. 2001;355:481–488.
- Hertzel AV, Bernlohr DA. The mammalian fatty acid-binding protein multigene family: molecular and genetic insights into function. Trends Endocrinol Metab. 2000;11:175–180.
- Gordon JI, Elshourbagy N, Low JB, et al. Tissue specific expression and developmental regulation of two genes coding for rat fatty acid binding proteins. J Biol Chem. 1985;260:1995–1998.
- Newberry EP, Xie Y, Kennedy S, et al. Decreased hepatic triglyceride accumulation and altered fatty acid uptake in mice with deletion of the liver fatty acid-binding protein gene. J Biol Chem. 2003;278:51664–51672.
- Wolfrum C, Borrmann CM, Borchers T, et al. Fatty acids and hypolipidemic drugs regulate peroxisome proliferator-activated receptors a- and g-mediated gene expression via liver fatty acid binding protein: a signaling path to the nucleus. PNAS. 2001;98:2323–2328.
- Kim HK, Kim DS, Cho HY. Protective Effects of platycodi radix on alcohol-induced fatty liver. J Biosci Biotechnol Biochem. 2007;71:1550–1552.
- Hostetler HA, Mcintosh AL, Atshaves BP, et al. L-FABP directly interacts with PPARα in cultured primary hepatocytes. J Lipid Res. 2009;50:1663–1675.
- Brunham LR, Kruit JK, Jahangir I, et al. Intestinal ABCA1 directly contributes to HDL biogenesis in vivo. J Clin Investig. 2006;116:1052–1062.
- Sliwkowski MB, Windmueller HG. Rat liver and small intestine produce proapo- lipoprotein A-I which is slowly processed to apolipoprotein A-I in the circulation. J Biol Chem. 1984;259:6459.
- Wu AL, Windmueller HG. Relative contributions by liver and intestine to individual plasma apolipoproteins in the rat. J Biol Chem. 1979;254:7316–7322.
- Mahley RW, Innerarity TL, Rall SC, et al. Plasma lipoproteins: apolipoprotein structure and function. J Lipid Res. 1984;25:1277–1294.
- Mashek DG, Li LO, Coleman RA. Long-chain acyl-CoA synthetases and fatty acid channeling. Future Lipidol. 2007;2:465–476.
- Hertz R, Bishara-Shieban J, Bar-Tana J. Mode of action of peroxisome proliferators as hypolipidemic drugs. Suppression of apolipoprotein C-III. J Biol Chem. 1995;270:13470–13475.
- Ooi EM, Barrett PH, Chan DC, et al. Apolipoprotein C-III: understanding an emerging cardiovascular risk factor. Clin Sci. 2008;114: 611–624.
- Del-Bas JM, Fernandez-Larrea J, Blay M, et al. Grape seed procyanidins improve atherosclerotic risk index and induce liver CYP7a1 and SHP expression in healthy rats. FASEB J. 2005;19:479–481.
- Haas MJ, Onstead-Haas LM, Szafran-Swietlik A, et al. Induction of hepatic apolipoprotein A-I gene expression by the isoflavones quercetin and isoquercetrin. Life Sci. 2014;110:8–14.
- Tocher DR. Metabolism and functions of lipids and fatty acids in teleost fish. Fish Sci. 2003;11:107–184.
- Mashek DG, Li LO, Coleman RA. Rat long-chain acyl-CoA synthetase mRNA, protein, and activity vary in tissue distribution and in response to diet. J Lipid Res. 2006;47:2004–2010.
- Li LO, Ellis JM, Paich HA, et al. Liver-specific loss of long chain acyl-CoA synthetase-1 decreases triacylglycerol synthesis and β-oxidation and alters phospholipid fatty acid composition. J Biol Chem. 2009;284:27816–27826.
- Patsouris D, Reddy JK, Muller M, et al. Peroxisome proliferator activated receptor alpha mediates the effects of high-fat diet on hepatic gene expression. Endocrinology. 2006;147:1508–1516.
- Cao A, Li H, Zhou Y, et al. Long chain acyl-coa synthetase-3 is a molecular target for peroxisome proliferator-activated receptor in hepg2 hepat- oma cells. J Biol Chem. 2010;285:16664–16674.
- Martin G, Schoonjans K, Lefebvre AM, et al. Coordinate regulation of the expression of the fatty acid transport protein and acyl-CoA synthetase genes by PPARα and PPARγ activators. J Biol Chem. 1997;272:28210–28217.
- Rakhshandehroo M, Hooiveld G, Muller M, et al. Comparative analysis of gene regulation by the transcription factor PPARα between mouse and human. Pub Lib Sci One. 2009;4:e6796.
- Peters JM, Cattley RC, Gonzalez FJ. Role of PPARα in the mechanism of action of the nongenotoxic carcinogen and peroxisome proliferator Wy-14,643. Carcinogenesis. 1997;18:2029–2033.
- Bonnefont JP, Djouadi F, Prip-Buus C, et al. Carnitine palmitoyltransferases 1 and 2: biochemical, molecular and medical aspects. Mol Asp Med. 2004;25:495–520.
- Muthukumaran P, Thiyagarajan G, Arun Babu R, et al. Raffinose from, Costus speciosus, attenuates lipid synthesis through modulation of PPARs/SREBP1c and improves insulin sensitivity through PI3K/AKT. Chem Biol Int. 2018;284:80–89.
- Saneyasu T, Shiragaki M, Nakanishi K, et al. Effects of short term fasting on the expression of genes involved in lipid metabolism in chicks. Comp Biochem Phys B. 2013;165:114–118.
- Arias N, Macarulla MT, Aguirre L, et al. Quercetin can reduce insulin resistance without decreasing adipose tissue and skeletal muscle fat accumulation. Genes Nutr. 2014;9:361.