Abstract
The aim of the present study was to investigate whether miRNA (miRNA or miR)-125a-5p regulates angiogenesis in hepatocellular carcinoma (HCC) via VEGF-A. Reverse transcription-quantitative polymerase chain reaction was used to determine the expression of miRNA or mRNA. Western blotting was used to determine the expression of target protein in cells, while ELISA was employed to measure the secretion of target protein by cells. Dual luciferase reporter assay was performed to identify the direct interaction between miRNA and the 3’-untranslated region of its target mRNA. CCK-8 assay was carried out to evaluate the proliferation of cells. MTT assay was performed to examine the migration of cells. The results showed that decreased expression of miR-125a-5p in HHC HepG2 cells was potentially related to the increased expression of VEGF-A. The expression of miR-125a-5p regulated VEGF-A expression and secretion of HepG2 cells. miR-125a-5p could bind with the 3’-UTR seed region of VEGF-A mRNA to inhibit its expression. miR-125a-5p increased the expression and secretion of VEGF-A by HepG2 cells, which subsequently promoted the proliferation of HUVECs by increasing the phosphorylation of Akt. miR-125a-5p regulated the migration of HUVECs via the VEGF-A/VEGFR2/Akt signalling pathway, which was affected by the expression and secretion of VEGF-A by HepG2 cells. Thus, the present study demonstrates that overexpression of miR-125a-5p inhibits the growth and angiogenesis of HCC by regulating the expression of VEGF-A.
Introduction
Hepatocellular carcinoma (HCC), which accounts for 80–90% of primary liver cancers, is one of the most common malignant tumours [Citation1]. There are many factors that are involved in tumourigenesis and development, and they are associated with high proliferation, anti-apoptosis, high invasiveness and angiogenesis [Citation2]. In recent years, the mechanism of proliferation, infiltration and metastasis of HCC cells has been widely studied, but there are still few studies on angiogenesis in HCC.
Angiogenesis refers to the recruitment of endothelial cells, smooth muscle cells and other cells by various means on a mature vessel trunk, or the extension or bifurcation of the vessel trunk and the formation of new vascular branches through the proliferation and migration of cells [Citation3]. Angiogenesis alters the permeability, migration, proliferation, extension and tubule formation of endothelial cells [Citation4]. In the meantime, there are also many cytokines that antagonize angiogenesis, including endostatin, angiostatin and pigment epithelium-derived factor [Citation5–7]. Numerous researches on related drugs are also reported. VEGF-A is a potent cytokine that promotes angiogenesis [Citation8], but the clinical practice of anti VEGF-A monoclonal antibody has not been satisfactory in recent years, suggesting that the current research and understanding of tumour angiogenesis is not enough.
MicroRNA (miRNA or miR) is a class of single chain small-molecule RNA with 22–24 nucleotides [Citation9]. miRNA is involved in regulating various physiological and pathological processes, such as cell proliferation, differentiation, apoptosis and metabolism [Citation10]. miR-125a is located on chromosome 19q13, and its mature form includes miR-125a-3p and miR-125a-5p [Citation11]. miR-125a is down-regulated in various human cancers, including breast cancer, ovarian cancer, lung cancer and medulloblastoma [Citation11]. The expression of miR-125a-3p is associated with tumour size and tumour invasion of HCC [Citation12]. Survival analysis shows that miR-125a-5p is an independent prognostic factor in HCC patients, which might be related to its target gene HER-2 [Citation13–15]. The expression of miR-125a-5p is significantly decreased in HCC tissues, and the down-regulation of miR-125a-5p is associated with invasion and metastasis of HCC [Citation16]. Overexpression of mi-125a-5p can inhibit the proliferation, migration and invasion of HCC cells, which may be related to the down-regulation of mTOR [Citation16]. However, the mechanism of action of miR-125a in the occurrence and development of HCC is not clear yet. It is reported that miR-125a-5p can regulate VEGF-A and is related to invasion and metastasis of HCC [Citation17]. VEGF-A is considered to be the most potent angiogenic factor, which binds to its ligand, VEGF receptor-2 (VEGFR-2), on the surface of endothelial cells. In the present study, we examine whether miR-125a-5p regulates angiogenesis in HCC via VEGF-A.
Materials and methods
Cells
THLE-2, SMMC7721, HepG2 and Huh-7 cell lines (Cell Bank, Chinese Academy of Sciences, Shanghai, China) were cultured in DMEM medium supplemented with 10% fetal bovine serum (FBS), 100 IU mL−1 penicillin and 100 IU mL−1 streptomycin under 37 °C and 5% CO2 (all reagents from Thermo Fisher Scientific, Waltham, MA).
One day before transfection, log-phase HepG2 cells (2 × 105) were seeded onto 6-well plates, and cultured in antibiotics-free DMEM medium until reaching 70% confluency. Transfection was performed using 1.5 µL miR-125a inhibitor (20 pmol µL−1; inhibitor group) or mimics (20 pmol µL−1; mimics group) (GenePharma, Shanghai, China) and 1.5 µL Lipofectamine 2000 (Thermo Fisher Scientific, Waltham, MA) according to the manufacturer’s manual.
Reverse transcription-quantitative polymerase chain reaction (RT-qPCR)
Total RNA was extracted from tissues or cells with TRIzol reagent (Thermo Fisher Scientific, Waltham, MA) according to the manufacturer’s instructions. For quantitative real-time PCR analysis, 2 μg of total RNA was reverse-transcribed using the Applied Biosystems High-Capacity cDNA Reverse-Transcription Kit. SYBR Green reactions using SYBR Green PCR Master Mix (Promega, Fitchburg, WI) were assembled along with 500 nmol L−1 primers according to the manufacturer’s instructions (C1000 thermal cycler CFX96 Real-Time System; Bio-Rad, Hercules, CA). PCR primers were as follows: VEGF-A, 5′-CGAAGTGGTGGTCATGGATG-3′ (forward) and 5′-TTCTGTATCAGTCTTTCCTGGTGAG-3′ (reverse); GAPDH, 5′-AGGTCGGAGTCAACGGATTTG-3′ (forward) and 5′-GTGATGGCATGGACTGTGGT-3′ (reverse). The reaction protocol was: initial denaturation at 95 °C for 3 min; 95 °C for 10 s and 60 °C for 25 s (40 cycles); elongation at 72 °C for 10 s (C1000; Bio-Rad, Hercules, CA, USA). The 2-ΔΔCt method was used to calculate the relative expression of VEGF-A mRNA against GAPDH.
Western blotting
Tissue and cell lysates were prepared using RIPA buffer (Beyotime, Shanghai, China) supplemented with proteinase inhibitor cocktail, phenylmethanesulfonyl fluoride and phosphorylase inhibitor (Roche, Rotkreuz, Switzerland). Equal amounts of proteins were loaded into sodium dodecyl sulfate-polyacrylamide gels and transferred onto polyvinylidene difluoride filter membrane. Immunoblotting was performed using the following primary antibodies: VEGF-A antibody (Cell Signaling Technology, Danvers, MA), Akt antibody and p-Akt antibody (Cell Signaling Technology, Danvers, MA) and β-Tubulin (Cwbiotech, Beijing, China).
Enzyme-linked immunosorbent assay (ELISA)
Forty-eight hours after transfection, the old medium was replaced with serum-free medium and cells were incubated for another 24 h. Then, the medium was collected to detect VEGF-A concentration by ELISA assay (Abcam, Cambridge, UK) according to the manufacturer's protocol. Thereafter, serum-free medium containing different concentrations of VEGF-A (HepG2-VEGF-A-medium) was placed on standby at −80˚C for epithelial cell proliferation and tube formation assays.
Bioinformatics
Bioinformatics prediction is a powerful tool for the study of the functions of miRNAs. We used miRanda (http://www.microrna.org/microrna/home.do), TargetScan (http://www.targetscan.org), PiTa (http://genie.weizmann.ac.il/pubs/mir07/mir07_data.html), RNAhybrid (http://bibiserv.techfak.uni-bielefeld.de/rnahybrid/) and PICTA (http://pictar.mdc-berlin.de/) to predict target genes that might be regulated by miR-125a-5p, and found that VEGF-A was a potential target gene of miR-125a-5p.
Dual luciferase reporter assay
Mouse VEGF-A gene promoter region was amplified using mouse genomic DNA and inserted into pGL3 control vector (Promega, Fitchburg, WI). HepG2 cells were cultured in 24-well plates and cotransfected with luciferase reporter construct (0.2 mg well−1), miR-125a-5p (0.2 mg well−1), or pcDNA4-myc-His (control) with the Profection kit (Promega, Fitchburg, WI), according to the manufacturer’s protocol. A renilla luciferase expression vector, pCMA-RL-TK, was used as an internal control to adjust for the transfection efficiency. After 48 h, cells were harvested and measured using the Dual-Luciferase Reporter assay system (Promega, Fitchburg, WI). All luciferase assay experiments were performed at least three times and each sample was conducted in triplicate.
CCK-8 assay
HUVECs were trypsinized and seeded into 96-well plates at a density of 1 × 103/well. On days 1–6, the medium was discarded, and the cells were washed with phosphate-buffered saline twice, followed by addition of DMEM medium and 10 μL CCK-8 reaction reagent. After incubation at 37 °C and 5% CO2 for 30 min, the absorbance of each well was measured at 450 nm to plot the cell proliferation curves. Each sample was tested in triplicate.
Transwell assay
HUVECs were collected by trypsin digestion, and resuspended to a density of 5 × 105 cells mL−1 using DMEM. HUVECs suspension (100 μL) was added into the migration chamber. In the lower chamber, 500 μL HEPG2 suspension or DMEM medium containing VEGF-A was added. After 24 h, the cells in the migration chamber were wiped by a cotton swab. Cells that moved to the other side of the chamber were fixed with 4% polyoxymethylene for 10 min. After being stained with 0.1% crystal violet for 15 min, the numbers of cells in five fields were counted under a microscope (200×) and were averaged.
Statistical analysis
Data are expressed as means values with standard error of the means (± SEM). Statistical significance was tested with one-way analysis of variance (ANOVA) followed by Tukey’s post hoc test or a paired Student’s t test. Statistical significance was set at P < 0.05.
Results and discussion
Decreased expression of miR-125a-5p in HHC HepG2 cells is potentially related to the increased expression of VEGF-A
Evidence has shown that miRNA molecules play a role in inhibiting or promoting tumour development by regulating the expression of their target genes [Citation18]. Studies show that high-density tumour microvessels are unfavourable prognostic factors in cancer patients [Citation19,Citation20]. Abundant microvessels are the basis for tumour growth, and the amount of angiogenic factors secreted by tumour cells through the paracrine pathway is important in determining tumour microangiogenesis [Citation12]. VEGF is a kind of specific endothelial growth factor, which plays an important role in the development of tumour angiogenesis. VEGF family has seven members, including VEGF-A, VEGF-B, VEGF-C, VEGF-D, VEGF-E, VEGF-F and placental growth factor [Citation21]. Activation of the VEGF-A/VEGFR-2 pathway can promote endothelial cell proliferation, migration and tubule formation. The VEGF-A/VEGFR-2 pathway also promotes the growth of primary or metastatic tumours. It is reported that VEGF-A plays a key role in tumour angiogenesis, and a large number of microvessels provide favourable conditions for tumour growth [Citation22]. miR-125a-5p is an independent prognostic factor for HCC, and may be related to its target gene HER-2 [23]. miR-125a-5p is abnormally expressed in HCC cells and specifically regulates the expression of E3F3, thereby inhibiting the proliferation, migration and invasion of tumour cells [Citation23]. It is also reported that miR-125a can inhibit the proliferation and metastasis of hepatoma carcinoma cells by down-regulating the expression of MMP11 and VEGF-A [Citation24]. However, the role of miR-125a-5p in HCC is still unclear. To examine the expression of miR-125a-5p and VEGF-A mRNA in cells, we performed RT-qPCR. The data showed that the levels of miR-125a-5p in SMMC7721, HepG2 and Huh-7 cells were significantly lower than that in THLE-2 cells (P < 0.05), with that in HepG2 cells being the lowest (). In addition, the levels of VEGF-A mRNA in SMMC7721, HepG2 and Huh-7 cells were significantly higher than that in THLE-2 cells (P < 0.05), with that in HepG2 cells being the highest (). The result suggests that decreased expression of miR-125a-5p in HHC HepG2 cells is potentially related to the increased expression of VEGF-A.
Expression of miR-125a-5p regulates VEGF-A expression and secretion of HepG2 cells
The paracrine secretion of VEGF-A by HCC cells could enhance angiogenesis by acting on the vascular endothelial cells around the tumour and promoting their proliferation, migration and tubule formation. We can infer that miR-125a-5p and the VEGF-A signalling pathway is a new way to regulate angiogenesis in HCC. HCC cells are metabolically active, and high-density microvessels can provide abundant nutrients, transport metabolites, and provide a pathway for tumour invasion and metastasis, thus promoting tumour development. However, whether miR-125a-5p and VEGF-A can be used as prognostic indicators in patients with liver cancer still needs further clinical studies. To investigate whether miR-125a-5p regulates the expression of VEGF-A in HepG2 cells, we transfected HepG2 cells with miR-125a-5p mimics or inhibitor. The data showed that the miR-125a-5p level in HepG2 cells transfected with miR-125a-5p inhibitor was significantly lower than that in the control group (P < 0.05), whereas that in HepG2 cells transfected with miR-125a-5p mimics was significantly higher than that in the control group (P < 0.05) (). In addition, the VEGF-A mRNA level in HepG2 cells transfected with miR-125a-5p inhibitor was significantly higher than that in the control group (P < 0.05), whereas that in HepG2 cells transfected with miR-125a-5p mimics was significantly lower than that in the control group (P < 0.05) (). Western blotting showed that VEGF-A protein expression in HepG2 cells transfected with miR-125a-5p inhibitor was significantly higher than that in the control group (P < 0.05), whereas that in HepG2 cells transfected with miR-125a-5p mimics was significantly lower than that in the control group (P < 0.05) (). The ELISA data showed that the VEGF-A content in the supernatant of HepG2 cells transfected with miR-125a-5p inhibitor was significantly higher than that in the control group (P < 0.05), whereas that in the supernatant of HepG2 cells transfected with miR-125a-5p mimics was significantly lower than that in the control group (P < 0.05) (). These results indicate that the expression of miR-125a-5p regulates VEGF-A expression and secretion of HepG2 cells.
Figure 2. Regulation of the expression of VEGF-A by miR-125a-5p. Relative expression of miR-125a-5p (A), VEGF-A mRNA (B), VEGF-A protein (C) in HepG2 cells, and VEGF-A content (D) in the culture supernatant of HepG2 cells. After transfection with miR-125a-5p inhibitors or miR-125a-5p mimics, the expression of miR-125a-5p or VEGF-A mRNA in HepG2 was analysed by RT-qPCR, and the expression of VEGF-A protein in HepG2 was determined by Western blotting. The content of VEGF-A in the supernatant of HepG2 cells was detected by ELISA. *, P < 0.05 compared with the control group, which was transfected with vehicle.
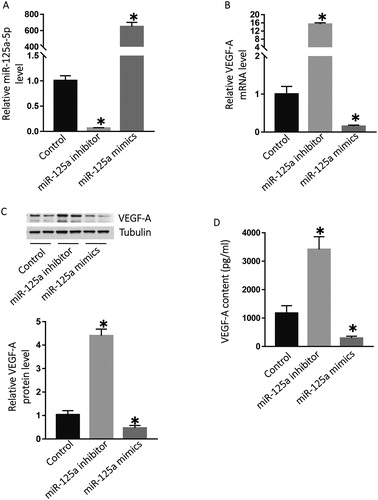
miR-125a-5p can bind with the 3’-UTR seed region of VEGF-A mRNA to inhibit its expression
To identify the interaction between miR-125a-5p and the 3’-UTR of VEGF-A mRNA, dual luciferase reporter assay was performed. The luciferase activity of cells co-transfected with miR-125a-5p inhibitor and wild-type (WT) luciferase reporter plasmids was significantly higher than that in the negative control group (P < 0.05), but that of cells co-transfected with miR-125a-5p mimics and WT luciferase reporter plasmids was significantly lower than that in the negative control group (P < 0.05) (). After mutating the CAGGGU sequence to a CUCCCU sequence at the 3'-UTR of VEGF-A, the luciferase activity of the cells co-transfected with miR-125a-5p inhibitor/mimics and mutant luciferase reporter plasmids was not different from that in the negative control group (P > 0.05) (). The result suggests that miR-125a-5p can bind with the 3’-UTR seed region of VEGF-A mRNA to inhibit its expression.
miR-125a-5p increases the expression and secretion of VEGF-A by HepG2 cells, which subsequently promote the proliferation of HUVECs by increasing the phosphorylation of Akt
The development, invasion and metastasis of malignant tumours are closely related to angiogenesis. Inhibiting angiogenesis of tumours has been considered by many researchers as an important starting point for inhibiting the growth process of tumours and carrying out anti-tumour therapy. Endothelial cell proliferation is an important process in angiogenesis [Citation25]. There are many cytokines promoting angiogenesis, including vascular endothelial growth factor, fibroblast growth factor and platelet-derived endothelial growth factor [Citation26]. Cytokines in circulation or tumour microenvironment activate downstream signalling pathways of receptors by binding to their corresponding receptors on endothelial cells, resulting in a series of cellular biological functions and promoting angiogenesis [Citation27]. To test the effect of VEGF-A secreted by HepG2 cells on the proliferation of HUVECs, we co-cultured HUVECs with the supernatant of HepG2 cells. CCK-8 assay showed that the absorbance of HUVECs co-cultured with HepG2 cells in the miR-125a-5p mimics group was significantly lower than that in the control group (P < 0.05), and that in the miR-125a-5p inhibitor group was significantly higher than that in the control group (P < 0.05) on days 2–7 (). Of note, treatment with Akt inhibitor, MK-2206, reduced the absorbance of the miR-125a-5p inhibitor group to levels similar to the control group (). Moreover, the absorbance of HUVECs treated with MK-2206 together with VEGF-A was significantly lower than that of HUVECs treated with VEGF-A alone (P < 0.05) (). Western blotting showed that addition of VEGF-A in the culture medium enhanced the expression of phosphorylated Akt, and addition of MK-2206 decreased the phosphorylation of Akt (). In addition, transfection with miR-125a-5p mimics reduced the secretion by VEGF-A in HepG2 cells, leading to reduced levels of phosphorylated Akt in HUVECs; transfection with miR-125a-5p inhibitor increased the secretion of VEGF-A by HepG2 cells, leading to elevated levels of phosphorylated Akt in HUVECs (). Of note, addition of Akt inhibitor, MK-2206, reversed the effect of the supernatant of HepG2 transfected with miR-125a-5p inhibitor on the phosphorylation of Akt in HUVECs (). These results indicate that miR-125a-5p increases the expression and secretion of VEGF-A by HepG2 cells, which subsequently promote the proliferation of HUVECs by increasing the phosphorylation of Akt.
Figure 4. Proliferation of HUVECs after treatment with the supernatant of HepG2 cells or VEGF-A. (A) Absorbance of HUVECs that were co-cultured with the supernatant of HepG2 cells transfected with vehicle (control group), miR-125a-5p mimics, or miR-125a-5p inhibitor. Akt inhibitor, MK-2206, was also used to treat HUVECs in the miR-125a-5p inhibitor group. *, P < 0.05 compared with the control group. (B) Absorbance of HUVECs treated with VEGF-A or both VEGF-A and MK-2206. *, P < 0.05 compared with the VEGF-A group. CCK-8 assay was used to detect cell proliferation. (C) Expression of phosphorylated Akt in HUVECs. Western blotting was used to determine the expression of Akt protein. *, P < 0.05 compared with the control group; #, P < 0.05 compared with the miR-125a-5p inhibitor group; &, P < 0.05 compared with the VEGF-A group.
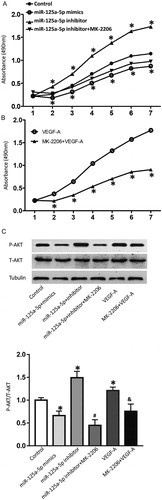
It is reported that microRNAs associated with the invasion and metastasis characteristics of hepatocellular carcinoma also include miR-34, miR-506 and miR-214 [28–30]. The loss of expression of miR-122 in hepatocellular carcinoma leads to the increase of cell migration and invasion. Recovering the expression of miR-122 in metastatic hepatocellular carcinoma cells can significantly inhibit cell migration, invasion, tumourigenesis, angiogenesis and intrahepatic metastasis [Citation31]. These invasive and metastatic abilities are due to the fact that miRNAs have been shown to inhibit or promote the development of tumours by regulating the expression of target genes. In the present study, we mainly studied the role of miR-125a-5p in angiogenesis of hepatocellular carcinoma. Whether other miRNAs have similar effects needs further study.
miR-125a-5p regulates the migration of HUVECs via the VEGF-A/VEGFR2/Akt signalling pathway, which is affected by the expression and secretion of VEGF-A by HepG2 cells
Although anti-angiogenesis therapy for malignant tumours has a certain therapeutic effect, there are also many controversies and shortcomings [Citation32,Citation33]. Theoretically, any molecule in the miR-125a-5p/VEGF-A/VEGFR-2/Akt signalling pathway may be a therapeutic target. Anti-angiogenesis drugs target VEGF or its receptors to reduce angiogenesis, and they are designed to reduce the blood supply to tumours. However, this may also affect the physiological function of normal tissues and organs, and make tumours therapeutically tolerant. Therefore, it is very likely to achieve therapeutic effect by targeting upstream molecules of the VEGF-A signalling pathway, such as miR-125a-5p. To examine how VEGF-A secreted by HepG2 cells affected the migration of HUVECs, Transwell assay was performed. The data showed that the number of migrated HUVECs co-cultured with HepG2 cells that were transfected with miR-125a-5p mimics was smaller than that in the control group (P < 0.05), whereas the number of migrated HUVECs co-cultured with HepG2 cells that were transfected with miR-125a-5p inhibitor was higher than that in the control group (P < 0.05). Treatment with Akt inhibitor, MK-2206, significantly reduced the number of migrated HUVECs co-cultured with HepG2 cells that were transfected with miR-125a-5p inhibitor (P < 0.05). In addition, treatment with VEGF-A alone significantly increased the number of migrated HUVECs compared with that in the control group (P < 0.05), whereas treatment with MK-2206 significantly reduced the number of migrated HUVECs compared with that in the VEGF-A group (P < 0.05) (). The results suggest that miR-125a-5p regulates the migration of HUVECs via the VEGF-A/VEGFR2/Akt signalling pathway, which is affected by the expression and secretion of VEGF-A by HepG2 cells.
Figure 5. Migration of HUVECs after treatment with the supernatant of HepG2 cells or VEGF-A. HUVECs were co-cultured with the supernatant of HepG2 cells transfected with vehicle (control group), miR-125a-5p mimics, or miR-125a-5p inhibitor. Akt inhibitor, MK-2206, was also used to treat HUVECs in the miR-125a-5p inhibitor group. HUVECs were also treated with VEGF-A or both VEGF-A and MK-2206. Transwell assay was used to detect the migration ability of HUVECs in different groups. *, P < 0.05 compared with the control group; #, P < 0.05 compared with the miR-125a-5p inhibitor group; &, P < 0.05 compared with the VEGF-A group.
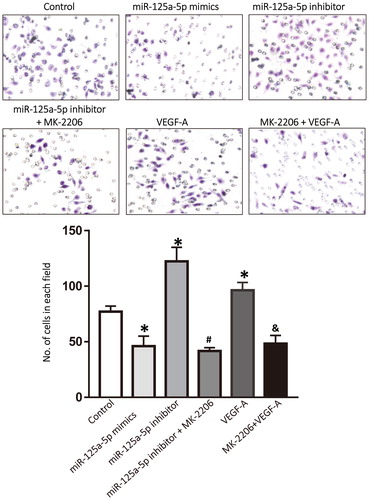
The targeted regulation by miRNA is not via a single gene, but a regulatory network. VEGF-A is only one of the target genes of miR-125a-5p. Therefore, this target may cause side effects and may not be effective in humans. Further studies on the mechanism of miR-125a-5p in vivo are still needed. In conclusion, the present study demonstrates that miR-125a-5p inhibits the growth of HCC cells by targeting VEGF-A expression, and increased expression of miR-125a-5p can inhibit angiogenesis in HCC. The study provides new insights into clinical treatments for HCC and other malignant tumours. It is reported that anti-angiogenesis therapy for malignant tumours has been intensively studied in the past 20 years, although it has many controversies and shortcomings [Citation34]. Theoretically, any molecule in the signal transduction pathway of miR-125a-5p/VEGF-A/VEGFR2/Akt may become a therapeutic target [Citation35]. Anti-angiogenic drugs target the growth factor or its receptors to reduce angiogenesis, with the aim of reducing blood supply to tumours, but it may also affect the physiological function of normal tissues and organs, and make tumours therapeutically tolerant [Citation36]. Therefore, targeting upstream molecules of vascular endothelial growth factor-A, such as miR-125a-5p, is likely to achieve therapeutic effect. Targeted regulation by miRNAs is not towards a single gene, but a regulatory network. VEGF-A is only one of the target genes of miR-125a-5p. Therefore, the use of this target in drug therapy may bring side effects and may not bring good therapeutic effects in the human body. Therefore, it is necessary to further study the mechanism of miR125a-5p in vivo in the future.
Conclusions
The present study demonstrates that miR-125a-5p inhibits the growth of hepatocellular carcinoma cells by targeting the expression of VEGF-A, and increasing the expression of miR-125a-5p inhibits angiogenesis of hepatocellular carcinoma. Our results provide new insights into the pathogenesis of hepatocellular carcinoma and bring new therapeutic ideas for the clinical diagnosis and treatment of hepatocellular carcinoma and other malignant tumours.
Ethical approval and consent to participate
All procedures performed in the current study were approved by the Ethics Committee of Liaocheng People’s Hospital. Written informed consent was obtained from all patients or their families.
Consent for publication
Written informed consents for publication of any associated data and accompanying images were obtained from all patients or their parents, guardians or next of kin.
Acknowledgements
The authors thank their department and research team for their help and dedication.
Availability of data and materials
The datasets used and/or analysed during the current study are available from the corresponding author on reasonable request.
Disclosure statement
No potential conflict of interest was reported by the authors.
Additional information
Notes on contributors
Jingjing Liu
The final version of the manuscript has been read and approved by all authors, and each author believes that the manuscript represents honest work. JL and CT collaborated to design the study. Both of JL and CT were responsible for performing experiments and analysing data. Both authors collaborated to interpret results and develop the manuscript.
Changming Tao
The final version of the manuscript has been read and approved by all authors, and each author believes that the manuscript represents honest work. JL and CT collaborated to design the study. Both of JL and CT were responsible for performing experiments and analysing data. Both authors collaborated to interpret results and develop the manuscript.
References
- Llovet JM, Burroughs A, Bruix J. Hepatocellular carcinoma. Lancet (London, England). 2003;362:1907–1917.
- Sherman M. Hepatocellular carcinoma: epidemiology, risk factors, and screening. Semin Liver Dis. 2005;25:143–154.
- Semela D, Dufour JF. Angiogenesis and hepatocellular carcinoma. J Hepatol. 2004; 41:864–880.
- Carmeliet P, Jain RK. Angiogenesis in cancer and other diseases. Nature. 2000; 407:249–257.
- O'Reilly MS, Boehm T, Shing Y, et al. Endostatin: an endogenous inhibitor of angiogenesis and tumor growth. Cell. 1997; 88:277–285.
- Zhang Y, Han J, Yang X, et al. Pigment epithelium-derived factor inhibits angiogenesis and growth of gastric carcinoma by down-regulation of VEGF. Oncol Rep. 2011;26:681–686.
- Folkman J. Angiostatin and endostatin: angiogenesis inhibitors in blood and stroma. US: Springer; 2008.
- Carmeliet P. VEGF as a key mediator of angiogenesis in cancer. Oncology. 2005;69:4–10.
- Carthew RW, Sontheimer EJ. Origins and mechanisms of miRNAs and siRNAs. Cell. 2009; 136:642–655.
- Liu Y, Li Z, Wu L, et al. MiRNA-125a-5p: a regulator and predictor of gefitinib's effect on nasopharyngeal carcinoma. Cancer Cell Int. 2014; 14:24.
- Huang B, Luo W, Sun L, et al. MiRNA-125a-3p is a negative regulator of the RhoA-actomyosin pathway in A549 cells. Int J Oncol. 2013; 42:1734–1742.
- Viallard C, Larrivee B. Tumor angiogenesis and vascular normalization: alternative therapeutic targets. Angiogenesis. 2017; 20:409–426.
- Kim JK, Noh JH, Jung KH, et al. Sirtuin7 oncogenic potential in human hepatocellular carcinoma and its regulation by the tumor suppressors MiR-125a-5p and MiR-125b. Hepatology (Baltimore, MD). 2013; 57:1055–1067.
- Hsieh TH, Hsu CY, Tsai CF, et al. miR-125a-5p is a prognostic biomarker that targets HDAC4 to suppress breast tumorigenesis. Oncotarget. 2015; 6:494–509.
- Xu Y, Huang Z, Liu Y. Reduced miR-125a-5p expression is associated with gastric carcinogenesis through the targeting of E2F3. Mol Med Rep. 2014; 10:2601–2608.
- Tang H, Li RP, Liang P, et al. miR-125a inhibits the migration and invasion of liver cancer cells via suppression of the PI3K/AKT/mTOR signaling pathway. Oncol Lett. 2015;10:681–686.
- Melton DW, Lei X, Gelfond JA, et al. Dynamic macrophage polarization-specific miRNA patterns reveal increased soluble VEGF receptor 1 by miR-125a-5p inhibition. Physiol Genom. 2016; 48:345–360.
- Pogue AI, Cui JG, Li YY, et al. Micro RNA-125b (miRNA-125b) function in astrogliosis and glial cell proliferation. Neurosci Lett. 2010;476:18–22.
- Kerbel RS. Tumor angiogenesis. N Engl J Med. 2008; 358:2039–2049.
- Ronca R, Benkheil M, Mitola S, et al. Tumor angiogenesis revisited: regulators and clinical implications. Med Res Rev. 2017; 37:1231–1274.
- Leppanen P, Koota S, Kholova I, et al. Gene transfers of vascular endothelial growth factor-A, vascular endothelial growth factor-B, vascular endothelial growth factor-C, and vascular endothelial growth factor-D have no effects on atherosclerosis in hypercholesterolemic low-density lipoprotein-receptor/apolipoprotein B48-deficient mice. Circulation. 2005; 112:1347–1352.
- Dellinger MT, Brekken RA. Phosphorylation of Akt and ERK1/2 is required for VEGF-A/VEGFR2-induced proliferation and migration of lymphatic endothelium. PLoS One. 2011;6:e28947.
- Nishida N, Mimori K, Fabbri M, et al. MicroRNA-125a-5p is an independent prognostic factor in gastric cancer and inhibits the proliferation of human gastric cancer cells in combination with trastuzumab. Clin Cancer Res Off J Am Assoc Cancer Res. 2011; 17:2725–2733.
- Potenza N, Mosca N, Zappavigna S, et al. MicroRNA-125a-5p is a downstream effector of sorafenib in its antiproliferative activity toward human hepatocellular carcinoma cells. J Cell Physiol. 2017;232:1907–1913.
- Maity G, Chakraborty J, Ghosh A, et al. Aspirin suppresses tumor cell-induced angiogenesis and their incongruity. J Cell Commun Signal. 2019;Jan 4.
- Silva TCPD, Silva MG, Shinjo SK. Relevance of serum angiogenic cytokines in adult patients with dermatomyositis. Adv Rheumatol.. 2018;58:17.
- Sun W, Wang X, Li J, et al. MicroRNA-181a promotes angiogenesis in colorectal cancer by targeting SRCIN1 to promote the SRC/VEGF signaling pathway. Cell Death Dis. 2018;9:438.
- Zhang L, Liao Y, Tang L. MicroRNA-34 family: a potential tumor suppressor and therapeutic candidate in cancer. J Exp Clin Cancer Res.. 2019;38:
- Liang TS, Zheng YJ, Wang J, et al. MicroRNA-506 inhibits tumor growth and metastasis in nasopharyngeal carcinoma through the inactivation of the Wnt/β-catenin signaling pathway by down-regulating LHX2. J Exp Clin Cancer Res.. 2019;38:97.
- Wang R, Sun Y, Yu W, et al. Downregulation of miRNA-214 in cancer-associated fibroblasts contributes to migration and invasion of gastric cancer cells through targeting FGF9 and inducing EMT. J Exp Clin Cancer Res. 2019;38:20.
- Satishchandran A, Ambade A, Rao S, et al. MicroRNA 122, regulated by GRLH2, protects livers of mice and patients from ethanol-induced liver disease. Gastroenterology. 2018;154:238–252.
- Murakami Y, Yasuda T, Saigo K, et al. Comprehensive analysis of microRNA expression patterns in hepatocellular carcinoma and non-tumorous tissues. Oncogene. 2006; 25:2537–2545.
- Jiang J, Gusev Y, Aderca I, et al. Association of MicroRNA expression in hepatocellular carcinomas with hepatitis infection, cirrhosis, and patient survival. Clin Cancer Res Off J Am Assoc Cancer Res. 2008;14:419–427.
- Nienhüser H, Schmidt T. Angiogenesis and anti-angiogenic therapy in gastric cancer. Int J Mol Sci. 2018;19:43.
- Shi H, Bi H, Sun X, et al. Antitumor effects of Tubeimoside-1 in NCI-H1299 cells are mediated by microRNA-126-5p-induced inactivation of VEGF-A/VEGFR-2/ERK signaling pathway. Mol Med Rep.. 2018;17:4327–4336.
- Tomida C, Yamagishi N, Nagano H, et al. VEGF pathway-targeting drugs induce evasive adaptation by activation of neuropilin-1/cMet in colon cancer cells. Int J Oncol.. 2018;52:1350.