Abstract
This study determined the expression of microRNA-497 (miR-497) and interleukin-6 receptor (IL-6R) in tumour, blood and urine from patients with clear cell renal cell carcinoma (ccRCC), and examined their relationship with the proliferation of ccRCC. Twenty-eight ccRCC patients and 32 healthy subjects gave peripheral blood and urine samples. Tumour and tumour-adjacent tissues were collected from ccRCC patients. Quantitative real-time polymerase chain reaction (qRT-PCR) was used to measure the expression of IL-6R mRNA and miR-497; Western blotting, to determine IL-6R protein expression in tumour tissues or ccRCC Caki-1 cells; enzyme-linked immunosorbent assay, to measure the content of IL-6R protein in blood or urine; dual luciferase reporter assay, to test the direct interaction between miR-497 and the 3′-untranslated region (UTR) of IL-6R mRNA; MTT (methyl thiazolyl tetrazolium) assay, to determine the proliferation of cells. The expression of IL-6R mRNA and protein was significantly higher in tumour tissues than in tumour-adjacent tissues. Similarly, the expression of IL-6R mRNA and protein in blood and urine was significantly higher in ccRCC patients than in healthy subjects. The levels of miR-497 in tumour tissues, blood and urine from ccRCC patients were decreased. miR-497 bound with the 3′-UTR of IL-6R mRNA to regulate the expression of IL-6R. Overexpression of miR-497 inhibited the proliferation of ccRCC Caki-1 cells by down-regulating the expression of IL-6R. These results demonstrate that up-regulation of IL-6R expression in tumour tissues, blood and urine from ccRCC patients is related with the down-regulation of miR-497 expression. miR-497 might regulate the proliferation of ccRCC via IL-6R.
Introduction
Renal cell carcinoma (RCC), also known as renal adenocarcinoma, is a malignant tumour mainly derived from renal tubular epithelial cells [Citation1]. RCC is the most common type of renal cancer in adults, accounting for about 80–90% [Citation1]. Among RCC, clear cell renal cell carcinoma (ccRCC) is the most common, accounting for about 75% of all RCC cases [Citation2].
Although scientists have done a lot of research on the pathogenesis and metastasis mechanism of RCC, the specific mechanism is not fully clear yet. However, progress has also been made in understanding molecular biology and genomics, which has promoted the development of new therapeutic drugs [Citation3]. Interleukin (IL)-6 is a key inflammatory factor in the process of inflammation [Citation4]. It has been reported that IL-6 plays an important role in the pathogenesis of RCC [Citation5]. Only when IL-6 binds with IL-6 receptor (IL-6R) to form IL-6/IL-6R complex can IL-6 bind with gp130 to form high-affinity complex and play a biological role [Citation6]. However, the regulatory role of IL-6R in ccRCC remains unclear.
MicroRNA (miRNA or miR) is a class of non-coding small RNA with 18–22 nucleotides, which widely exists in eukaryotes and regulate the expression of proteins at mRNA level [Citation7,Citation8]. Changes in the expression of miRNAs and proteins are associated with the pathogenesis of ccRCC, suggesting that miRNAs may play important roles in the regulation of disease-related proteins [Citation9,Citation10]. Studies show that miR-497 participate in many processes, such as inflammatory response, malignant behaviours of tumours and epithelial-mesenchymal transformation [Citation11–13]. However, the relationship between miR-497 and IL-6R in ccRCC has not been reported before.
In the present study, we determined the expression of IL-6R in tumour, blood and urine from patients with ccRCC, and tried to predict and validate its relationship with miR-497.
Subjects and methods
Subjects
A total of 28 patients with ccRCC who underwent resection surgery at our hospital between December 2014 and June 2018 were included in the experimental group (16 males and 12 females; age range, 43–65 years old; median age, 55.6 years old). For all these patients it was the first time to suffer from the disease. They did not have a history of hormone treatment, intake of traditional Chinese medicine, radiotherapy or chemotherapy before operation. In addition, 32 healthy subjects who underwent physical examination during the same period at our hospital were included into the control group (17 males and 15 females; age range, 42–69 years old; median age, 56.1 years old). Peripheral blood and urine samples were collected from both experimental and control groups. Tumour tissues were collected from ccRCC patients, and tumour-adjacent tissues were also collected as control.
Ethics statement
All procedures performed in the present study were approved by the Ethics Committee of Jinzhou Medical University. Written informed consent was obtained from all patients or their families.
Quantitative real-time polymerase chain reaction (qRT-PCR)
Tissue samples (100 mg) were ground into powder in liquid nitrogen and lysed with 1 mL TRIzol reagent following the manufacturer’s manual (Thermo Fisher Scientific, Waltham, MA). Blood or urine (100 μL) was directly lysed with 1 mL TRIzol reagent. Total RNA was extracted using the phenol–chloroform method. The concentration and quality of RNA was measured using ultraviolet spectrophotometry (Nanodrop ND2000, Thermo Scientific, Waltham, MA). Then, cDNA was obtained by reverse transcription from 1 μg RNA and stored at −20 °C. Reverse transcription of mRNA was performed using TIANScript II cDNA First Strand Synthesis Kit (Tiangen, Beijing, China), and reverse transcription of miRNA was carried out using miRcute miRNA cDNA First Strand Synthesis Kit (Tiangen, Beijing, China).
SuperReal PreMix (SYBR Green) qRT-PCR kit (Tiangen, Beijing, China) was used to detect mRNA expression of IL-6R, using GAPDH (glyceraldehyde 3-phosphate dehydrogenase) as internal reference. The primers for amplification of IL-6R were 5′- TGGTGGATGTTCCCCCCGAG -3′ (forward) and 5′- TCCTGGGAATACTGGCACGG -3′ (reverse). The s primers for amplification of GAPDH were 5′- AAGGCTGTGGGCAAGG -3′ (forward) and 5′- TGGAGGAGTGGGTGTCG -3′ (reverse). The reaction system (20 μL) contained 10 μL qRT-PCR-Mix, 0.5 μL upstream primer, 0.5 μL downstream primer, 2 μL cDNA and 7 μL ddH2O. The reaction protocol was: initial denaturation at 95 °C for 30 s; denaturation at 95 °C for 5 s and annealing at 60 °C for 20 s (39 cycles) (iQ5; Bio-Rad, Hercules, CA). The 2-ΔΔCt method [Citation14] was used to calculate the relative expression of IL-6R against GAPDH. Each sample was tested in triplicate.
The expression of miR-497 was determined by miRcute miRNA RT-PCR Kit (Tiangen, Beijing, China), using U6 as internal reference. The sequences of the miR-497 primers were 5′- TCGGGCAGCAGCACACTGTG -3′ (forward) and 5′- GTGCAGGGTCCGAGGT -3′ (reverse); the sequences of the U6 primers were 5′- CGCTTCGGCAGCACATATAC -3′ (forward) and 5′- TTCACGAATTTGCGTGTCAT -3′ (reverse). The reaction system (20 μL) contained 10 μL qRT-PCR-Mix, 0.5 μL upstream primer, 0.5 μL downstream primer, 2 μL cDNA and 7 μL ddH2O. The reaction protocol was: initial denaturation at 95 °C for 5 min; denaturation at 95 °C for 10 s, annealing at 60 °C for 20 s (40 cycles); elongation at 72 °C for 10 s (iQ5; Bio-Rad, Hercules, CA). The 2-ΔΔCt method [Citation15] was used to calculate the relative expression of miR-497 against U6. Each sample was tested in triplicate.
Western blotting
Before lysis, tissues (100 mg) were ground into powder. Then, tissue samples were lysed with precooled Radio-Immunoprecipitation Assay (RIPA) lysis buffer: 600 μL; 50 mmol L−1 Tris-base, 1 mmol L−1 EDTA, 150 mmol L−1 NaCl, 0.1% sodium dodecyl sulfate, 1% TritonX-100, 1% sodium deoxycholate (Beyotime Institute of Biotechnology, Shanghai, China), for 30 min on ice. The mixture was centrifuged at 12,000 rpm and 4 °C for 10 min. The supernatant was used to determine protein concentration by bicinchoninic acid (BCA) protein concentration determination kit (RTP7102, Real-Times Biotechnology, Beijing, China). The samples were then mixed with 5× sodium dodecyl sulfate loading buffer before denaturation in boiling water bath for 5 min. Afterwards, the samples (20 µg) were subjected to 10% sodium dodecyl sulfate-polyacrylamide gel electrophoresis at 100 V. The resolved proteins were transferred to polyvinylidene difluoride membranes on ice (100 V, 2 h) and blocked with 5% skimmed milk at room temperature for 1 h. Then, the membranes were incubated with rabbit anti-human IL-6R (1:1000; ab128008; Abcam, Cambridge, UK) or β-actin (1:5000; ab8227; Abcam, Cambridge, UK) polyclonal primary antibodies at 4 °C overnight. After extensive washing with phosphate-buffered saline with Tween 20 (three times for 15 min), the membranes were incubated with goat anti-rabbit horseradish peroxidase-conjugated secondary antibody (1:3,000; ab6721; Abcam, Cambridge, UK) for 1 h at room temperature before washing with phosphate-buffered saline with Tween 20 (three times for 15 min). Then, the membrane was developed with enhanced chemiluminescence detection kit (ab65623; Abcam, Cambridge, UK) for imaging. Image lab v3.0 software (Bio-Rad, Hercules, CA) was used to acquire and analyse the imaging signals. The relative content of target protein was expressed against β-actin.
Enzyme-linked immunosorbent assay (ELISA)
Blood samples were centrifuged at 1200 g and 4 °C for 10 min before serum was separated for ELISA. Urine was centrifuged at 1200 g and 4 °C for 10 min before supernatant was aspirated for ELISA. Human IL-6R ELISA kit (ab46029; Abcam, Cambridge, UK) was used to determine the concentration of IL-6R. In microplates, standards (50 μL) and samples (10 μL serum and 40 μL diluent) were added into predefined wells, while blank wells were left empty. In the wells for standards and samples, horseradish peroxidase-labelled conjugates (100 μL) were added before sealing the plates for incubation at 37 °C for 1 h. After washing the plates 5 times, substrates A (50 μL) and B (50 μL) were added into each well. After incubation at 37 °C for 15 min, stop solution (50 μL) was added into each well, and the absorbance of each well was measured at 450 nm within 15 min.
Bioinformatics
We used miRanda (http://www.microrna.org/microrna/home.do) to predict target genes that might regulate IL-6R.
Cells
One day before transfection, ccRCC Caki-1 cells (3 × 105; ATCC, Manassas, VA) in logarithmic growth were seeded onto 24-well plates, and cultured in antibiotics-free F12/DMEM medium supplemented with 10% fetal bovine serum until reaching 70% confluency. In the first vial, 1.5 µL plasmids, small-interfering RNA (siRNA), agomiRNAs (20 pmol µL−1) (Sangon Biotech, Shanghai, China) was mixed with 50 µL Opti Mem medium (Thermo Fisher Scientific, Waltham, MA). In the second vial, 1 µL Lipofectamine 2000 (Thermo Fisher Scientific, Waltham, MA) was mixed with 50 µL Opti Mem medium. After standing still for 5 min, the two vials were combined for additional waiting at room temperature for 20 min. Then, the mixtures were added onto cells in respective groups. Six hours later, the medium was replaced with F12/DMEM medium containing 10% fetal bovine serum. After cultivation for 48 h, the cells were collected for further assays.
Dual luciferase reporter assay
According to the bioinformatics results, wild-type (WT) and mutant seed regions of miR-497 in the 3′-untranslated region (UTR) of the IL-6R gene were chemically synthesised in vitro. Then, their two ends were attached with Spe-1 and HindIII restriction sites, and then cloned into pMIR-REPORT luciferase reporter plasmids. Plasmids (0.8 μg) with WT or mutant 3′-UTR sequences were co-transfected with agomiR-497 [100 nmol/L; 5′- CAGCAGCACACUGUGGUUUGU -3′ (forward) and 3′- GUCGUCGUGUGACACCAAACA -5′ (reverse); Sangon Biotech, Shanghai, China] into 293 T cells. For control, 293 T cells were transfected with agomiR-negative control [NC; 100 nmol L−1; 5′- UUCUCCGAACGUGUCACGUTT -3′ (forward) and 3′- TTAAGAGGCUUGCACAGUGCA -5′ (reverse); Sangon Biotech, Shanghai, China]. After cultivation for 24 h, the cells were lysed using dual luciferase reporter assay kit (Promega, Fitchburg, WI) according to the manufacturer’s manual, and luminescence intensity was measured using GloMax 20/20 luminometer (Promega, Fitchburg, WI). Using renilla luminescence activity as internal reference, the luminescence values of each group of cells were measured.
MTT assay
After transfection, cells were seeded into 96-well plates at a density of 2 × 103 cells per well. Each condition was tested in triplicate wells. At 24, 48 and 72 h after transfection, 20 µL MTT (5 g L−1; JRDC000003, JRDUN Biotechnology, Shanghai, China) was added to each well, followed by incubation for 4 h at 37 C. After aspiration of medium, DMSO (150 µL per well) was added to dissolve purple crystals. Then, the absorbance of each well was measured at 490 nm with a microplate reader (Bio-Rad, Hercules, CA) and cell proliferation curves were plotted.
Statistical analysis
The results were analysed using SPSS 18.0 statistical software (IBM, Armonk, NY). The data were expressed as means values with standard deviations (±SD). Data were tested for normality. Multigroup measurement data were analysed using one-way analysis of variance (ANOVA). In case of homogeneity of variance, Least Significant Difference and Student–Newman–Keuls methods were used; in case of heterogeneity of variance, Tamhane’s T2 or Dunnett’s T3 method was used. Comparison between two groups was carried out using Student’s t test. P < 0.05 indicated statistically significant differences.
Results and discussion
Expression of IL-6R mRNA is elevated in tumour tissues, blood and urine from ccRCC patients
RCC is associated with many factors, including smoking, obesity, hypertension, occupational exposure, and so forth [Citation15,Citation16]. Surgery is the main treatment for ccRCC. Over 97% of patients with localised ccRCC who have undergone partial nephrectomy or radical nephrectomy have a tumour-specific survival time of more than 9 years [Citation17]. However, once ccRCC migrates, the prognosis is poor. The median survival time of untreated metastatic ccRCC patients is only 6–10 months [Citation18,Citation19]. It is important to find molecular biological indicators for early warning and screening of ccRCC. IL-6, which is elevated in immune responses, is a multifunctional cytokine produced by the body after stimulation by foreign bodies such as bacteria, endotoxin and dust particles. Increased levels of IL-6 in the body can cause inflammatory diseases such as rheumatoid arthritis by binding to IL-6R [Citation20]. IL-6 has chemotactic effect on other inflammatory cells in inflammatory responses [Citation21]. IL-6 can also induce the body to produce C-reactive protein and fibrinogen in inflammation, and can promote thrombosis [Citation22]. These suggest that IL-6 plays important roles in inflammatory responses. To play a role in cells, IL-6 must first bind to its membrane receptor IL-6R, which is only expressed in hepatocytes, monocytes/macrophages and some lymphocytes. Subsequently, this receptor-ligand complex binds to gp130, causing gp130 dimerisation and activating intracellular signals [Citation23]. Therefore, IL-6R is one of the most important components for IL-6 to exert its biological functions. Blocking IL-6R may delay the occurrence and development of ccRCC [Citation24,Citation25]. To determine the expression of IL-6R mRNA, qRT-PCR was carried out. The data showed that the level of IL-6R mRNA in tumour tissues was significantly higher than that in tumour-adjacent tissues (P < 0.01) (), and those in blood and urine from ccRCC patients were also significantly higher than those from the control group (P < 0.05) (). The results suggest that the expression of IL-6R mRNA is elevated in tumour tissues, blood and urine from ccRCC patients.
IL-6R protein expression is up-regulated in ccRCC tissues, being consistent with the expression of IL-6R mRNA
To measure the expression of IL-6R protein in tumour tissues, Western blotting was performed. The data showed that IL-6R protein expression in ccRCC tissues was significantly higher than that in tumour-adjacent tissues (P < 0.01) (). The result indicates that IL-6R protein expression is up-regulated in ccRCC tissues, being consistent with the expression of IL-6R mRNA.
Levels of IL-6R protein secreted into the blood and urine of ccRCC patients are higher than those of healthy subjects
To test the contents of IL-6R protein in blood and urine, ELISA was used. The data showed that the contents of IL-6R protein in blood and urine from ccRCC patients were significantly higher than those from the control group (P < 0.05) (). The results suggest that the levels of IL-6R protein secreted into the blood and urine of ccRCC patients are higher than those of healthy subjects.
Expression of miR-497 is reduced in tumour tissues, blood and urine from ccRCC patients
Then, we further studied the upstream regulatory genes of IL-6R. Using bioinformatics, we discovered that miR-497 was a potential upstream gene that might regulate IL-6R, and UGCUGCU is a potential seed region of miR-497 (). It is reported that mR-497 can inhibit colon cancer by targeting IGF-1 receptor [Citation26]. It also inhibits angiogenesis in ovarian cancer by regulating PI3K/AKT and MAPK/ERK pathways, using vascular endothelial growth factor gene as a target [Citation27]. In addition, miR-497 targets CCNE1 and inhibits the proliferation of cervical cancer cells [Citation28]. By inhibiting eIF4E, miR-497 inhibits the proliferation of gastric cancer cells [Citation29]. Moreover, it negatively regulates CHEK1 and inhibits hepatocellular carcinoma cells [Citation30]. On the basis of these reports, we hypothesised that miR-497 also plays a similar role in tumour regulation in ccRCC. To evaluate the expression of miR-497, qRT-PCR was employed. The data showed that the level of miR-497 in tumour tissues from ccRCC patients was significantly lower than that in tumour-adjacent tissues (P < 0.01) (). In addition, the levels of miR-497 in blood and urine from patients with ccRCC were significantly lower than those from healthy subjects (P < 0.01) (). The results indicate that the expression of miR-497 is reduced in tumour tissues, blood and urine from ccRCC patients.
miR-497 binds with the 3′-UTR of IL-6R mRNA to regulate the expression of IL-6R
To identify the direct interaction between miR-497 and IL-6R mRNA, dual luciferase reporter assay was performed. The data showed that the luminescence intensity of the wild-type group was significantly lower than that in negative control group (P < 0.01), and that of the mutant group was not different from that in the negative control group (P > 0.05) (). The result suggests that miR-497 binds with the 3′-UTR of IL-6R mRNA to regulate the expression of IL-6R.
Overexpression of miR-497 inhibits the proliferation of ccRCC Caki-1cells by down-regulating the expression of IL-6R
To examine how miR-497 expression affects the proliferation of ccRCC Caki-1 cells, the cells were transfected with agomiR-497. The data showed that the level of miR-497 in Caki-1 cells transfected with agomiR-497 was significantly higher than that in cells transfected with agomiR-NC (P < 0.01) (). The expression of IL-6R mRNA in Caki-1 cells transfected with agomiR-497 was significantly lower than that in cells transfected with agomiR-NC (P < 0.05) (). Similarly, expression of IL-6R protein in Caki-1 cells transfected with agomiR-497 was significantly lower than that in cells transfected with agomiR-NC (P < 0.05) (). Moreover, the absorbance of Caki-1 cells transfected with agomiR-497 was significantly slower than that of cells transfected with agomiR-NC at 48 h and 72 h (P < 0.05 for both) (). The results indicate that overexpression of miR-497 inhibits the proliferation of ccRCC Caki-1 cells by down-regulating the expression of IL-6R.
Figure 7. Effect of miR-497 overexpression on the proliferation of ccRCC Caki-1 cells. (A) Relative expression of miR-497 in Caki-1 cells transfected with agomiR-negative control (NC) or agomiR-497. **P < 0.01 compared with agomiR-NC group. (B) Relative expression of IL-6R mRNA in Caki-1 cells transfected with agomiR-NC or agomiR-497. *P < 0.05 compared with agomiR-NC group. (C) Relative expression of IL-6R protein in Caki-1 cells transfected with agomiR-NC or agomiR-497. *P < 0.05 compared with agomiR-NC group. (D) Absorbance of Caki-1 cells transfected with agomiR-NC or agomiR-497 at 24 h, 48 h or 72 h after transfection. MTT assay was used to examine proliferation. *P < 0.05 compared with agomiR-NC group.
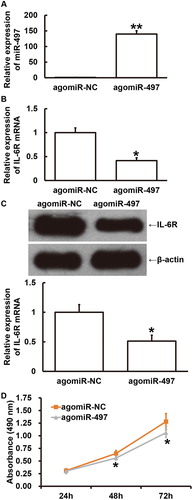
Conclusions
The present study demonstrates that reduced expression of miR-497 in ccRCC patients regulates the expression of IL-6R as well as inflammatory responses mediated by IL-6/IL-6R. Therefore, miR-497 and IL-6R play important biological roles in the occurrence and development of ccRCC.
Ethical approval and consent to participate
All procedures performed in the present study were approved by the Ethics Committee of Jinzhou Medical University. Written informed consent was obtained from all patients or their families.
Consent for publication
Written informed consents for publication of any associated data and accompanying images were obtained from all patients or their parents, guardians or next of kin.
Acknowledgements
The authors thank their department and research team for their help and dedication.
Availability of data and materials
The datasets used and/or analysed during the current study are available from the corresponding author on reasonable request.
Disclosure statement
No potential conflict of interest is reported by the authors.
Additional information
Funding
Notes on contributors
Caixia Gao
The final version of the manuscript has been read and approved by all authors, and each author believes that the manuscript represents honest work. CG and LL collaborated to design the study. CG and YL were responsible for performing experiments. CG and LL analysed the data. All authors collaborated to interpret results and develop the manuscript.
Yanxia Li
The final version of the manuscript has been read and approved by all authors, and each author believes that the manuscript represents honest work. CG and LL collaborated to design the study. CG and YL were responsible for performing experiments. CG and LL analysed the data. All authors collaborated to interpret results and develop the manuscript.
Lin Liu
The final version of the manuscript has been read and approved by all authors, and each author believes that the manuscript represents honest work. CG and LL collaborated to design the study. CG and YL were responsible for performing experiments. CG and LL analysed the data. All authors collaborated to interpret results and develop the manuscript.
References
- Ferlay J, Soerjomataram I, Dikshit R, et al. Cancer incidence and mortality worldwide: sources, methods and major patterns in GLOBOCAN 2012. Int J Cancer. 2015;136:E359–E386.
- Park BK. Renal angiomyolipoma based on new classification: how to differentiate it from renal cell carcinoma. Am J Roentgenol. 2019;212:582–588.
- Jonasch E, Gao J, Rathmell WK. Renal cell carcinoma. BMJ (Clin Res Ed). 2014;349:g4797.
- Chen Y, Liu J, Lv P, et al. IL-6 is involved in malignancy and doxorubicin sensitivity of renal carcinoma cells. Cell Adh Migr. 2018; 12:28–36.
- Kaminska K, Czarnecka AM, Escudier B, et al. Interleukin-6 as an emerging regulator of renal cell cancer. Urol Oncol. 2015; 33:476–485.
- Wolf J, Waetzig GH, Chalaris A, et al. Different soluble forms of the interleukin-6 family signal transducer gp130 fine-tune the blockade of interleukin-6 trans-signaling. J Biol Chem. 2016;291:16186–16196.
- Graziano A, Lo Monte G, Piva I, et al. Diagnostic findings in adenomyosis: a pictorial review on the major concerns. Eur Rev Med Pharmacol Sci. 2015; 19:1146–1154.
- Jia W, Wu Y, Zhang Q, et al. Expression profile of circulating microRNAs as a promising fingerprint for cervical cancer diagnosis and monitoring. Mol Clin Oncol. 2015; 3:851–858.
- Wang C, Cai L, Liu J, et al. MicroRNA-30a-5p inhibits the growth of renal cell carcinoma by modulating GRP78 expression. Cell Physiol Biochem. 2017;43:2405–2419.
- Marchionni L, Hayashi M, Guida E, et al. MicroRNA expression profiling of Xp11 renal cell carcinoma. Hum Pathol. 2017; 67:18–29.
- Li WA, Efendizade A, Ding Y. The role of microRNA in neuronal inflammation and survival in the post ischemic brain: a review. Neurol Res. 2017;1–9.
- Pengcheng S, Ziqi W, Luyao Y, et al. MicroRNA-497 suppresses renal cell carcinoma by targeting VEGFR-2 in ACHN cells. Biosci Rep. 2017; 37.
- Zhao X, Zhao Z, Xu W, et al. Down-regulation of miR-497 is associated with poor prognosis in renal cancer. Int J Clin Exp Pathol. 2015;8:758–764.
- Livak KJ, Schmittgen TD. Analysis of relative gene expression data using real-time quantitative PCR and the 2(-Delta Delta C(T)) method. Methods. 2001; 25:402–408.
- Daniel CR, Cross AJ, Graubard BI, et al. Large prospective investigation of meat intake, related mutagens, and risk of renal cell carcinoma. Am J Clin Nutr. 2012; 95:155–162.
- Pischon T, Lahmann PH, Boeing H, et al. Body size and risk of renal cell carcinoma in the European prospective investigation into cancer and nutrition (EPIC). Int J Cancer. 2006; 118:728–738.
- Van Poppel H, Da Pozzo L, Albrecht W, et al. A prospective, randomised EORTC intergroup phase 3 study comparing the oncologic outcome of elective nephron-sparing surgery and radical nephrectomy for low-stage renal cell carcinoma. Eur Urol. 2011; 59:543–552.
- Hoffmann NE, Gillett MD, Cheville JC, et al. Differences in organ system of distant metastasis by renal cell carcinoma subtype. J Urol. 2008; 179:474–477.
- Medical Research Council Renal Cancer Collaborators. Interferon-alpha and survival in metastatic renal carcinoma: early results of a randomised controlled trial. Lancet. 1999; 353:14–17.
- Tone M, Powell MJ, Tone Y, et al. IL-10 gene expression is controlled by the transcription factors Sp1 and Sp3. J Immunol. 2000; 165:286–291.
- Luo Q, Ma X, Wahl SM, et al. Activation and repression of interleukin-12 p40 transcription by erythroid Kruppel-like factor in macrophages. J Biol Chem. 2004; 279:18451–18456.
- Baeuerle PA, Henkel T. Function and activation of NF-kappa B in the immune system. Annu Rev Immunol. 1994;12:141–179.
- Heo TH, Wahler J, Suh N. Potential therapeutic implications of IL-6/IL-6R/gp130-targeting agents in breast cancer. Oncotarget. 2016; 7:15460–15473.
- Yao X, Huang J, Zhong H, et al. Targeting interleukin-6 in inflammatory autoimmune diseases and cancers. Pharmacol Ther. 2014; 141:125–139.
- Oguro T, Ishibashi K, Sugino T, et al. Humanised antihuman IL-6R antibody with interferon inhibits renal cell carcinoma cell growth in vitro and in vivo through suppressed SOCS3 expression. Eur J Cancer. 2013; 49:1715–1724.
- Guo ST, Jiang CC, Wang GP, et al. MicroRNA-497 targets insulin-like growth factor 1 receptor and has a tumour suppressive role in human colorectal cancer. Oncogene. 2013; 32:1910–1920.
- Wang W, Ren F, Wu Q, et al. MicroRNA-497 suppresses angiogenesis by targeting vascular endothelial growth factor A through the PI3K/AKT and MAPK/ERK pathways in ovarian cancer. Oncol Rep. 2014; 32:2127–2133.
- Han J, Huo M, Mu M, et al. miR-497 suppresses proliferation of human cervical carcinoma HeLa cells by targeting cyclin E1. Xi Bao Yu Fen Zi Mian Yi Xue Za Zhi = Chin J Cell Mol Immunol. 2014; 30:597–600.
- Li W, Jin X, Deng X, et al. The putative tumor suppressor microRNA-497 modulates gastric cancer cell proliferation and invasion by repressing eIF4E. Biochem Biophys Res Commun. 2014; 449:235–240.
- Xie Y, Wei RR, Huang GL, et al. Checkpoint kinase 1 is negatively regulated by miR-497 in hepatocellular carcinoma. Med Oncol. 2014; 31:844.