Abstract
Sweet cherry is an important fruit crop in Bulgaria. One of the most common viruses of sweet cherry and other stone fruits in the world is Prune dwarf virus (PDV). Field surveys were conducted in 43 commercial and 3 collection sweet and sour cherry orchards at 10 locations in six regions of Bulgaria. Leaf samples from 2090 trees showing and not showing virus-like symptoms were tested by double antibody sandwich-enzyme linked immunosorbent assay (DAS-ELISA) for PDV infection. The average PDV infection level in sweet and sour cherry was 14.4%. In 92.4% of the trees, PDV caused single infection, whereas in 7.6%, it caused mixed infection with Prunus necrotic ringspot virus (PNRSV). Visual observations were made to connect the symptoms with PDV infection. There were PDV symptoms in 56.2% of infected trees whereas, 43.8% of the trees were asymptomatic. The coat protein (CP) sequences of 14 isolates from four regions were determined. The obtained sequences were 654 nucleotides (nts) in length, translated to 218 amino acids. Sequence comparisons showed 87%–100% to 93%–100% identity at nucleotide and amino acid level, respectively. Phylogenetic analyses using nucleotide and amino acid sequences of the CP genes of PDV isolates from Bulgaria and 38 cherry isolates retrieved from the GenBank database indicated the existence of three and two phylogenetic groups, respectively. No association between the phylogenetic grouping of the studied isolates and the symptoms of infected trees and/or the sampling-region was observed.
Introduction
Presently more than 30 viruses from several taxonomic groups have been reported to infect sweet (Prunus avium) and sour cherry (P. cerasus) [Citation1,Citation2]. Among these viruses Prune dwarf virus (PDV) is the most widespread virus with significant economic impact and yield losses of up to 100% in some commercial crops [Citation1,Citation3].
PDV belongs to subgroup 4 of genus Ilarvirus, family Bromoviridae, and has a tripartite single-stranded positive sense RNA genome [Citation3]. RNA1 and RNA2 encode the replicase subunits, whereas RNA3 is bicistronic and codes for the movement and capsid proteins (MP, CP) [Citation4].
Symptoms of PDV vary widely depending on climatic conditions, virus isolate, host species and cultivar [Citation1]. Trees infected with PDV generally have small and narrow or curled leaves, short internodes, and the leaves of sweet and sour cherry trees also have chlorotic ring spots [Citation5]. Trees with mixed infections of PDV with Prunus necrotic ring spot virus (PNRSV) have a reduced number of fruit buds and form leafless shoots [Citation6]. In addition to the transmission through infected propagating material [Citation7], PDV is also seed- and pollen-borne [Citation8].
Studies of PDV variability in various Prunus spp. including sweet and sour cherry from different geographic regions have mostly been focused on the CP gene [Citation4,Citation9–14] and more recently also on the movement protein (MP) gene [Citation13]. To date, PDV isolates from Bulgaria have not been analyzed molecularly.
Sweet cherry (P. avium) is an economically important stone fruit species in Bulgaria. The total area of sweet and sour cherry plantations amounts to 11,443 ha and 1672 ha, respectively [Citation15]. Cherry production increases annually and presently it ranks second after that of apple. Many new plantations were established during the last years in several regions of the country as South Eastern, South Central and South Western.
In this study, we conducted a visual survey to determine the symptoms typical of PDV infection in differently aged commercial orchards planted with sweet and sour cherry, as well in single home-growing trees at several locations in six regions of Bulgaria. Collected samples were serologically tested to determine the PDV infection rate. The CP genes of 14 isolates from trees with and without symptoms and representing different locations were sequenced. These sequences were phylogenetically analyzed against GenBank accessions of PDV sweet and sour cherry isolates from different geographical regions.
Materials and methods
Field survey and visual evaluation of leaf symptoms
Field observations and sample collections were carried out at several locations in six regions of Bulgaria from April to the end of June, 2018. We surveyed 43 orchards of sweet cherry and 3 orchards of sour cherry. The 43 sweet cherry orchards included 42 commercial ones and the collection at the Research Institute of Mountain Stockbreeding and Agriculture (RIMSA), Troyan. The three orchards of sour cherry included a commercial one and two collection orchards, at the Institute of Agriculture (IA), Kyustendil and at RIMSA, Troyan (). Additionally, single home-growing sweet and sour cherry trees from three locations were also inspected and sampled. Each tree in the field and each leaf sample in the laboratory were evaluated for symptoms. In total, 2090 samples were collected from trees showing and not showing virus-like symptoms. Samples were taken randomly from several different branches around the trees, placed in an ice bag and kept at 4 °C prior to testing. All positive samples were divided into portions and preserved at –70 °C and dried under CaCl2. Dried samples were stored at 4 °C.
Table 1. PDV infection rate of sweet cherry in the surveyed regions of Bulgaria.
Serological assays
Collected leaf samples were tested by double antibody sandwich-enzyme linked immunosorbent assay (DAS-ELISA) as described [Citation16]. Since PDV often causes mixed infections with PNRSV, the samples were tested for both viruses. Standard protocols were followed as given by the manufacturer (Loewe Phytodiagnostica Gmbh). Both, specific and conjugated antibodies were used in a quantity of 100 µL and incubated at 37 °C for 4 h. One hundred fifty microlitres of diluted 1:20 extracts (w/vol) were loaded in duplicate wells of a polystyrene microtiter plate and incubated overnight at 4 °C. Between each step the plate was washed three times with PBS-T (phosphate-buffered saline-Tween). Then 100 µL freshly prepared p-nitrophenylphosphate in substrate buffer (1 mg/mL) were loaded to each well. The plate was incubated at room temperature and photometric measurement was done at 405 mn after 2 h. Samples were considered as positive if their absorbance values were more than three times higher than the negative control.
Reverse transcription polymerase chain reaction (RT-PCR) analyses
The coat protein (CP) gene of 14 serologically detected PDV isolates from sweet cherry was amplified by a two-step RT-PCR method in a Quanta Biotech thermal cycler. The primer pair PDV-F (5′-GTGTAGAAAGAAGAGAAGTCCGACAAG-3′) and PDV-R (5’- ATCTAGAAGCAGCATTTCCAACTACGA-3‘) [Citation9] was selected. The expected amplified product was 874 bp long representing the whole CP open reading frame (ORF).
RNA was isolated from at least a few fresh and fully developed leaves by the use of a commercially available RNeasy Plant Mini extraction kit (Qiagen, Germany) and served as a template to generate single-stranded cDNA. Total nucleic extracts were dissolved in 50 µL of RNase-free water and stored at –70 °C until use.cDNA was synthesized using a smART Reverse Transcriptase kit (EURx, LtD.) in nuclease-free microcentrifuge tubes. The reaction mixture contained 2 µL of template RNA, 1 µL (10 mmol/L) of the specific antisense primer and 2.5 µL (2.5 mmol/L) dNTPs (containing 2.5 mmol/L of each dATP, dGTP, dCTP and dTTP) and sterile distilled water to a final volume of 12.5 µL. The mixture was heated to 65 °C for 5 min and quickly chilled on ice. Then 4 µL of 5× first strand buffer, 2 µL of 0.1 mol/L DDT (both, supplied with the kit), 0.5 µL of RNase OUT (40 units/µL) and 1 µL smART (200 U/μL) were added, mixed gently and incubated at 50 °C for 30 min. The reaction was terminated by heating at 85 °C for 5 min.
Secondly, PCR amplifications were performed, in which 1 μL of cDNA was mixed with 24 μL of the amplification mixture containing 2.5 μL of 10X reaction buffer (200 mmol/L Tris-HCL pH 8.4, 500 mmol/L KCL), 1.5 μL of MgCl2 (25 mmol/L), 2.5 μL dNTPs (2.5 mmol/L), 0.5 μL of each primer (10 mmol/L), 0.25 μL Taq DNA polymerase (5 U/μL; EURx, LtD.) and 16.25 μL RNase-free sterile water.
The following PCR programme was used: 94 °C for 2 min, then 40 cycles of 94 °C for 30 s, 50 °C for 30 s and 72 °C for 90 s, followed by 72 °C for 10 min.
Amplified products (5 µL each) were electrophoresed at 80–140 V for 1–1.5 h in 1.2% agarose gels in 40 mmol/L Tris-acetate and 1 mmol/L EDTA, pH 8.0 (TAE) and stained with GelRed dye (stock solution 10,000×) (Biotium) to confirm the expected size of the PCR products.
Phylogenetic and sequence identity analysis
RT-PCR products obtained as described above were purified using IllustraTM GFXTM PCR DNA purification system (Healthcare, UK) and sequenced directly by priming the sequencing reaction with the same oligonucleotides used for PCR (Eurofins, Germany). To obtain the nucleotide sequence containing the CP gene, the resulting sequences were compared and analyzed by Vector NTI software. The ORF encoding the CP gene and the deduced amino acid sequences were determined in Vector NTI. All sequences were compared to PDV sequences available in the GenBank database (https://www.ncbi.nlm.nih.gov/genbank/).
Multiple sequence comparison of the nucleotide and amino acid sequences of the CP genes of Bulgarian and other PDV isolates retrieved from the GenBank database were done by the AlignX module of Vector NTI software. Their percent identity was determined at nucleotide and amino acid level.
Phylogenetic analyses were performed using MEGA v.6 [Citation17]. The phylogenetic trees were constructed by the neighbor-joining (NJ) method using Kimura two-parameter. The statistical significance of the clusters and branches of the phylogenetic tree was tested by ‘bootstrap’ analyses with 500 replications. To determine the genetic relations among the Bulgarian PDV isolates and between them and cherry isolates from other geographic regions, we performed phylogenetic analyses based on nucleotide and amino acid sequences.
Results and discussion
Field surveys and symptoms evaluation
The field surveys covered locations with intensive growing of sweet cherry, such as Kyustendil, Petrich, Sliven and Plovdiv. To represent the different types of orchards, old (over 50 years old) and abandoned plantations (Kyustendil and Simitli), newly established ones (1 to 5 years old in Kyustendil, Petrich and Sliven) and middle aged (10–15 years old in Petrich, Sliven and Kyustendil) were included. The higher number of surveyed commercial sweet cherry orchards (42) reflects their predominance over the orchards of sour cherry in Bulgaria.
The visual observation of sweet cherry trees in the field and collected leaves in the laboratory revealed slight prevalence of symptomatic infection. Around 56.2% of collected samples with serologically proved infection of PDV showed symptoms, whereas 43.8% were without symptoms. Our results are in agreement with Yardimci and Culal-Klllc [Citation18], who observed PDV infection to be commonly associated with symptomatic cherry trees, whereas Perez-Sánchez et al. [Citation19] reported 65%–100% asymptomatic reaction to the virus. Several types of symptoms () were observed: A/chlorosis in the base of central and secondary veins; B/chlorotic spots and rings; C/mottling and wrinkling of the leaves; D/small necrotic spots; E/chlorotic and necrotic dark-brown spots and stripes and F/reddish necrosis and rupture. Such symptoms induced by PDV have been already described [Citation19–21]. It should also be noted that the symptoms described above were clearer at the beginning of the vegetation period (April, May and early June) and some of them were masked with the progression of the season.
Figure 1. Symptoms on the leaves of sweet cherry caused by PDV: (A) chlorosis in the base of central and secondary veins; (B) chlorotic spots and rings; (C) mottling and wrinkling of the leaves; (D) small necrotic spots; (E) chlorotic and necrotic dark-brown spots and stripes; (F) reddish necrosis and rupture (original A. Borisova and I. Kamenova).
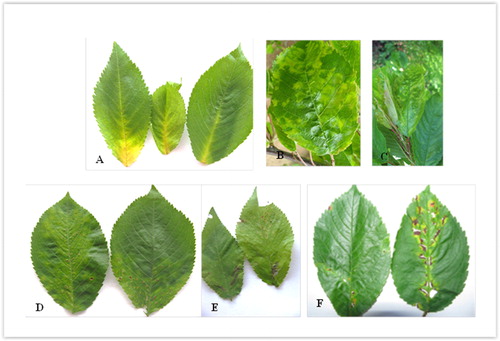
Serological evaluation
In total 1783 samples from sweet cherry trees were serologically analyzed for PDV and PNRSV. DAS-ELISA revealed that 275 samples (15.4%) were infected, of which 269 with PDV only and 6 ones with the two viruses (). The highest (27.0%) and lowest (6.0%) infection rates were determined in the commercial orchards of Kyustendil (Western) and Plovdiv (South Central) regions, respectively. The number of commercial orchards infected with PDV (29) was more than twofold higher than the number of orchards without PDV infection (13 orchards). Only one single home-grown tree was found infected.
In regard to sour cherry, 307 samples were tested and PDV was not detected in the single surveyed commercial orchard, or in single home-grown trees (results not shown). Only mixed infections of PDV and PNRSV at a rate of 5.2% (16 out of 307 tested samples) were found in the collection orchard of IA, Kyustendil.
According to the obtained data, the overall average infection level of PDV in sweet and sour cherry was 14.4% (291 infected out of 2090 tested). In 269 trees (92.4%) PDV caused single infection and in 22 trees (7.6%), mixed infection with PNRSV. This infection rate is similar to the infection rate (15.8%) of PDV in cherry in Bulgaria reported previously [Citation22]. In contrast, another study found 62% PDV infection rate of sweet cherry in Spain [Citation19]. Extended field studies in the countries of the Mediterranean region have revealed a high incidence of Ilarvirus infection (23.5%), with a prevalence of PDV (35.4%) in cherry [Citation4]. Mixed infections of two or more viruses are frequently detected on stone fruit species including cherry in Japan [Citation23], California [Citation24], Turkey [Citation18] and Mediterranean countries [Citation25].
To track PDV infection in sweet cherry orchards of different age, we provisionally grouped them into ‘young’, ‘middle-aged’ and ‘old’ orchards, respectively, aged from 1 to 5 years, from 6 to 10 years and over 10 years (one being even 65 years old). No significant difference among the orchards was detected, since the levels of infection were 20.7%, 21.1% and 24.2% in the ‘young’, ‘middle-aged’ and ‘old’ sweet cherry orchards, respectively. These results indicate that the introduction of PDV in the orchards has most likely occurred by infected plant materials.
Phylogenetic and sequence identity analysis
Nucleotide sequences obtained from the 14 isolates were compiled and trimmed to correspond to the CP gene and deposited to the GenBank database under accession nos. MK139682-MK139693 and MK392155-MK392156 (). These isolates were selected on the basis of there being single PDV infection in the tree, their geographic origin and the presence or lack of symptoms in infected trees. The obtained sequences were analyzed together with other PDV CP gene sequences from sweet and sour cherry isolates available in the GenBank database ().
Table 2. List of PDV isolates characterized in this study or available in the GenBank datebase.
The complete CP coding sequences of the 14 isolates analyzed in this study were with a length of 654 nts, translated to 218 amino acids. The nucleotide sequence identity ranged from 87% to 100%. The number of variable nucleotides was counted directly after alignment and the obtained consensus. This number ranged from 0 [among several isolates from the same location (isolates P128 and P138; B34, B25 and B87) but also between isolates from different regions, e.g. Iv2/1 (Western) and P113 (South Western)] to 13 (between isolates RS28 and BR115 from different regions).
The identity of translated amino acid sequences ranged from 93% (isolates D6 vs. BF105) to 100% (isolates B87 vs. B25 and B34; Iv2/1 vs. P113), as the number of variable amino acids ranging from 0 to 7. The reported nucleotide and amino acid sequence identity of 24 Slovak PDV isolates from cherry ranges from 85.8 to 99.1% and from 85.3 to 100%, respectively [Citation13]. Similarly, another study reported 84%–99% and 81%–100% sequence identity at the nucleotide and amino acid level, respectively, of the CP genes of PDV cherry isolates from Turkey and from other parts of the world [Citation14].
The phylogenetic tree constructed on the basis of the nucleotide alignments of 52 PDV isolates (14 from Bulgaria and 38 from other geographic regions) using the NJ method showed the formation of three groups (1, 2 and 3) related to each other and supported by high bootstrap values (). Group 1 was the largest one, consisting of 32 isolates from a number of countries such as Slovakia, Poland, Italy, Germany, the Czech Republic, Moldova, Israel, the USA and Canada. Twelve out of the 14 Bulgarian isolates fell into group 1, whereas the other two isolates (D6 BG and RS28 BG) fell into group 2. The isolates in group 1 subdivided into four clusters (1a, 1b, 1c and 1d) and the analyzed isolates from Bulgaria belonged to cluster 1a (six isolates), cluster 1c (four isolates) and cluster 1d (two isolates). Group 2 consisted of 13 isolates: eight ones from Turkey, two ones from Hungary, one from China and two of the isolates sequenced in this study. None of the analyzed Bulgarian isolates belonged to group 3, which consisted of seven isolates mainly from Hungary, Poland and Turkey. In general, the PDV isolates from Bulgaria did not form a homogeneous group within the tree and scattered in the two groups. A similar phylogenetic grouping (three groups and several subgroups) using the nucleotide sequences of cherry isolates from Turkey has been reported [Citation14]. Presence of four phylo-groups: 1/mixed; 2/cherry I; 3/cherry II and 4/almond from different Prunus spp. including plum, peach, sweet and sour cherry and almond without host-based clustering has been shown [Citation12]. Another study separated 24 phylogenetically analyzed PDV sweet cherry isolates from Slovakia into two major groups, both on the basis of CP and MP gene [Citation13].
Figure 2. Phylogenetic tree based on nucleotide sequences of the coat protein of PDV cherry isolates from Bulgaria and other isolates available in the GenBank database. The tree was produced using the NJ algorithm option of MEGA6 [17]. Bootstrap analysis of 500 replicates was performed. The scale bar shows the number of substitutions per nucleotide. The analyzed isolates from Bulgaria are in boldface and noted with the abbreviation BG.
![Figure 2. Phylogenetic tree based on nucleotide sequences of the coat protein of PDV cherry isolates from Bulgaria and other isolates available in the GenBank database. The tree was produced using the NJ algorithm option of MEGA6 [17]. Bootstrap analysis of 500 replicates was performed. The scale bar shows the number of substitutions per nucleotide. The analyzed isolates from Bulgaria are in boldface and noted with the abbreviation BG.](/cms/asset/c4324514-0d73-4dc4-a572-45899c11d863/tbeq_a_1637278_f0002_b.jpg)
The average nucleotide genetic diversity among the compared isolates from the three phylogenetic groups is shown in . The largest intra-group diversity was observed in group 2 (0.053 ± 0.005), represented by isolates from several countries, such as China, Turkey, Hungary and Bulgaria. The largest diversity among the three groups was observed between group 1 and group 3 (0.103 ± 0.011). The average divergence of 0.040 ± 0.004 within the Bulgarian isolates (not shown) was less than that reported for cherry isolates from Hungary (0.081 ± 0.007), Turkey (0.080 ± 0.005) and Slovakia (0.076 ± 0.076) [Citation13].
Table 3. Average pairwise distance (p-distance) within and between phylogenetic groups using nucleotide CP gene sequences of PDV isolates.
When phylogenetic analysis was conducted using amino acid sequences, the PDV isolates were divided into two groups (). Group 1 was the larger one, consisting of 45 isolates, whereas group 2 was formed by the same seven isolates which formed group 3 in the phylogenetic tree based on nucleotide sequences. The affiliation of each of the studied Bulgarian isolates to the respective cluster coincides with the observed clustering in the phylogenetic tree constructed based on the nucleotide sequences.
Figure 3. Phylogenetic tree based on amino acid sequences of the coat protein of PDV cherry isolates from Bulgaria and other isolates available in the GenBank database. The tree was produced using the NJ algorithm option of MEGA6 [17]. Bootstrap analysis of 500 replicates was performed. The scale bar shows the number of substitutions per amino acid. The analyzed isolates from Bulgaria are in boldface and noted with the abbreviation BG. Cluster numbering follows that in .
![Figure 3. Phylogenetic tree based on amino acid sequences of the coat protein of PDV cherry isolates from Bulgaria and other isolates available in the GenBank database. The tree was produced using the NJ algorithm option of MEGA6 [17]. Bootstrap analysis of 500 replicates was performed. The scale bar shows the number of substitutions per amino acid. The analyzed isolates from Bulgaria are in boldface and noted with the abbreviation BG. Cluster numbering follows that in Figure 2.](/cms/asset/fc05217a-206b-451b-b84e-9eec7c37f1ef/tbeq_a_1637278_f0003_b.jpg)
The calculated average amino acid diversity among the isolates studied in this work was 0.034 ± 0.007; among all the isolates from phylo-group 1 it was 0.057 ± 0.009 and among the isolates from phylo-group 2 it was 0.047 ± 0.009. The average amino acid diversity between groups 1 and 2 was 0.115 ± 0.020.
In general, the results from the study of the Bulgarian isolates did not show a correlation between the clustering of the isolates and the symptoms induced by them. In each of the three clusters (1a, 1c and 1d) there were isolates inducing and not inducing symptoms on infected trees. Similarly, in each of the two groups (group 1 and 2) there were isolates from different locations of the included regions of Bulgaria. Our results are in agreement with the reported absence of clear association between phylogenetic groups and geographical origins of PDV cherry isolates in Turkey [Citation14]. So far a clear divergence only between almond isolates and isolates from other Prunus hosts, possibly reflecting differences in agricultural practices has been observed [Citation10]
Conclusions
DAS-ELISA tests of sweet and sour cherry trees showed a moderate (14.4%) infection rate with PDV. As PDV is a pollen-transmissible virus, an increase of the infection rate in the surveyed orchards could be expected. The analyzed PDV isolates showed a relatively low degree of nucleotide sequence diversity. The phylogenetic grouping of the Bulgarian isolates into two and three phylo-groups at the nucleotide and amino acid level, respectively, concurs with reported phylogrouping of cherry isolates from different geographical regions. There was no relation between the phylogenetic grouping and the expressed symptoms, or with the geographical origin. In order to prevent and control PDV infection, serological and/or molecular methods such as RT-PCR are necessary to be applied as mandatory practice for assessment of the sanitary status of the planting material in the nurseries.
Acknowledgments
The authors thank all the owners of sweet and sour cherry plantations for their help and assistance during the field surveys.
Disclosure statement
The authors declare that they have no conflict of interest.
Additional information
Funding
References
- Nemeth M, Szalay-Marzsó L, Posnette A. Virus, mycoplasma and rickettsia diseases of fruit trees. Dordrecht (Netherlands): Academia Kiado; 1986.
- Myrta A, Savino V. Virus and virus-like diseases of cherry in the Mediterranean region. Acta Hortic. 2008;795:891–896.
- Cağlayan K, Ulubas-Serce C, Gazel M. Prune dwarf virus. In: Hadidi A, Barba M, Candresse T, Jelkmann W, editors. Virus and virus-like diseases of pome and stone fruits. St. Paul (MN): American Phytopathological Society; 2011. p. 199–205.
- Pallas V, Aparicio F, Herranz MC, et al. Ilarviruses of Prunus spp.: a continued concern for fruit trees. Phytopathology 2012;102:1108–1120.
- Šutic DD, Ford RE, Tosic MT. Virus diseases of plant virus diseases. In: Handbook of plant virus diseases. Boca Raton (FL): CRC Press; 1999.
- Kunze L. Prune dwarf virus. In: Smith IM, Dunez J, Lelliot RA, Philips DH, Archer SA, editors. European hand-book of plant diseases. Oxford (UK): Blackwell Scientific Publications; 1988. p. 15–17.
- Mink GI. Ilarvirus vectors. Adv Dis Vector Res. 1992;9:261–281.
- Mink GI. Pollen and seed-transmitted viruses and viroids. Annu Rev Phytopathol. 1993;31:375–402.
- Vaškova D, Pertzik K, Spak J. Molecular variability of the capsid protein of the Prune dwarf virus. Eur J Plant Pathol. 2000;106:573–580.
- Fonseca F, Neto JD, Martins V, et al. Genomic variability of Prune dwarf virus as affected by agricultural practice. Arch Virol. 2005;150:1607–1616.
- Boulila M. Molecular characterization of an almond isolate of Prune dwarf virus in Tunisia: putative recombination breakpoints in the partial sequences of the coat protein-encoding gene in isolates from different geographic origin. J Genet. 2009;48:411–421.
- Ulubas Serçe Ç, Ertunç F, Oeztuerk A. Identification and genomic variability of prune dwarf virus variants infecting stone fruit trees in turkey. J Phytopathol. 2009; 157:298–305.
- Predajňa L, Sihelská N, Benediková D, et al. Molecular characterization of prune dwarf virus cherry isolates from Slovakia shows their substantial variability and reveals recombination events in pdv RNA3. Eur J Plant Pathol. 2017;147:877–888.
- Ȫztürk Y, Cevik B. Genetic diversity in the coat protein genes of Prune dwarf virus isolates from sweet cherry growing in Turkey. Plant Pathol J. 2015;31:41–49.
- MAF. Annual agricultural report of Ministry of Agriculture, Food and Forest. Bulgaria (Bulgarian). Bulletin 341. Bulgaria: MAF; 2018.
- Clark MF, Adams AN. Characteristics of the microplate method of enzyme-linked immunosorbent assay for the detection of plant viruses. J Gen Virol. 1977;34:475–483.
- Tamura K, Stecher G, Peterson D, et al. MEGA6: molecular evolutionary genetics analysis version 6.0. Mol Biol Evol. 2013;30:2725–2729.
- Yardimci BCN, Culal-Klllc H. Detection of viruses infecting stone fruits in Western Mediterranean region of Turkey. Plant Pathol J. 2011;27:44–52.
- Sánchez RP, Corts RM, Benavides PG, et al. Main viruses in sweet cherry plantations of Central-Western Spain. Sci Agric (Piracicaba, Braz). 2015;72:83–86.
- Smith IM, Dunez J, Phillips DH, et al. European handbook of plant diseases. Oxford (UK): Blackwell Scientific; 1988.
- Massart S, Brostaux Y, Barbarossa L, et al. Interlaboratory evaluation of a duplex RT-PCR method using crude extracts for the simultaneous detection of Prune dwarf virus and Prunus necrotic ringspot virus. Eur J Plant Pathol. 2008;122:539–547.
- Milusheva S, Borisova A. The incidence of Prunus necrotic ringspot and Prune dwarf viruses in Prunus species in South Bulgaria. Biotechnol Biotechnol Equip. 2005;19:42–45.
- Isogai M, Aoyagi J, Nakagawa M, et al. Molecular detection of five cherry viruses from sweet cherry trees in Japan. J Gen Plant Pathol. 2004;70:288–291.
- Sabanadzovic S, Abou Ghanem-Sabanadzovic N, Rowhani A, et al. Detection of cherry virus A. cherry necrotic rusty mottle virus and little cherry virus 1 in California orchards. J Plant Pathol. 2005;87:173–177.
- Myrta A, Di-Terlizzi B, Savino V, et al. Virus diseases affecting the Mediterranean stone fruit industry: A decade of surveys. Option Méditerranéennes 2003; 45:15–23.
- Kalinowska E, Mroczkowska K, Paduch-Cichal E, et al. Genetic variability among coat protein of Prune dwarf virus variants from different countries and different Prunus species. Eur J Plant Pathol. 2014;140:863–868.