Abstract
To explore the colonization of endophytic nitrogen-fixing bacterium Klebsiella variicola in Penniseum sinense, pET28a and the enhanced green fluorescent protein (EGFP) were used as basic elements to construct a recombinant expression vector pET28a-Lac-EGFP with the addition of starter Lac in pUC18 vector using enzymatic digestion and splicing methods. The constructed recombinant vector was transformed into K. variicola GN02 cells with electroporation method and the colonization of EGFP-tagged K. variicola GN02 in the roots of Pennisetum sp. was observed using laser confocal scanning microscopy. The results of restriction enzyme digestion and sequencing analysis indicated that pET28a-Lac-EGFP was successfully constructed and the target gene was successfully transferred into K. variicola GN02 cells. Positive colonies with obvious green fluorescence were observed under ultraviolet (UV) light. Sodium dodecyl sulphate-polyacrylamide gel-electrophoresis (SDS-PAGE) showed that expression of the EGFP-labelled target protein was also successfully induced in K. variicola GN02 cells. Root scanner and confocal microscopy showed that inoculation of K. variicola GN02 strain was more conducive to the root growth of P. sinense and K. variicola GN02 mainly colonized the endothelial layer of Pennisetum sp. roots. However, colonization with a small amount of K. variicola GN02 led to its concentration in the epidermis, middle column and ducts. Our findings suggest that the endophytic nitrogen-fixing bacterium K. variicola accumulates in plant roots and is subsequently dispersed, aggregated or even colonized in susceptible plants. Furthermore, we provided a theoretical basis for the practical used of K. variicola GN02-based microbial fertilizers in P. sinense and gramineous plants.
Introduction
Endophytic diazotrophs are a group of microorganisms that colonize plant tissues and play an important role in plant growth and disease control. They also execute a series of biological functions such as nitrogen fixation, growth promotion and stress, disease and insect resistance in host plants by regulating their metabolites or signal transduction [Citation1, Citation2]. For example, numerous endophytic bacteria in plants protect them from the harsh environment and have a wide range of biological effects. The use of endophytic bacteria as biological agents or fertilizers during the biological pre-treatment of plants has broad application prospects [Citation3, Citation4]. However, exploring their colonization in plants is a prerequisite for developing production strategies [Citation5] and current detection methods include antibiotic labelling [Citation6], gene labelling [Citation7], isotope tracer [Citation8], DNA/RNA probe [Citation9] and immunological [Citation10] methods. Recently, with the continuing development of molecular biological technology, gene-labelling technology has been gaining increasing attention. Among these labelling methods, the enhanced green fluorescent protein (EGFP) exhibits strong fluorescence intensity, stable performance, convenient detection and high sensitivity and, therefore, is an ideal fluorescent marker that is currently widely used in molecular biological research [Citation11–13].
Pennisetum giganteum z.x.lin is a plant species introduced from South Africa in 2005–2007 by the Institute of Fungi of Fujian Agricultural and Forestry University. It belongs to the genus Pennisetum and is known as Giant JUNCAO. It is a perennial, erect, clumped grass with developed roots, strong stress resistance and high production [Citation14]. P. sinense is currently cultivated and has been improved by Fujian Agricultural and Forestry University. It is a typical C4 plant grown in extensive plantations in non-farm fields such as wasteland, saline-alkali land, dry land and sandy land. It is an energy or ecological grass with excellent application potentials because of its strong ecological control functions such as wind prevention and sand fixation [Citation15]. Klebsiella variicola GN02, isolated from the roots of mature P. sinense, is an endogenous bacterium with high nitrogen-fixing, phosphorus-dissolving, ammonia-producing, iron carrier-producing and antibiotic-promoting properties and, therefore, is a good strain source for fertilizers [Citation16]. K. variicola has few available vectors and the current major vector used in transformation experiments of Klebsiella-related strains is pET28a [Citation17]. This is a widely used prokaryotic expression vector [Citation18] because it can be expressed in gram-negative bacteria. contains a variety of restriction endonuclease sites and is only 5369 bp in size. It is expected to be useful in K. variicola-related studies and establishment of novel genetic operation systems.
In the present study, the expression vector pET28a and EGFP were used as basic elements with the addition of Lac promoter to construct a recombinant expression vector pET28a-Lac-EGFP. The expression of EGFP in K. variicola enabled the colonization of EGFP-tagged K. variicola GN02 in P. sinense to be observed using laser confocal scanning microscopy, which revealed the nitrogen-fixing and growth-promoting potentials of nitrogen-fixing bacteria in sugarcane. This finding expands the host scope of K. variicola GN02, and encourages exploration of the cross-talk interaction between nitrogen-fixing bacteria and host plants. Furthermore, this will provide a theoretical basis for the practical application of prepared K. variicola GN02-based microbial fertilizers in P. sinense and gramineous plants.
Materials and methods
Strains, media and solutions
K. variicola GN02 was isolated from the roots of P. sinense during the mature stage in our laboratory and was preserved in the China General Microbial Culture Collection Centre after identification and confirmation using 16S rRNA gene sequence and growth-promoting performance (Accession No: CGMCC 1.13619). The following media and solutions were used: Ashby nitrogen-free medium (mannitol 10 g/L, monopotassium phosphate (KH2PO4) 0.2 g/L, magnesium sulphate heptahydrate (MgSO4·7H2O) 0.2 g/L, sodium chloride (NaCl) 0.2 g/L, calcium sulphate dihydrate (CaSO4·2H2O) 0.1 g/L and calcium carbonate 9CaCO3) 5.0 g/L, pH 7.0–7.2), 50 mL lithium acetate-DDT (LiAc-DTT) solution (100 mmol/L LiAc, 10 mmol/L DDT, 0.6 mol/L sorbitol, 10 mmol/L Tris-HCl, pH 7.5, filtered and sterilized), 1 mol/L sorbitol (9.1 g sorbitol dissolved in distilled water to a final volume of 50 mL, sequentially filtered and sterilized), 2× loading buffer (20 mmol/L Tris-HCl pH 8.0, 100 mmol/L DTT, 2% sodium dodecyl sulphate [SDS], 2% glycerol and 0.016% bromophenol blue) and phosphate-buffered sale (PBS) sulphate buffer solution (pH 7.4, NaCl 8 g/L, KCl 0.2 g/L, Na2HPO4 1.42 g/L and KH2PO4 0.27 g/L).
Major reagents
The plasmid PET28a (Kan+), pEGFP-N1, pUC18 and Escherichia coli DH5α were all provided by Wuhan Transduction Biotechnology Co. Ltd (China). PCR reagents; bacterial genomic, DNA, and plasmid extraction kits, and DNA agarose recovery kit were purchased from Tiangen Company (Beijing). DNA marker, restriction enzymes (EcoRI, XhoI and XbaI), alkaline phosphatase (CIAP), T4 DNA ligase and the protein marker were purchased from TakaRa Company (Dalian). Primers were designed and synthesized by Sangon Biotech (Shanghai) Co. Ltd. (China) Ultrapure grade isopropyl β-D-1-thiogalactopyranoside (IPTG) was purchased from Beijing Solarbio Technology Co. Ltd. (China).
Construction of pET28a-Lac-EGFP recombinant vector
The EGFP gene fragment was amplified using the pEGFP-N1 plasmid as a template and the primers were designed using the Primer version 5.0 software, Primers F1, R1 (). The PCR system (25 μL) consisted of 4 μL DNA template, 2 μL each of the forward (F) and reverse (R) primers (each 10 μmol/L), 1 μL TransStrat FastPfu DNA polymerase (5 U/μL), 10 μL 10× buffer, 5 μL and dNTPs mixture (2.5 mmol/L). The PCR conditions were as follows: 95 °C for 3 min; 35 cycles at 94 °C for 30 s, 65 °C for 30 s, 72 °C for 30 s; and 72 °C for 5 min (ABI-2720, Applied Biosystems Inc., USA). The amplified product was separated by electrophoresis using 1% agarose gel (90 V, 45 min) and recovered using a DNA purification kit.
Table 1. Primers used for cloning.
The pET28a-EGFP vector was constructed using the purified EGFP gene product as the template by inserting cleavage sites of the restriction enzymes (EcoRI (5′-GAATTC-3′) and XhoI (5′-CTCGAG-3′)). The PCR system and parameters were consistent with those described above (primers F2 and R2, ). The amplified EGFP fragment and plasmid pET28a carrying restriction enzyme sites were digested with EcoRI and XhoI, respectively, and then purified. The double-digested EGFP fragment was ligated with pET28a using T4 DNA Ligase and the product was transformed into competent E. coli DH5α cells. After cultivation, positive colonies in the Lysogeny broth (LB) medium were selected for plasmid extraction to obtain the pET28a-EGFP plasmid, which was further identified using enzyme digestion with EcoRI and XhoI.
The Lac fragment was amplified using PCR with the pUC18 vector as a template and the PCR-amplified primer was introduced into XbaI restriction site (primers F3 and R3, ). The PCR-amplified product was purified and digested using XbaI and after digestion the Lac fragment was ligated to the CIAP-treated vector pET28a-EGFP with the same restriction sites as the T4 DNA ligase to obtain the recombinant vector pET28a-Lac-EGFP. Then, restriction enzyme digestion and sequence analysis were used to verify the successful construction of the vector. The PCR mixture (10 μL) was as follows: 5 μL 2× TSINGKE Master Mix, 1 μL bacterial solution template, 3 μL each of primers F and R (each 10 μmol/L), 3.2 μL ddH2O. The PCR conditions were as follows: 94 °C for 3 min; 35 cycles at 94 °C for 30 s, 55 °C for 45 s, 72 °C for 2 min; and 72 °C for 5 min. The flow chart for the construction of the recombinant vector pET28a-Lac-EGFP is shown in .
Figure 1. Construction of recombinant expression vector pET28a-Lac-enhanced green fluorescence protein (EGFP).
Note: EGFP fragment was cloned from pEGFP-N1 and ligated into pET28a vector to construct the intermediate vector pET28a-EGFP. pUC18 vector was used as the template to introduce Lac promoter to obtain recombinant vector pET28a-Lac-EGFP.
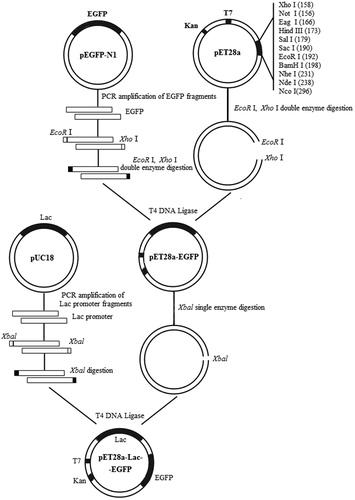
Recombinant vector transformation in K. variicola GN02
The activated K. variicola GN02 was inoculated into antibiotic-free LB medium, cultured at 30 °C with shaking at 180 rpm to obtain an optical density value at 600 nm (OD600) of 0.5. The bacterial cells were collected, washed with LiAc-DTT solution three times and then 2–3 mL pre-cooled 1 mol/L sorbitol was added. Approximately 80 µL of the cell suspension was transferred into a 1.5 mL centrifuge tube, followed by the addition of 10 μL recombinant plasmid pET28a-Lac-EGFP (10–20 μg, concentration > 1 μg/μL) with sequential slow pipetting and mixing. Then, the mixture was transferred to the pre-cooled 0.2 cm electrotransfer tube on ice and the transformation method of Wu et al. [Citation19] was performed. The electric shock treated bacterial cells were transferred to a 1.5 mL centrifuge tube and 100 µL of the inoculum was applied to LB solid medium containing 50 μg/mL kanamycin and the plate was cultured at 30 °C. Single colonial PCR amplification of the Lac promoter fragment was used to identify positive transformants using plasmid pET28a-Lac-EGFP as the positive control.
EGFP expression in K. variicola GN02
For the IPTG induction, positive transformants were selected, streaked onto the Kan-resistant LB plates, which were cultured at 30 °C overnight for colony growth and then, 20 μL IPTG was added to the plate evenly for the induction of EGFP expression. The plate was then incubated at 30 °C for colony growth and after 6 h, the colonial morphology was observed in the dark room to detect the fluorescence.
Low temperature overnight induction was performed on the positive transformants that were inoculated into LB liquid medium and shaken to an OD600 of approximately 0.6. The bacterial solution was divided into two volumes of 500 and 1000 μL and labelled as non-induced and induced bacterial solution, respectively. An aliquot of 10 μL of IPTG was added to the induced-bacterial solution. Both bacterial solutions were cultivated at 19 °C with shaking at 180 rpm overnight. The next day, the bacterial cells were collected by centrifuging at 65,000 rpm for 5 min and the supernatant was removed. Then, 20 μL PBS was added to bacterial cell pellets and thoroughly mixed. Finally, the bacterial cells were mixed with 10 μL 2× loading buffer, boiled for 10 min in a boiling water bath and then, the samples were centrifuged at 12,000 rpm for subsequent SDS-PAGE.
Expression and colonization of EGFP-tagged K. variicola GN02 in P. sinense roots
The obtained EGFP-tagged K. variicola GN02 strain was inoculated into the Ashby nitrogen-free medium containing Kanamycin (50 µg/mL) and IPTG (40 µg/mL) at a concentration of 1.5%, cultured at 30 °C for 24 to 48 h with shaking at 180 rpm and centrifuged at 12,000 rpm at 4 °C for 5 min to collect the cells. The cells were resuspended in an equal volume of pH 7.4 PBS buffer. The clonal seedlings of P. sinense were sown, sterilized with 75% ethanol and 2% NaClO, washed with sterile water 3–4 times and placed in a sterile beaker for rooting cultivation. The cultured shoots were immersed in PBS for 30 min (cell concentration 1 × 108 CFU/mL), and then returned to the sterile water beaker for further culturing. The non-inoculated cultured seedlings were used as the control. After 14 days of co-cultivation, the cultured seedlings in both groups were harvested. Some roots were cleaned with distilled water and observed under a root scanner (Model EPSON EU-88, Epson Ltd., China), while others were surface disinfected, washed with sterile water and placed it in IPTG (40 μ g/mL) solution for 2 h. Immediately after that, the roots were cut into slices, which were observed under a laser confocal scanning microscope (Model Leica TCS SP8, Leica Instruments, Germany) with dual-channel scanning at excitation wavelengths of 488 and 568 nm as the green and red channels, respectively [Citation20]. The laser intensity was adjusted to 30% and the photomultiplier tubes PMT1 and PMT2 (red and green channel, filters 605DF32 and 522DF32, respectively) received fluorescence signals simultaneously with the gain adjusted to ensure a moderate red and green image contrast. Furthermore, the fluorescence signal was accumulated to the peak and the output image was stored. Another 1 g roots with surface disinfection and sterile water cleaning were grinded to homogenate in sterilizing mortar and 9 mL of PBS buffer (pH 7.4) was added to the blend. The mixture was diluted with aseptic water gradient and then plated into the Ashby nitrogen-free medium containing Kanamycin (50 µg/mL) and IPTG (40 µg/mL). After 24 h of inverted culture at 30 °C, the colonization quantification of GFP labelled K. variicola GN02 strains in per gram root was counted.
Results and analysis
Transformation of recombinant vector pET28a-Lac-EGFP in K. variicola GN02
The constructed recombinant vector pET28a-Lac-EGFP shown in is a closed loop structure of 6138 bp. The recombinant vector was transformed into K. variicola GN02, the DNA was extracted and identified using XbaI digestion. After 1.0% agarose gel electrophoresis, the expected fragment of 750 bp was obtained (). In addition, the recombinant vector pET28a-Lac-EGFP was bi-directionally determined using the internal sequence of the EGFP gene as the sequencing primer. The results showed that the inserted sequence of the Lac gene and EGFP-encoding sequence were included in the recombinant vector (), indicating the successful construction of vector pET28a-Lac-EGFP.
Figure 2. Map of the constructed recombinant vector pET28a-Lac-enhanced green fluorescence protein (EGFP).
Note: It is a closed 6138-bp long loop structure and includes a T7 promoter, an inserted Lac fragment, a kanamycin-resistant fragment, an EGFP-encoding region and enzyme cleavage sites such as EcoRI, XhoI and XbaI.
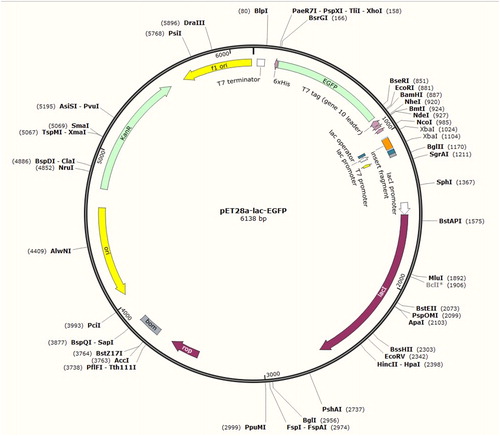
Figure 3. Electrophoresis plot of vector pET28a-Lac-EGFP (a) and a nucleotide sequence map of pET28a-Lac-enhanced green fluorescence protein (EGFP) vector (b).
Note: (a) Lane 1-3, products digested by restriction enzymes for recombinant plasmid pET28a-Lac-EGFP; Lane M, DNA marker. (b) Underlined portion is fragment of Lac promoter with 74 bp size; the fragment highlighted in yellow is ribosome-binding site (RBS); EGFP-encoding sequence is highlighted in purple; starter code is ATG.
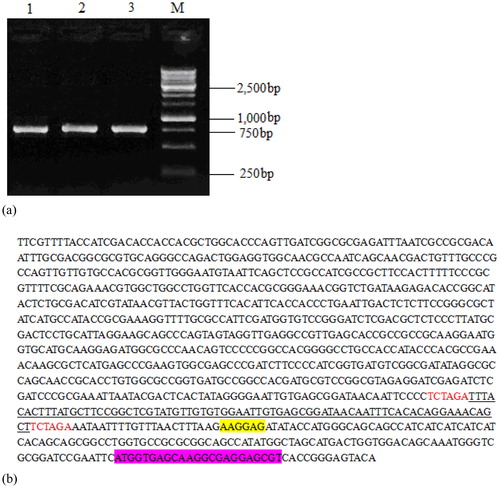
Expression of EGFP in IPTG-induced K. variicola GN02
Fifteen colonies grown on the LB solid medium containing kanamycin were random selected and all verified as positive clones using PCR amplification of the Lac promoter fragment. The selected positive transformants were plated to obtain a single colony, which had a morphology (mucous-like, round), colour and size consistent with the basic morphological characteristics of K. variicola. After induction by IPTG, the green fluorescent colonies could be observed under UV light in a dark room (). As shown in , the SDS-PAGE result was consistent with the predicted protein size of 26.9 kDa after the overnight induction of positive transformants in cultivation broth at low temperature, suggesting the successful expression of EGFP in K. variicola GN02 following IPTG induction.
Figure 4. Morphological observation of positive transformants under natural light (a) and under UV light in a dark room (b,c) without IPTG induction (b) and under IPTG induction (c).
Note: (a) Colonial morphology of positive transformants under natural light conforming to basic morphological characteristics of Klebsiella variicola. (b) No obvious green fluorescence without IPTG induction under the UV lamp in the dark. (c) Green fluorescence in positive transformants under IPTG induction, observed under the UV lamp in the dark.
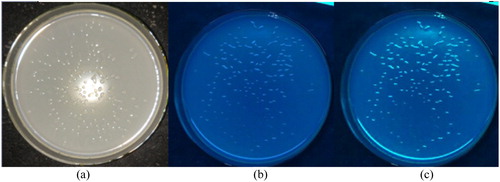
Expression and colonization of EGFP-tagged K. variicola GN02 in P. sinense roots
The hydroponic test results of P. sinense roots with and without inoculation of nitrogen-fixing bacteria are shown in . After inoculation with K. variicola GN02 strain 5 days later, the aggregation pattern around the roots of P. sinense was examined (). After 14 days of culture, the roots of both groups of P. sinense plants were examined using a root scanner. The results showed that, compared to the samples not inoculated with nitrogen-fixing bacteria, inoculation with the K. variicola GN02 strain effectively increased the number of roots, especially fibrous roots and their root surface area of 20.85 ± 2.31cm2 was significantly higher (p < 0. 05) than that of the control group (11.78 ± 1.56 cm2) (). Thus, inoculation with the K. variicola GN02 strain more effectively increased the absorption area of plant nutrients and promoted plant growth. The cross-section and longitudinal section of the roots of P. sinense with and without inoculation of nitrogen-fixing bacteria were examined using a laser confocal scanning microscope (). The results showed that numerous green fluorescent dots were observed in root tissues inoculated with nitrogen-fixing bacteria () and no green fluorescent dots were observed in root tissues without inoculated nitrogen-fixing bacteria (), indicating that K. variicola GN02 carrying EGFP penetrated the roots and colonized the endothelial layer of P. sinense. The cross-sectional analysis revealed that K. variicola GN02 was mainly distributed in the endothelial layer of the roots, had low distribution in the epidermis and middle column and even colonized the ducts. In Ashby nitrogen-free medium, containing Kanamycin (50 µg/mL) and IPTG (40 µg/mL), the total number of colonies with obvious green fluorescence was found to be 6.5 × 106 CFU/g, which means that K. variicola GN02 could colonize P. sinense and maintain a certain population density under the condition of inoculation with limited bacteria (1.0 × 108 CFU/g).
Figure 6. Promotion of Pennisetum sinense growth induced by Klebsiella variicola GN02 after 5 days. Note: (a) Red arrow indicates where bacteria congregated. (b) Inoculation with K. variicola GN02 strain effectively increased the number of roots, especially fibrous roots, and their root surface area, p < 0. 05.
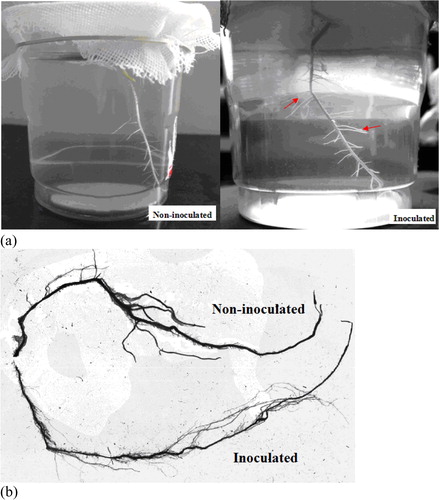
Figure 7. Observation of Pennisetum sinense with and without inoculation of EGFP-labelled Klebsiella variicola GN02 under laser confocal scanning microscope. Longitudinal (a) and cross cuts (b) of green fluorescent dots in P. sinense roots inoculated with EGFP-labelled K. variicola GN02. Longitudinal (c) and cross cuts (d) of P. sinense roots not inoculated with EGFP-labelled K. variicola GN02.
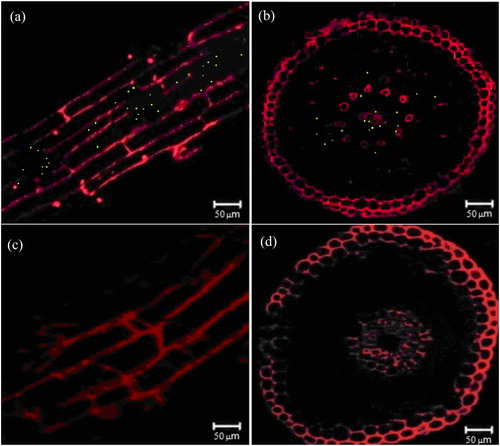
Final remarks
Nitrogen is an important elemental constituent of proteins and nucleic acids and is extremely important for animals, plants and microorganisms. Endophytic nitrogen-fixing bacteria present in plants for a long time can co-develop with the host to provide certain nitrogen compounds for the host plant, promote the absorption of various nutrients by plant roots and indirectly promote plant growth [Citation21, Citation22]. As ideal plant growth-promoting bacteria, nitrogen-fixing bacteria not only have high nitrogenase activity, but also require successful colonization of the roots of host plants [Citation23, Citation24]. Therefore, exploring the colonization pattern of nitrogen-fixing bacteria in different P. sinense could enhance our understanding of the invasion pathway, colonization and mode of action of nitrogen-fixing bacteria in host plants. This would contribute to uncovering nitrogen-fixing mechanisms and growth-promoting potential of nitrogen-fixing bacteria in plants and also provide a theoretical basis for practical production.
EGFP is a variant of GFP with a fluorescent intensity 35 times that of GFP [Citation25, Citation26]. Its high fluorescent intensity and stable expression enable convenient observation under a laser confocal or fluorescence microscope to track the invasion and specific colonization sites of nitrogen-fixing bacteria in plant tissues. Saravanakumar et al. [Citation27] ligated EGFP to the vector pCAMBIA1300 to monitor the expression of recombinant vector pCAMBIA1300-eGFP in Trichoderma harzianum CCTCC-RW0024 using a fluorescence microscope and the recombinant strain successfully colonized maize roots. Previous studies have shown that the T7 promoter directly links the EGFP gene to vector pET28a. Although it can be successfully transformed into the host K. variicola, it cannot be recognized, which may be due to the low expression efficiency of EGFP. Therefore, in the present study, based on the linkage of the EGFP gene to vector pET28a, the recombinant expression vector pET28a-Lac-EGFP was constructed by introducing the Lac promoter of pUC18 vector. The practice has confirmed that the constructed recombinant vector successfully transferred the target EGFP into the host K. variicola GN02 and the target gene protein was detected in the bacteria, indicating that the constructed recombinant vector was an expression vector suitable for expressing EGFP in K. variicola GN02.
Recently, numerous scholars have studied the colonization mechanisms and sites of endophytic nitrogen-fixing bacteria in different plants. The results showed that different types of nitrogen-fixing bacteria have different colonization rules, such as the invasion of Acetobacter diazotrophicus from the root tips and lateral roots of host plants [Citation28] and the colonization of Azospirillum brasilense in the periplasmic space between root surface and cortex of rice [Citation29]. Furthermore, the interstitial invasion of Klebsiella oxytoca from the lateral roots of rice and the epidermal cells of root epithelial cells and the inward and longitudinal migration through the cortical cell gaps differ [Citation30]. This study showed that the endophytic nitrogen-fixing K. variicola accumulated in the roots of P. sinense, promoted their growth and mainly colonized the endothelium of P. sinense roots with low amounts in the epidermis, middle column and ducts. We speculated that after accumulating in the plant roots, the colonizing K. variicola GN02 strain may penetrate the endothelial layer of the roots and continue to penetrate the middle column through the cortical cell gaps. Therefore, nitrogen-fixing K. variicola can colonize suitable plants in a dispersed or aggregated manner without fully spreading in the plants. The root cortex, epidermis and middle column are common colonization locations of the nitrogen-fixing K. variicola. However, inoculation and colonization by nitrogen-fixing K. variicola at different growth stages and of different growing parts (roots and leaves) in P. sinense and other gramineous plants still need to be further explored.
Conclusions
In this study, the recombinant vector pET28a-Lac-EGFP was successfully constructed using splicing restriction enzymes, and it had a closed loop structure of 6138 bp that included a T7 promoter, a Lac fragment, a kanamycin-resistant fragment, an EGFP-encoding region, and corresponding enzyme cleavage sites of EcoRI, XhoI and XbaI. The recombinant vector was transformed into K. variicola GN02 cells with electroporation method, and the screened colonies were PCR amplified to obtain a Lac promoter fragment with a full length of 74 bp. In addition, low amounts were expressed and the green fluorescent positive colonies were examined under UV light in a dark room. Green fluorescence and SDS-PAGE showed that the EGFP-target protein was successfully expressed in K. variicola GN02. Inoculation of K. variicola GN02 strain more effectively enhanced P. sinense root growth, and confocal microscopy showed that the EGFP-tagged K. variicola GN02 was mainly concentrated in the endothelial layer of the roots. Furthermore, low distribution was observed in the epidermis and middle column, as well as the ducts. These observations indicate that after accumulating in the plant roots, the endophytic nitrogen-fixing K. variicola were dispersed or aggregated, and, therefore, could even colonized suitable plants.
Disclosure statement
No potential conflict of interest was reported by the authors.
Additional information
Funding
References
- Stone JK. An overview of endophytic microbes: endophytism defined. Microb Endophytes. 2000;57:3–29.
- Liaqat F, Eltem R. Identification and characterization of endophytic bacteria isolated from in vitro cultures of peach and pear rootstocks. Biotech. 2016; [cited 2019 Jun 19];6:e120. [8 p.].
- Camelo-Rusinque M, Moreno-Galván A, Romero-Perdomo F, et al. Development of a liquid fermentation system and encystment for a nitrogen-fixing bacterium strain having biofertilizer potential. Rev Argent Microbiol. 2017;49:289–296.
- Bhattacharjee RB, Singh A, Mukhopadhyay SN. Use of nitrogen-fixing bacteria as biofertiliser for non-legumes: prospects and challenges. Appl Microbiol Biotechnol. 2008;80:199–209.
- Cocking EC, Stone PJ, Davey MR. Symbiosome-like intracellular colonization of cereals and other crop plants by nitrogen-fixing bacteria for reduced inputs of synthetic nitrogen fertilizers. Sci China C Life Sci. 2005;48:888–896.
- Reiter B, Pfeifer U, Schwab H, et al. Response of endophytic bacterial communities in potato plants to infection with Erwinia carotovora subsp. Atroseptica. Appl Environ Microbiol. 2002;68:2261–2268.
- Sessitsch A, Jjemba PK, Hardarson G, et al. Measurement of the competitiveness index of Rhizobium tropici strain CIAT899 derivates marked with the gusA gene. Soil Biol Biochem. 1997;29:1099–1110.
- Njoloma J, Tanaka K, Shimizu T, et al. Infection and colonization of aseptically micropropagated sugarcane seedlings by nitrogen-fixing endophytic bacterium, Herbaspirillum sp. B501 gfp1. Biol Fertil Soils.. 2006;43:137–143.
- Gupta G, Panwar J, Jha PN. Natural occurrence of Pseudomonas aeruginosa, a dominant cultivable diazotrophic endophytic bacterium colonizing Pennisetum glaucum (L.) R. Br. Appl Soil Ecol. 2013;64:252–261.
- Gao JL, Sun JG, Li Y, et al. Numerical taxonomy and DNA relatedness of tropical rhizobia isolated from Hainan province, China. Int J Syst Bact. 1994;44:151–158.
- Krzyzanowska D, Obuchowski M, Bikowski M, et al. Colonization of potato rhizosphere by GFP-Tagged Bacillus subtilis MB73/2, Pseudomonas sp. P482 and Ochrobactrum sp. A44 shown on large sections of roots using enrichment sample preparation and confocal laser scanning microscopy. Sensors. 2012;12:17608–17619.
- Quecine MC, Silva TM, Carvalho G, et al. A stable Leifsonia xyli subsp. xyli GFP‐tagged strain reveals a new colonization niche in sugarcane tissues. Plant Pathol. 2016;65:154–162.
- Akbari DL, Akbari LF. Detection of GFP expression and colonization of wheat by two endophytic bacteria tagged with GFP Gene. Int J Pure App Biosci.. 2017;5:844–848.
- Wang YS, Zhou BF. The sociological value of invention and development of Juncao technology. Fujian Agr Univ (Philos Soc Sci). 2006;9:1–5. Chinese.
- Tong XY, Zheng SH, Ye DP, et al. The research progress and prospect of Pennisetum sp. (Giant Juncao) planting working mechanism. AMM. 2014;641–642:1093–1098.
- Lin BS, Wang LF, Zhang KL, et al. Study on effect of interaction between endophytic diazotrophs from Pennisetum sp. and P-releasing bacteria and manufacture of their compound bacterial fertilizer. Jiangsu Agr Sci. 2018;46:218–283. Chinese.
- Kim B, Lee S, Park J, et al. Enhanced 2,3-butanediol production in recombinant Klebsiella pneumoniae via over expression of synthesis-related genes. J Microbiol Biotechnol. 2012;22:1258–1263.
- Ai-Jia JI, Ning XB. Construction and expression of prokaryotic expression vector pET28a-EGFP. J Microbiol. 2011;31:69–73.
- Wu N, Matand K, Kebede B, et al. Enhancing DNA electrotransformation efficiency in Escherichia coli DH10B electrocompetent cells. Electron J Biotechnol. 2010;13:0–22.
- Slattum G, Mcgee KM, Rosenblatt J. P115 RhoGEF and microtubules decide the direction apoptotic cells extrude from an epithelium. J Cell Biol. 2009;186:693–702.
- Abdalla MA, Matasyoh JC. Endophytes as producers of peptides: an overview about the recently discovered peptides from endophytic microbes. Nat Prod Bioprospect. 2014;4:257–270.
- Feng Y, Shen D, Song W. Rice endophyte Pantoea agglomerans YS19 promotes host plant growth and affects allocations of host photosynthates. J Appl Microbiol. 2006;100:938–945.
- Kovtunovych GL, Lar OV, Kordyum VA, et al. Enhancing the internal plant colonization rate with endophytic nitrogen-fixing bacteria. Biopolym Cell. 1999;15:300–305.
- Cocking EC. Endophytic colonization of plant roots by nitrogen-fixing bacteria. Plant Soil. 2003;252:169–175.
- Heim R, Cubitt AB, Tsien RY. Improved green fluorescence. Nature. 1995;373:663–664.
- Wang Z, Chen Y, Li S, et al. Successful construction and stable expression of an anti-CD45RA scFv–EGFP fusion protein in Chinese hamster ovary cells. Protein Express Purif. 2014;94:1–6.
- Saravanakumar K, Li Y, Yu C, et al. Effect of Trichoderma harzianum on maize rhizosphere microbiome and biocontrol of Fusarium Stalk rot. Sci Rep. 2017;7:1771–1771.
- Bellone CH, De Bellone SDVC, Pedraza RO, et al. Cell colonization and infection thread formation in sugar cane roots by Acetobacter diazotrophicus. Soil Biol Biochem. 1997;29:965–967.
- Baciliojimenez M, Aguilarflores S, Mvdel V, et al. Endophytic bacteria in rice seeds inhibit early colonization of roots by Azospirillum brasilense. Soil Biol Biochem. 2001;33:167–172.
- An QL, Kuang BJ, Mu XJ, et al. Nitrogen-fixing bacteria Klebsiella oxytoca SA 2 in rice root colonization and expression of nitrogenase. Chin J Prog Nat Sci. 1999;9:1262–1268.