Abstract
GRAS gene family encodes transcription factors (TFs) which plays a vital role in different processes of plant growth and development of stems and roots, phytohrome-A signal transduction (PAT), gibberellic acid signal transduction (GA), plant response to biotic and abiotic stress conditions and disease resistance. They could be induced by salt and drought stress and in response to pathogen attack. In this research we investigated the expression pattern of the gene encoding MtGRAS TF (Medtr2g026250) by functional GUS assay in the model legume species Medicago truncatula. Transcriptional reporter lines carrying the promoter region of the gene encoding MtGRAS TF fused to GUS and GFP reporter genes were obtained via Agrobacterium-transformation of suspension cultures. The activity of the GUS reporter gene was detected in various parts and tissues of T1 generation of transformed plants, as well as under salinity stress.
Introduction
Some of the most important crops, largely distributed worldwide, sustainable source of human food, animal feed and vegetable oil production belong to the family of leguminous plants. Their ability to establish beneficial symbiotic associations with rhizobial bacteria and to fix atmospheric nitrogen reduces the need for agricultural fertilizers [Citation1, Citation2]. In the last decade, the plant species Medicago truncatula has been the subject of increasing interest as a model plant for molecular and genetic research of leguminous plants. Its genome has been sequenced, which has contributed to resolving some biological questions by using contemporary methods of the functional genomics approaches. One of the basic research tools in plant systems biology are the protocols for effective regeneration and transformation of model legumes for production of stable transgenic plants and their offspring–evolving to a platform for functional analyses for plant growth and development. Another advantage for M. truncatula as a model legume is an actively divided root meristem at their apex [Citation3], important for studying the processes of mycorrhizal and nitrogen fixation.
A vital part of plant functional genomics is transcription factors (TFs). They play role in various physiological processes and regulatory networks [Citation4]. For the first time TFs were discovered in maize, then they were identified in many more plant species [Citation5]. GRAS family proteins are plant-specific TFs with various functions in plant growth and development. They play an essential role in plant growth and development in the response to different stress conditions and nitrogen fixation and arbuscular myccorhizal symbiosis [Citation6–8]. GRAS family proteins are named after the three functional members–GIBBERELLIC ACID INSENSITIVE (GAI), REPRESSOR OF GAI (RGR) and SCARECROW (SCR) [Citation9]. The typical GRAS TFs contain 400–770 amino acid residues with conservative motifs in the C-terminal region [Citation10], whereas others are highly variable in the N-terminal domain [Citation11]. Based on sequence homology and functional similarity, GRAS proteins are divided into subfamilies: DELLA, SCR, PAT1, HAM, LS, SHA and SCL9, each one with different conservative domain and functions [Citation10, Citation12]. GRAS TFs play a vital role in the development of roots and stems, phytohrome-A signal transduction (PAT), gibberellic acid signal transduction (GA) and disease resistance [Citation6, Citation11]. The GRAS family proteins play a role in nodulation and symbiotic nitrogen fixation, and the GRAS proteins NODULATION SIGNALING PATHWAY (NSP1) and NSP2 from M. truncatula bind directly to the promoter of genes associated to nodulation [Citation13, Citation14]. They could be induced by high-salt and drought treatment [Citation15] and in response to various diseases [Citation16].
By using the data from M. truncatula genome and phylogenetic analyses, Song et al. [Citation7] identified 68 MtGRAS genes in silico, classified in 16 groups, localized in 8 chromosomes. They analysed the expression profile of these genes in the response to different abiotic stress conditions. According to the Phytozome (htttp//phytozome.jgi.doe.gov) data base and blast analyses, we established that the MtGRAS gene (previously identified on the basis of Tnt1 insertion mutagenesis by Iantcheva et al. [Citation17] and later cloned) corresponds to one of the genes identified by Song et al. [Citation7], MtGRAS7 (Medtr2g026250). During the GRAIN LEGUMES integrated project – GLIP, FP6 of EU a M. truncatula Tnt1 insertion mutant collection was created by Iantcheva et al. [Citation18]. Randomly selected mutant lines from the Tnt1 insertion mutant collection were screened by transposon display (TD) technique in order to confirm presence of new copies of the Tnt1 retrotransposon. In some of the selected lines Tnt1 insertions were sequenced. The plant genomic region that borders the Tnt1 (Flanking Sequence Tag, FST) could be identified and some of them were located in the coding sequences of different genes, including MtGRAS TF, partially corresponding to insert 6 of line So5945 [Citation17] or Medtr2g026250 MtGRAS7 TF, (htttp//phytozome.jgi.doe.gov).
The MtGRAS7 gene and its promoter were cloned by using GatewayтМ technology (Invitrogen Life Technologies, Inc.) [Citation19]. Vectors for overexpression and downregulation of MtGRAS7, as well as transcriptional-reporters vectors were constructed.
Iantcheva et al. [Citation20] developed an efficient procedure for Agrobacterium-mediated transformation of M. truncatula cv. Jemalong 2HA by cell suspension culture. M. truncatula transcriptional reporter plants were created: transgenic plants carrying the promoter region of the gene encoding MtGRAS7 TF (MtGRAS7 promoter fused to the reporter genes GUS and GFP).
The main objective in the present research was to establish the expression pattern of MtGRAS7 during plant growth and development of the model legume M. truncatula before and after salinity stress induction. The activity of GUS reporter gene under the control of MtGRAS7 promoter was traced in different tissues and plant organs of T1 transgenic plants expressing the GUS gene. For this purpose transcriptional reporter plants were grown in a hydroponic system. After treatment, GUS signal was observed in the root tips, secondary root branches and leaves of the NaCl-treated and non-treated (control) plants. The intensity of the GUS signal decreased with increasing the time for treatment.
Materials and methods
Cloning and plasmid construction
To construct MtGRAS7 recombinant plasmids, we used GATEWAY system technology [Citation19]. The detailed cloning protocol and plasmid construction was described in Iantcheva et al. [Citation20]. Verified plasmids with transcriptional reporter of MtGRAS7 were then transferred to Agrobacterium tumefaciens strain C58C1, maintained on solid YEB medium (nutrient broth; 1.5% agar) supplemented with 100 mg L−1 rifampicin, 100 mg L−1 spectinomycin and 50 mg L−1 gentamicin.
Plant material
As plant material we used T1 transgenic seeds from MtGRAS7 transcriptional reporter lines of the model legume plant M. truncatula and wild-type (WT) seeds from M. truncatula cv. ‘Jemalong 2HA’ [Citation21]. The transgenic plants were created via genetic transformation of M. truncatula plants by the A. tumefaciens system. The transformation protocol and all of the developmental phases were described in Iantcheva et al. [Citation20]. The plants with well-developed roots were screened by polymerase chain reaction (PCR) for the presence of the Km resistance gene (nptII). The following primers were used to amplify a 550 bp fragment of the nptII gene: FW 50-GAA CAA GAT GGA TTG CAC GC and REV 50-GAA GAA CTC GTC AAG AAG GC. The WT plants used in this study are in vitro regenerated plants, produced and grown and set for seed production in the same conditions and at the same time as the transgenic lines.
In vitro growth conditions
A pool of 35 T1 seeds from 3 independent MtGRAS7 transcriptional reporter lines and 35 seeds from WT plants of M. truncatula were surface sterilized according to the protocol of Revalska et al. [Citation22] and stacked on sterile filter paper (Whatman). The seeds were placed in Petri dishes on Murashige and Skoog (MS) basal medium [Citation23] supplemented with 50 mg mL−1 Km in darkness at 4 °C for 48 h. The progeny was grown for 22 days under in vitro conditions: temperature 23–24°С, relative humidity 70–80%, photoperiod 16/8 h (day/night), light intensity 150–300 mol m−2 s−1. Afterwards the plants were transferred to hydroponic containers or to pods with a zeolite/perlite substrate for nodulation.
Growing legume plants under hydroponic conditions
Ten plants from the MtGRAS7 transcriptional reporter lines and 10 WT plants were transferred to the hydroponic containers containing commercial nutrient solution under constant light and aeration, pH 5.8, temperature 23–24°С and relative humidity 70–80%. The composition of the nutrient solution was as follows: 2 mL L−1 Dutch formula – Advanced hydroponics of Holand – 2 Bloom; 200 µL: L−1 MicroMix-Bio Nova (Premium Fertilizers); 10 mL L−1 CaCl2 × 2H2O. Ten days later the plants were inoculated with Sinorhizobium meliloti 1021 (2 × 108 cells mL−1) by adding the bacterial suspension to hydroponic pots. The nutrient solution was changed once a week. For the GUS assay, root samples were taken at 6 different time points of Rhizobium inoculation (0 h, 6 h, 16 h, 144 h (6 days), 7 days and 50 days). Samples from different plant parts were taken from 45- to 50-day-old plants.
Growing legume plants under salinity stress conditions
Twelve plants from the transcriptional reporter lines and 12 WT plants were transferred to the pods containing substrate for nodulation (zeolite:perlite = 1:1). The plants were watered with the commercial solution every 2 days for 10 days. Afterwards 1.7 mL L−1 Sinorhizobium meliloti 1021 with OD = 0.3 was added to the nutrient solution and watering continued every 2 days for approximately 50 days (until symbiotic nodules developed). To set up the salinity stress experiment, transcriptional reporters and WT plants were transferred to hydroponic containers with 750 mL commercial solution supplemented with 0, 50 and 100 mmol L−1NaCl. Samples of roots, leaves and nodules for GUS assay were taken at 0, 24, 48 and 72 h.
Histochemical staining for GUS activity assays
The expression of GUS reporter gene fused to MtGRAS7 endogenous promoter was traced in different tissues and organs of T1 transcriptional reporter plants cultivated in zeolite:perlite substrate or hydroponic system. Histochemical staining for GUS activity was performed essentially as described previously [Citation24, Citation25]. All samples were collected in 90% acetone for 30 min at 4 °C, subsequently washed with phosphate buffer at room temperature, followed by incubation in GUS solution at 37 °C overnight [Citation22]. The images were taken by using stereomicroscope Nikon G-AL equipped with a camera. The light microscopy for root and leaf epidermal assessment was done with a Carl Zeiss Axio Imager upright microscope equipped with a XC50 digital microscope camera.
Statistical analysis
All of the performed experiments were repeated three times and triplicate assays were done for each experimental dataset.
Results and discussion
In this study we determined the expression pattern of GRAS transcription factor from the model legume M. truncatula identified as MtGRAS7 during plant growth and development before and after Rhizobium inoculation and salinity stress induction. For this purpose we grew a pool of T1 seeds of three independent transgenic lines of M. truncatula transcriptional reporters, as well as WT plants For histochemical analyses we used the T1 progeny of GUS positive transgenic plants of M. truncatula after performing a PCR screen for the presence of the nptII gene.
Expression pattern of MtGRAS7 TF fused to GUS reporter gene after Rhizobium inoculation
The expression pattern of MtGRAS7 was monitored during the development of hydroponically grown MtGRAS7-transcriptional reporter plants (pMtGRAS7::GUS-GFP) of M. truncatula. The results from the GUS assay in root samples taken at 6 different time points of S. meliloti inoculation (from 0 h up to 50 days) are shown in , and those in samples from different plant parts from 45- to 50-day-old plants, in .
Figure 1. Histochemical GUS signal localization in М. truncatula pGRAS::GUS-GFP transcriptional reporter plants before and after Rhizobium inoculation. Signal detection in vascular system of the secondary root branches and leaf petiole (A), elongation zone of main root and lateral roots (B), elongation and meristematic zone of the root tip and vascular system of the root (C and D), infection thread and small vesicles (E), lateral root primordial and in meristematic zone of root nodules (F). Scale bar = 50 µm.
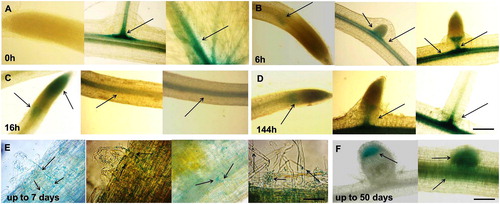
Figure 2. Histochemical GUS signal localization in mature М. truncatula pGRAS::GUS-GFP transcriptional reporter plants grown in hydroponic containers. Signal detection in the vascular system of leaves and in petiole (A, B and C), root tip and secondary root primordium (D), root colummela (E), vascular system of the roots (F), root nodules (G), nodules vasculature (H), meristematic zone of the induced nodules (I and J). Scale bar = 0.50 to 3 cm.
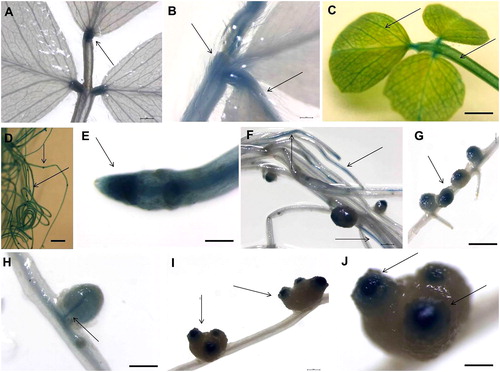
In young plants, GUS signal was detected in the vascular system of the roots and leaves and in the elongation and meristematic zone of roots. displays the expression of the GUS marker gene under the control of the MtGRAS7 promoter before Rhizobium inoculation, where the signal was present in the vascular system of the secondary root branches and the leaf petiole. GUS signal was not detected in the meristematic and elongation zone of the main root cap. At the 6 h after inoculation, there was a weak GUS signal in the elongation zone of the main root and in very small lateral roots. In the bigger lateral roots, the signal had the form of a scapula and started forming a ring (). At 16 h, the gene expression was localized in the elongation and meristematic zone of the root tip and the vascular system of the root (. At 6 days, we observed a very well formed ring above the elongation zone and in the meristematic zone of the roots. MtGRAS7 expression was detected in the vascular system along the root (. Up to 7 days after S. meliloti inoculation, GUS expression was detected in the infection thread formed in the root hair of transcriptional reporter plants, and exited the root hair cell and directed toward the cortex as a thread. A few days later, the threads ramified into the dividing cells of the nodule primordium (. The expression of MtGRAS7 was also detected in small vesicles trafficking trough the root toward the root hairs (. shows the GUS signal in the lateral root primordial and in the meristematic zone of root nodules taken by light microscopy. These results suggest that the bacterial infection induces MtGRAS7 gene expression and confirmed the study of Smit et al. [Citation26] for expression of GRAS family genes during the nodulation of M. truncatula.
In hydroponically grown mature plants (45–50-day-old), the GUS reporter gene under the control of the MtGRAS7 promoter was expressed in the actively dividing zones of roots and leaves, suggesting that it plays a role in plant development. The signal was localized in the vascular system of leaves and in petiole (), in the root tip and secondary root primordium (), root columella () and vascular system of the roots (). MtGRAS7 is a part of the PAT1 subfamily [Citation7] genes, regulating phytochrome-A signal transduction and highly expressed in the photosynthetic tissues of M. truncatula. These genes are involved in various morphological characteristics during plant development. Iantcheva et al. [Citation20] showed GUS and GFP expression in plants in vitro and T1 progeny of MtGRAS7 transformed plants. The activity of the GUS reporter gene under the control of the GRAS promoter was observed in the roots, leaves and stems of in vitro-derived plants [Citation20].
Strong MtGRAS7 expression was observed in the nodules vasculature () and in the meristematic zone of the induced nodules (). The GRAS proteins NODULATION SIGNALING PATHWAY1 (NSP1) and NSP2 from M. truncatula bind directly to the promoter of genes associated with nodulation [Citation13, Citation14]. Middleton et al. [Citation27] has shown that an ERF transcription factor, ERF Required for Nodulation (ERN), is necessary for Nod factor–induced gene expression and spontaneous nodulation activated by the calcium- and calmodulin-dependent protein kinase (DMI3), which is a component of the Nod factor signaling pathway. The team proposed that ERN functions downstream of DMI3 to activate nodulation gene expression.
Expression pattern of MtGRAS7 TF fused to GUS reporter gene before and after salinity stress
In MtGRAS7 transcriptional reporter plants before and after salinity stress, GUS signal was observed in root tips, secondary root branches and root nodules, and in leaf epidermal cells (). Strong expression was observed in the areas of injury in the leaves. In transcriptional reporter plants used as a control without salt supplementation in the solution (, MtGRAS7 expression was observed in the vascular system of roots, secondary buds, in meristematic zone of root nodules, in the epidermal cells of the roots and petiole and leaf and at the place of the wounding. Twenty-four hours after addition of 50 mmol L−1 NaCl, we observed expression in the same plant parts (), but in the plants treated with 100 mmol L−1 NaCl the intensity of the GUS signal was higher in the areas of leaf injury (). Then, 48 h after stress induction, the gene expression in the meristematic zones of root nodules () was stronger compared to that in the control plants (. GUS intensity was lower at 72 h. Some point expression was observed in the roots, leaves and nodules after stress induction. The results showed that the higher salt concentration activated the expression of MtGRAS7. GRAS TFs participate in plant responses to biotic and abiotic stress conditions. They could be induced by high-salt and drought treatment [Citation15] and in response to various diseases [Citation16]. In silico research of Song et al. [Citation7] showed induction of MtGRAS genes (PAT1 subfamily) in the response to various stress conditions, including cold and freezing. These results support the vital role of MtGRAS genes in the regulation of M. truncatula plant response to stress.
Figure 3. Histochemical GUS signal localization in М. truncatula pGRAS::GUS-GFP transcriptional reporter plants before and after salinity stress. Signal detection in control plants (A), plants exposed to 50 mmol L−1 NaCl (B) or 100 mmol L−1 NaCl (C). Scale bar = 0.50 to 3 cm.
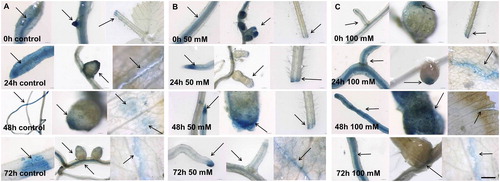
By using light microscopy we screened the roots and leaves of MtGRAS7 transcriptional reporter plants treated with 50 mmol L−1 NaCl for 72 h. It is interesting to note the point expression in the epidermal cells of roots () and leaves (). Some of the root cells were filled with small blue vesicles, which are most likely to be transported out of the cells. After induction of salinity stress, the number of these vesicles increased dramatically. In the roots they were with cellular localization, arranged in rows (). According to Hirsh and Oldroyd [Citation6] approximately 17% of the transcription factors expressed in the roots are able to move from cell-to-cell by trafficking through plasmodesmata. Cell-to-cell movement of transcription factors is required for normal development. Sometimes vesicles could be packed in bigger spheres () which separate from the roots together with some single beads toward the root hairs (). In leaves we observed similar structures, packed or as single beads inside the epidermal cells ().
Figure 4. Light microscopy for histochemical GUS signal localization in М. truncatula pGRAS::GUS-GFP transcriptional reporter plants after salinity stress with 50 mmol L−1 NaCl. Signal detection in root epidermal cells (A and B), root hairs (C and D), leaf epidermal cells (E and F). Scale bar = 0.50 to 3 cm.
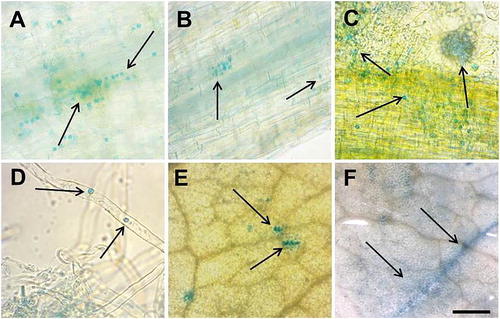
A procedure for developing transgenic M. truncatula plants with overexpression and downregulation of MtGRAS7 is in progress. The newly created stable transformants will be used for studies on the role of the MtGRAS7 gene in the processes of symbiotic nodulation, formation of leaves, flowers and seeds in different stages of plant development in normal conditions and conditions of abiotic stress. In general, knowledge gained from studies into the structure and function of genes, biochemical features and metabolic pathways in model legumes provide an approach to the improvement of the agronomic characteristics of leguminous crops such as alfalfa (Medicago sativa), pea (Pisum sativum) and clover (Trifolium) [Citation28]. Such comparative studies provide important information and contribute to the economic improvement of other crop species, such as tomato, sunflower, cotton, corn and rice [Citation29, Citation30].
Conclusions
The results in this study revealed that the MtGRAS7 gene from M. truncatula is involved in plant development. MtGRAS7 especially participates in the processes of root development and symbiotic nodulation, as suggested by the tissue-specific expression. Also MtGRAS7 was expressed after salt treatment. Furthermore, this study provides a solid foundation for further functional analysis of GRAS genes. Identifying and studying GRAS family TFs in the response to stress will provide valuable information that can be used to improve the quality of leguminous crops.
Disclosure statement
No potential conflict of interest was reported by the authors.
Additional information
Funding
References
- Graham PH, Vance CP. Legumes: importance and constraints to greater use. Plant Physiol. 2003;131:872–877.
- Varshney RK, Close TJ, Singh NK, et al. Orphan legume crops enter the genomics era! Curr Opin Plant Biol. 2009;12:202–210.
- Xiao TT, Schilderink S, Moling S, et al. Fate map of Medicago truncatula root nodules. Development 2014;141:3517–3528.
- Sun X, Jones WT, Rikkerink EH. GRAS proteins: the versatile roles of intrinsically disordered proteins in plant signaling. Biochem J. 2012;442:1–12.
- Huang W, Xian Z, Xia K, et al. Genome-wide identification, phylogeny and expression analysis of GRAS gene family in tomato. BMC Plant Biol. 2015;15:209–226.
- Hirsch S, Oldroyd GE. GRAS-domain transcription factors that regulate plant development. Plant Signal Behav. 2009;4:698–700.
- Song L, Tao L, Cui H, et al. Genome-wide identification and expression analysis of the GRAS family proteins in Medicago truncatula. Acta Physiol Plant. 2017;39:93. [cited 2019 Jun 02]
- Ho-Plágaro T, Molinero-Rosales N, Flores DF, et al. Identification and expression analysis of GRAS transcription factor genes involved in the control of arbuscular mycorrhizal development in tomato. Frontiers in Plant Sci. 2019;10:268.
- Sun X, Xue B, Jones WT, et al. A functionally required unfoldome from the plant kingdom: intrinsically disordered N-terminal domains of GRAS proteins are involved in molecular recognition during plant development. Plant Mol Biol. 2011;77:205–223.
- Bolle C. The role of GRAS proteins in plant signal transduction and development. Planta 2004;218:683–692.
- Lu J, Wang T, Xu Z, et al. Genome-wide analysis of the GRAS gene family in Prunus mume. Mol Genet Genomics. 2015;290:303–317.
- Li M, Sun B, Xie F, et al. Identification of the GRAS gene family in the Brassica juncea genome provides insight into its role in stem swelling in stem mustard. Peer J. 2019;7:e6682.
- Hirsch S, Kim J, Munoz A, et al. GRAS proteins form a DNA binding complex to induce gene expression during nodulation signaling in Medicago truncatula. Plant Cell. 2009;21:545–557.
- Kaló P, Gleason C, Edwards A, et al. Nodulation signaling in legumes requires NSP2, a member of the GRAS family of transcriptional regulators. Science 2005;308:1786–1789.
- Ma H-S, Liang D, Shuai P, et al. The salt- and drought-inducible poplar GRAS protein SCL7 confers salt and drought tolerance in Arabidopsis thaliana. J Exp Bot. 2010;61:4011–4019.
- Mayrose M, Ekengren SK, Melech-Bonfil S, et al. A novel link between tomato GRAS genes, plant disease resistance and mechanical stress response. Mol Plant Pathol. 2006;7:593–604.
- Iantcheva A, Vassileva V, Ugrinova M, et al. Development of functional genomic platform for model legume Medicago truncatula in Bulgaria. Biotechnol Biotechnol Eq. 2009;23:1440–1443.
- Iantcheva A, Chabaud M, Cosson V, et al. Osmotic shock improves Tnt1 transposition frequency in Medicago truncatula cv Jemalong during in vitro regeneration. Plant Cell Rep. 2009;28:1563–1572.
- Karimi M, Inze D, Depicker A. GATEWAY vectors for Agrobacterium-mediated plant transformation. Trends Plant Sci. 2002;7:193–195.
- Iantcheva A, Revalska M, Zehirov G, et al. Agrobacterium-mediated transformation of Medicago truncatula cell suspension culture provides a system for functional analysis. In Vitro Cell Dev Biol-Plant. 2014;50:149–157.
- Nolan KE, Rose RJ, Jr G. Regeneration of Medicago truncatula from tissue culture: increased somatic embryogenesis using explants from regenerated plants. Plant Cell Rep. 1989;8:278–281.
- Revalska M, Vassileva V, Zechirov G, et al. Is the auxin influx carrier LAX3 essential for plant growth and development in the model plants Medicago truncatula, Lotus japonicus and Arabidopsis thaliana? Biotechnol Biotechnol Equip. 2015;29:786–797.
- Murashige T, Skoog F. A revised medium for rapid growth and bio assays with tobacco tissue cultures. Physiol Plant. 1962;15:473–497.
- Jefferson RA, Kavanagh TA, Bevan MW. GUS fusions: beta-glucuronidase as a sensitive and versatile gene fusion marker in higher plants. EMBO J. 1987;6:3901–3907.
- Willemsen V, Wolkenfelt H, de Vrieze G, et al. The HOBBIT gene is required for formation of the root meristem in the Arabidopsis embryo. Development 1998;125:521–531.
- Smit P, Raedts J, Portyanko V, et al. NSP1 of the GRAS protein family is essential for rhizobial Nod factor-induced transcription. Science 2005;308:1789–1791.
- Middleton PH, Jakab J, Penmetsa RV, et al. An ERF transcription factor in Medicago truncatula that is essential for Nod factor signal transduction. Plant Cell. 2007;19:1221–1234.
- Young ND, Udvardi M. Translating Medicago truncatula genomics to crop legumes. Curr Opin Plant Biol. 2009;12:193–201.
- Revalska M, Vassileva V, Goormachtig S, et al. Recent progress in development of a Tnt1 functional genomics platform for the model legumes Medicago truncatula and Lotus japonicus in Bulgaria. Curr Genomics. 2011;12:147–152.
- Iantcheva A, Revalska M, Zehirov G, et al. Tnt1 retrotransposon as an efficient tool for development of an insertional mutant collection of Lotus japonicus. In Vitro Cell Dev Biol-Plant. 2016;52:338–347.