Abstract
The aim of this study was to investigate a novel means of treating piglet diarrhoea caused by enterotoxigenic Escherichia coli (ETEC) strain K88 using traditional Chinese medicine (TCM) fermented by selected probiotic strains. Six types of TCMs were determined to be efficient at inhibiting ETEC growth. In addition, two types of probiotics, Lactobacillus 0111 and 0115, were shown to be the most efficient at inhibiting ETEC. The results of studies with mice indicated that the administration of individual TCMs or probiotic strains increased the growth of mice to a different extent. Specifically, the fermentation of Fructus mume, Galla chinensis and Herba taraxaci by the Lactobacillus 0111 strain enhanced their antibacterial ability compared with traditional Jade screen, possibly due to the presence of a compound producing an absorption peak with a retention time of 19.5 min by HPLC. The fermentation of TCMs by probiotic strain provides a novel approach to relieve the effects of the ETEC strain K88.
Introduction
Escherichia coli-associated diarrhoea in piglets is an infectious disease with high morbidity and mortality, causing significant economic losses to the pig industry [Citation1]. This disease is primarily caused by enterotoxigenic Escherichia coli (ETEC) [Citation2], with K88 being the most well studied serotype [Citation3, Citation4]. Antibiotics are primarily used to treat this disease, the long-term overuse of which leads to antibiotic resistance in pathogens. Because the efficacy of antibiotics is uncertain, it is important to identify new treatments. Traditional Chinese medicine (TCM) and probiotics have no side effects, generate no residues and do not promote resistance, making them an ideal substitute for antibiotics with wide application prospects [Citation5, Citation6].
As one of the most widely used probiotics, Lactobacillus can effectively regulate both intestinal development and flora balance, helping to prevent and treat some early infections caused by specific bacterial pathogens [Citation7]. The first step ETEC K88 uses to invade host organisms is the colonization in the small intestine [Citation8, Citation9]. The primary function of Lactobacillus is in the competitive exclusion of pathogenic bacterial adhesion and the production of a variety of antibacterial substances [Citation10]. Furthermore, some probiotics can enhance immunity by producing nonspecific immune regulatory factors and interferon and by improving the immunoglobulin concentration and macrophage activity. Previous studies have shown that some probiotics can reduce the permeability of the small intestine, enhance the specific mucosal immune response and enhance the activities of IgA and IgM to repair the intestinal barrier function [Citation11, Citation12].
TCMs contain a variety of active ingredients, including alkaloids, terpenoids, acids, ketones, aldehydes, phenols and volatile oils [Citation13]. These active ingredients can inhibit bacterial adhesion and inflammatory responses, disrupting its ability to produce enterotoxin and having a therapeutic role in piglet diarrhoea [Citation14]. Furthermore, these active components can promote immune function. Biopolysaccharide drugs can activate T cells and enhance immune function. Additionally, some TCMs containing plant lectin can increase the total number of lymphocytes and other white blood cells and promote T-cell division and reproduction. Furthermore, some TCM active ingredients have an effect on nucleic acid and cyclic nucleotide systems to improve immune function decline, improving the natural defense systems of the body [Citation15]. Fermentation is a traditional processing method of TCMs, which has been shown to provide unparalleled advantages in improving drug efficacy, producing new compounds and reducing drug toxicity. Increasing numbers of studies have shown the use of microorganisms to improve the efficacy, taste and toxicity of TCMs. For example, the oral administration of probiotic-fermented red ginseng to diabetic mice significantly decreased the blood glucose levels by approximately 62.5% in the fasting state and induced a significant increase in glucose tolerance by approximately 10.2% compared to the control mice [Citation16]. In another study, Salvia miltiorrhizae fermented by Geomyces luteus was shown to significantly improve its antioxidant activity and total phenolic content [Citation17].
The aim of this study was to obtain TCM and probiotics with good antibacterial activity and immune enhancement capacity. A total of 21 types of TCM and 8 Lactobacillus strains were screened via in vitro and in vivo tests. Using the selected TCM and Lactobacillus strains, changes in organic acids and unknown components of probiotic-fermented TCMs were studied, the results of which provide a basis for the use of probiotic-fermented Chinese medicine preparations for piglets with ETEC-induced diarrhoea.
Materials and methods
Bacterial strains and culture conditions
ETEC strain K88 was obtained from the China Institute of Veterinary Drug Control. A single bacterial colony was inoculated into TSB broth (HopeBiol, Qingdao, China) and incubated at 37 °C with vigorous shaking overnight, after which the cultured bacteria were diluted 1:1000 in 0.9% NaCl solution for subsequent use. Eight Lactobacillus strains that had been preserved by Shandong BaoLai-LeeLai Bioengineering Co., Ltd., including Lactobacillus plantarum 0001, L. casei 0003, Enterococcus faecium 0012, L. plantarum 0015, L. plantarum 0020, L. rhamnosus 0031, L. rhamnosus 0038 and L. acidophilus 0111, were cultured statically for 24 h in de Man-Rogosa-Sharpe (MRS) broth medium (HopeBiol, Oingdao, China) at 37 °C and then stored at 4 °C. The culture suspension was directly used for subsequent antibacterial tests.
Preparation of TCMs
According to the Chinese Dictionary of TCM, 5767 varieties of Chinese herbal medicines are known, ranking first in the world, of which nearly 200 are known to affect immunocompetence. By referring to the literature, 21 types of TCMs were identified as primary targets for study and were purchased from the YongchunTai’an city Shandong Tong Pharmaceutical Co. Ltd. and Yongxin pharmacies, including Radix pulsatillae, Cortex phellodendri, Fructus mume, Rhizoma coptidis, R. atractylodis macrocephalae, Galla chinensis, Flos lonicerae, Herba taraxaci, R. isatidis, C. fraxini, R. astragali seu hedysari, F. forsythiae, Poria, R. codonopsis, Folium isatidis, R. bupleuri, Pericarpium granati, H. portulacae, H. andrographis, R. saposhnikoviae and R. scutellariae. Single TCM extracts were prepared using the traditional water extraction method, and the liquids were concentrated to 1 g/mL and stored in a refrigerator at 4 °C. Traditional TCM Jade screen (R. astragali seu hedysari: R. atractylodis macrocephalae: R. saposhnikoviae; 3:1:1) from the Chinese Pharmacopoeia was prepared in the same manner as single TCM preparations.
Bacteriostatic assays
The Oxford cup method was used for bacteriostatic assays in this study. Vernier caliper was used to measure the inhibitory zones. The bacteriostatic effects of the TCMs were scored as follows: inhibitory zones of ≥20, 15 ∼ 19, 10 ∼ 14 and <10 mm indicated extreme, high, moderate and low sensitivity, respectively.
Animals and experimental design
All experimental procedures and animal management procedures complied with the requirements of the Animal Care and Ethics Committees of Jilin Agriculture University (JLAU08201608). One hundred and sixty Kunming mice (18 ∼ 20 g, half male and half female) were obtained from the Shandong Taibang Biological Group Animal Feeding Center. After adapting for 2 days, the mice were randomly divided into 16 groups with 10 mice in each group, and the initial weight of each group was recorded. Eight groups (1 ∼ 8) of the 16 groups were intragastrically administered with normal saline, Jade screen, R. codonopsis, F. mume, G. chinensis, H. taraxaci, Lactobacillus 0015, Lactobacillus 0111 (the appropriate concentration and dosage of the treatment as shown ) without cyclophosphamide (Cy) injection for 10 days. The remaining eight groups (1+∼8+) were carried out under the same drug management while injected with 75 mg/kg cyclophosphamide (Shanxi Powerdone Pharmaceutics Co., Ltd.) on the 3rd, 5th, 7th and 9th days, respectively. The test was completed for 10 days, samples were collected on the 11th day.
Table 1. Experimental design.
Blood and tissue sample collection
Two days after the last administration of Cy, the final weight was recorded and weight gain rate was calculated. In addition, blood samples from the eyes of the mice were collected, and 20 μL of each blood sample was placed in a 1.5 mL centrifuge tube containing EDTA-K2 for white blood cell (WBC), red blood cell (RBC), haematocrit (HCT), mean cell haemoglobin (MCH), mean cell volume (MCV) analysis at the Taian City Central Hospital of Shandong Province in China using an MEK-63 18 K photoelectric automatic blood analyzer (Japan). In addition, 0.5 mL of blood sample were transferred to separate tubes to obtain serum by centrifugation at 3000 rpm for 20 min, which were subsequently stored at –20 °C for IgA and IgG analysis. The spleen, liver and thymus samples were harvested with dissection shears and used to calculate the organ indices. Organ index = organ weight/final body weight × 100. The results are reported as mean values ± SEM (n = 10) and were analyzed using one-way analysis of variance (ANOVA) followed by Duncan’s posttest.
Detection of serum immunoglobulin levels by ELISA
The concentrations of IgA and IgG in serum samples were measured using commercially available ELISA kits specific for mouse IgA and IgG (Shanghai Bang Yi Biological Technology Co., Ltd.) according to the manufacturer’s instructions.
Determination of intestinal floral diversity
Denatured gradient gel electrophoresis (DGGE) was performed as described previously to determine the diversity of the intestinal flora [Citation18]. An E.Z.N.A.DNA kit was used to extract the total DNA from the faeces of the mice. The V3 region of the bacterial 16S rDNA gene was amplified with the primers 338F-GC (5′-CGCCCGCCGCGCGCGGCGGGCGGGGCGGGGGCACGGGGGGTACGGGAGGCAGCAG-3′) and 518R (5′-ATTACCGCGGCTGCTGG-3′). For the PCR, 30 PCR cycles were used (95 °C 30 s, 56 °C 30 s and 72 °C 60 s). The denaturing gradient was formed with two 8% acrylamide (acrylamide-bis 37.5:1) with denaturing gradients ranging from 30% to 70% for analysis of the amplified 16S rRNA fragments. The 100% denaturant solution contained 40% (v/v) deionized formamide and 7 M urea. Gels were run in 0.5× Tris/Acetate/EDTA buffer at 60 °C for 11 h at 60 V. The gels were stained with silver staining solution. Image Lab software, version 5.0 (Bio-Rad) was used for the identification of bands and normalization of band patterns from DGGE gels.
Determination of organic acid content associated with the antibacterial activity of probiotic-fermented TCMs
The TCMs H. taraxaci, F. mume and G. chinensis were used for further fermentation at a ratio of 6:1:1 using the Lactobacillus strain 0111 at a ratio of 4% at 37 °C for 24 h (pH = 5.5), with the individual Lactobacillus 0111 culture and TCM water extraction included as controls. At the end of fermentation, the Oxford cup method was used to evaluate the inhibitory effects of the fermented TCMs on the ETEC strain K88. High-performance liquid chromatography (HPLC) was used to compare the changes in the chromatographic peaks before and after fermentation using the lactic acid bacteria fermentation broth as a blank control. The water extractions of each TCM were also included as controls.
Statistical analysis
The data were evaluated using one-way ANOVA, and Tukey’s multiple comparison test was used to assess differences among the means of the treated groups. A probability value of <0.05 was considered significant. Quantity One software was used to analyze the DGGE profiles and perform cluster analysis. Statistical analysis of the data sets was performed using MATLAB 2013.
Results and discussion
TCMs and micro-ecological preparations have globally become a focus of research as they are purely natural feed additives, which are non-toxic, safe and convenient, with comprehensive function and no drug residues. In addition, TCMs contain a variety of active ingredients including alkaloids, terpenoids, acids, ketones, aldehydes, phenols and volatile oil, which lead to immune activity and bacteriostatic activity. The antimicrobial activity of Lactobacillus was associated with the production of metabolites or the secretion of mucin [Citation18–20].
The results of the TCM antibacterial tests showed that F. mume (26.3 ± 0.50 mm), G. chinensis (23.7 ± 0.26 mm), R. codonopsis (20.0 ± 1.00 mm), P. granati (21.7 ± 0.36 mm), H. taraxaci (15.8 ± 0.85 mm) and R. coptidis (11.3 ± 1.6 mm) contained antibacterial components that exhibited antibacterial activity toward the ETEC strain K88, which is consistent with previously reported results [Citation21, Citation22]. F. mume exhibited a good antimicrobial effect on the ETEC K88 strain, potentially due to its high content of fumaric acid, which was previously shown to inhibit gram-positive and gram-negative bacteria [Citation23–25]. In addition, gallic acid from G. chinensis inhibits bacterial growth by disrupting bacterial cell membranes, resulting in the leakage of cell contents [Citation26].
Eight Lactobacillus strains were tested for their antibacterial activity against ETEC K88, all of which produced zones of inhibition, exhibiting no significant differences. Compared to the other 6 lactic acid bacteria strains, Lactobacillus strains 0015 and 0111 strains were observed to efficiently inhibit the growth of K88, yielding zones of inhibition zone with diameters of 14.6 ± 1.83 and 13.7 ± 0.72 mm, respectively. The antibacterial effects of different Lactobacillus strains toward E. coli K88 were different. Lactic and acetic acid were previously reported to be the primary substances responsible for the inhibition of Lactobacillus [Citation27]. A Lactobacillus strain, isolated from the intestinal mucosa of weaned piglets, capable of producing large amounts of lactic acid further supports this result [Citation28]. The results showed that the antibacterial effects of the metabolites produced by Lactobacillus were better than those of the control group, demonstrating that the fermentation broth contains additional antibacterial ingredients, such as bacteriocin and antimicrobial peptides.
Cy is a strong alkylating agent, which can damage DNA by forming interstrand DNA crosslinks, and thus, it can destroy active immune cells and inhibit immune function. With this regard, it has been widely used as a modelling agent for immunosuppressive model in immunopharmacology. TCMs and probiotics have a direct inhibition or killing effect toward pathogenic bacteria and improve the immunity of livestock and poultry and enhance their resistance to disease [Citation29, Citation30]. Therefore, an immunosuppressed mouse model was established using Cy to investigate the effect of TCMs and probiotics on immune function [Citation31]. The evaluation was done on the growth performance, organ index, blood indices, immunoglobulin and diversity of faecal bacteria.
As shown in , no significant differences were observed in the initial and end weights of the mice in the normal group (P > 0.05). However, comparing the average growth rates of mice, the TCM and probiotics improved the average weight gain rate trends to a different extent, with a 54% weight gain rate observed in the H. taraxaci group, which was close to that observed using the traditional prescription Jade screen (with average weight gain of 57.15%). The average weight gain rate of F. mume and R. codonopsis groups were 52.73% and 51.41%, respectively, and a comparison of two probiotics showed that the average rate of mouse weight increase in the group treated with Lactobacillus 0111 was 51.29%, which was better than that of Lactobacillus 0015.
Table 2. Effects of TCMs and Lactobacillus on the weights of the normal mice (x̄±S, n = 10).
According to the results shown in , the body weights of mice in the normal group decreased significantly after the injection of Cy, with the average rate weight gain decreasing from 48.97% to 16.32%. The average rate of weight gain in each group was higher than that of negative control group after the oral administration of TCM and probiotics. Except for the H. taraxaci group and Lactobacillus 0015 groups, the final weight of each group was significantly higher than that of the control group (P < 0.05). The average weight gain of mice in the F. mume and G. chinensis groups was higher than that of the Jade screen group, 31.9% and 28.8%, respectively. Thus, the oral administration of TCMs and probiotics had a positive role in increasing the average weight gain rate of mice.
Table 3. Effect of TCMs and Lactobacillus on the weights of mice immunosuppressed with cyclophosphamide (x̄±S, n = 10).
Compared to the normal saline group, the groups of mice treated with F. mume, H. taraxaci, R. codonopsis and Lactobacillus 0111 exhibited different degrees of increased spleen index, and the trend was more obvious than Jade screen group. The liver indices for the groups treated with F. mume, H. taraxaci, Lactobacillus 0015, Lactobacillus 0111 and Jade screen were similar, but lower than that of the Jade screen group. Since the spleen, thymus and liver indices could indicate immune function to some extent, the results showed that Chinese herbal medicine and probiotics had a positive effect on most of the immune organ indices of normal mice.
Interestingly, the spleen and thymus indices of mice decreased after injection of Cy, especially the thymus index (). The liver index values were also slightly reduced, with the exception of the R. codonopsis group. The spleen index in the H. taraxaci group was significantly higher than that of the F. mume and Lactobacillus 0111 groups (P < 0.05). Lactobacillus 0015 was superior to the Jade screen in improving the thymus index. In general, the majority of TCMs and probiotics were observed to increase the spleen and thymus indices in immunosuppressed mice.
Table 4. Effect of TCMs and Lactobacillus on the organ indices of mice immunosuppressed with cyclophosphamide (x̄±S, n = 10).
As shown in , in normal mice, the white blood cell (WBC) () and haemoglobin (HGB) () levels decreased in mice fed with TCMs and probiotics compared to the control mice, but the difference was not significant (P > 0.05). The red blood cell (RBC) () levels also decreased, with significant differences observed between the Lactobacillus 0111 and the control groups (P < 0.05), while no significant differences were observed for the other groups (P > 0.05). The average MCV level increased, but the difference was not significant (P > 0.05) and were the highest in the F. mume and the Lactobacillus 0111 groups (). The effect of the treatments on MCH levels was not significant (P > 0.05), with the exception of Lactobacillus 0111 (). In addition, the effect of the treatments on HCT levels was not significant (P > 0.05) (). Generally, TCMs and probiotics exhibited little effect on the assayed blood indices in normal mice.
Figure 1. Effects of TCMs and lactic acid bacteria on the haematological indices of normal mice. Normal mice without Cy were fed five types of TCM (Jade screen, Radix codonopsis, Fructus mume, Galla chinensis, or Herba taraxaci) and two lactic acid bacterial strains (Lactobacillus 01111 and 0015) for 1 week. The normal saline-treated mice represented the untreated control. Blood was collected to examine the WBC (A), RBC (B), HGB (C), MCV (D), MCH (E) and HCT (F) contents. Mean values ± SEM (n = 10). *P < 0.05, compared with the normal saline group.
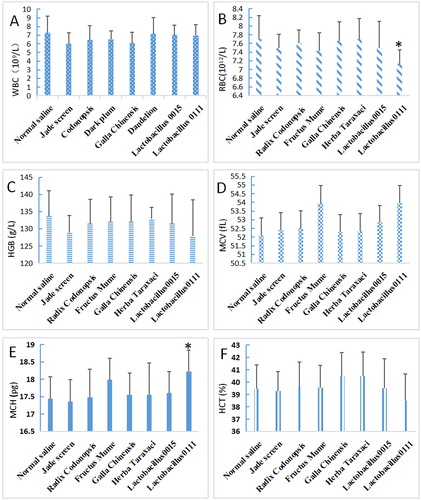
Compared to the normal mice, the WBC, RBC and HGB levels were significantly decreased in the immunosuppressed mice (P < 0.05), whereas the MCV, MCH and HCT levels were not significantly different (P > 0.05). The WBC, RBC and HGB levels in the immunosuppressed mice increased to different degrees after mice were administered TCMs and probiotics, but did not reach the levels observed in the normal mice (). The WBC level in the group treated with F. mume and Lactobacillus 0015 was higher than that of Jade screen-treated group. The RBC and HGB levels in all of the treatment groups were higher than those observed in the group treated with Jade screen, with the exception of Lactobacillus 0015. Compared to the normal control group, the MCV, MCH and HCT levels in the of immunocompromised group were not significantly altered ().
Figure 2. Effects of TCMs and lactic acid bacteria on the haematological indices of mice immunosuppressed with Cy. The mice were treated with five types of TCM (1 g/mL Jade screen, 1 g/mL Radix codonopsis, 0.5 g/mL Fructus mume, 0.25 g/mL Galla chinensis, or 0.5 g/mL Herba taraxaci) and two strains of Lactobacillus (6 × 108 cfu/mL) by intragastric administration for 1 week. From the third day on, the mice were intraperitoneally injected with 75 mg/kg of Cy every other day four times. The mice in the positive control group (normal saline group) were treated with 0.9% saline and provided normal drinking water without any drugs. The mice in the negative control group (normal saline + Cy) were treated with 75 mg/kg of Cy and provided normal drinking water without any drugs. Blood was collected to examine the WBC (A), RBC (B), HGB (C), MCV (D), MCH (E) and HCT (F) contents. Mean values ± SEM (n = 10). *P < 0.05, compared with normal saline group.
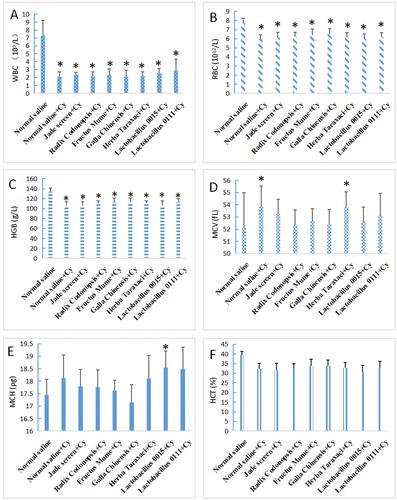
The effects on haematological indices (WBC, RBC and HGB) were significant in the immunosuppressed mice (P < 0.05). TCMs and probiotics had little effect on the physiological indices of blood in normal mice but could promote the growth and improve the organ indices.
Compared the normal control group, the IgA and IgG levels in the serum of immunosuppressed mice were significantly decreased (). For the normal mice, the IgA and IgG levels in serum samples increased after the oral administration of TCMs and probiotics. The IgA levels in the R. codonopsis, G. chinensis, Lactobacillus 0111 group and Lactobacillus 0015 groups were significantly higher than that observed in the blank control group (P < 0.05). In addition, the IgG levels in the Jade screen group was significantly higher than that of the blank control group (P < 0.05). For the immunosuppressed mice, the IgA levels for each TCM and probiotics group was higher than that of the model control group, but the differences were not significant (P > 0.05).With the exception of the R. codonopsis group, the IgG levels in the other TCM and probiotic groups were significantly higher than that of the model control group (P < 0.05). In contrast, the effects of the TCM and probiotics on the IgA content in the normal mice and on the IgG content in the immunosuppressed mice were more significant.
Figure 3. Effects of TCMs and lactic acid bacteria on serum immunoglobulin levels in mice. Groups 1 to 8 were normal mice that were intragastrically administered TCMs or lactic acid bacteria for 1 week. Group 1 was the normal control group without any treatment administration. Groups 1+ to 8+ were mice that were immunosuppressed with 75 mg/kg Cy and administered TCM or lactic acid bacteria. Group 1+ was the immunosuppressed control group, with mice only having received Cy injections. Blood samples were collected on the second day after the Cy injection. The IgA and IgG levels in serum samples were evaluated. The values were compared with those of the normal control group. Mean values ± SEM (n = 10). *P < 0.05, compared with normal saline group. #P < 0.05, compared with normal saline + Cy group.
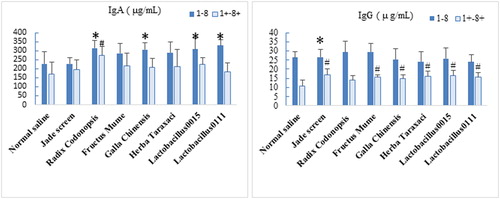
The DGGE results showed that the richness and diversity of the caecal flora decreased after the injection of normal mice with Cy [Citation32]. After the oral administration of TCMs and probiotics, the richness, diversity and evenness of the intestinal microflora of mice improved to different degrees, possibly because some TCM components, such as polysaccharides, can provide nutrition for normal flora and promote the growth of probiotics [Citation33, Citation34]. In addition, the effective TCM components are decomposed and metabolized by enzymes produced by intestinal microorganisms. This phenomenon allows TCMs to be more readily absorbed and utilized and shows how TCMs and probiotics interact and influence each other.
As shown in , the bands observed for the normal mouse group were basically the same as those observed in the non-immunosuppressed groups (1 ∼ 8), with differences in bands being relatively small. In contrast, differences between the immunosuppressed groups (9 ∼ 16) and the non-immunosuppressed groups (1 ∼ 8) were observed. The principal component analysis results showed that the microflora structure of the normal mice was more altered than that of the immunosuppressed mice, indicating that the Cy treatment had an effect on the faecal flora of mice. These results suggest that the immunosuppressive effect of the Cy treatment alters the faecal flora of mice.
Figure 4. DGGE map of the faecal flora of mice. Groups 1 to group 8 were normal mice intragastrically administered TCM or lactic acid bacteria for 1 week. Group 1 was the normal control group without any treatment administration. Groups 1+ to 8+ were mice that were immunosuppressed with 75 mg/kg Cy and administered TCM or lactic acid bacteria. Group 1+ was the immunosuppressed control group, with mice only having received Cy injections.
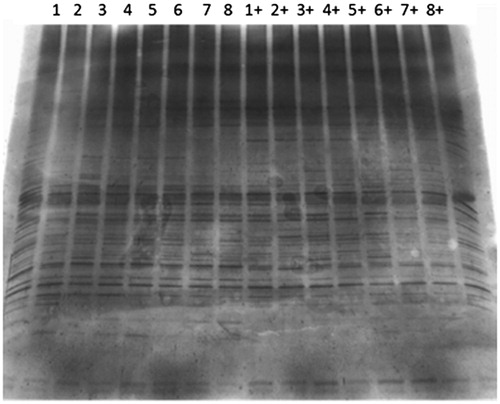
In the non-immunosuppressed mouse groups (1 ∼ 8), Lactobacillus 0111 had the greatest effect on microflora richness, followed by Lactobacillus 0015 and H. taraxaci (). Lactobacillus 0111 had the greatest effect on microbial diversity, followed by H. taraxaci. In terms of microbial evenness, Lactobacillus 0111 had the greatest effect, followed by dandelion. These results suggest that Lactobacillus 0111 and H. taraxaci greatly influenced the faecal flora of non-immunosuppressed mice and could enrich the diversity and quantity of intestinal flora to some extent. Compared to the non-immunosuppressed mice (group 1), Cy decreased the richness, diversity and evenness in immunosuppressed mice (group 1+). After the oral administration of TCMs and probiotics to the immunosuppressed mice, the richness and evenness of the intestinal flora in mice improved. The diversity of the intestinal flora of the immunosuppressed mice was higher than that of the group 1 mice except for the F. mume group. Thus, the results showed that TCMs and probiotics could counteract the effect of Cy on the intestinal flora of mice.
Table 5. Effects of TCMs and lactic acid bacteria on the richness and diversity indices of the faecal microflora of mice.
The fermentation of TCMs using probiotics causes biochemical reactions and elevates health beneficial effects by synergy of the microbial and herbal activities. It has been proved to be one of the promising approaches for antibiotic substitutions, particularly in livestock and poultry breeding industries. Using the Oxford cup method, the fermentation of TCM by Lactobacillus strain 0111 significantly increased the diameter of the ETEC strain K88 zone of inhibition (19.75 ± 0.48 mm) compared with either the Lactobacillus 0111 culture (14.13 ± 0.32 mm) or TCM water extraction (11.10 ± 0.69 mm) alone, indicating that the fermented TCM solution contained additional antibacterial products.
To further identify the component that could be responsible for the observed inhibitory effects, HPLC was performed as described in the Materials and Methods section. The water extractions of original and fermented mixed TCM were compared by HPLC, with the results showing the disappearance of one peak and the presence of a newly formed peak after fermentation (). To further confirm the novelty of this newly produced peak, the solutions from the mixed TCMs before fermentation and the individual TCMs were compared, with the results demonstrating that the retention time of the unknown peak in the water extract of G. chinensis was the same as that in the water extract of the mixed TCMs. In contrast, no similar peaks were observed with the same retention time for the H. taraxaci and F. mume extracts (), indicating that the unknown peak before fermentation came from G. chinensis. In addition, the fermented extracts were also evaluated by HPLC. The results showed that the retention time of the unknown peak in the G. chinensis fermented solution was the same as that present in the mixed TCM fermentation broth, while the unknown peak in the H. taraxaci and F. mume extracts did not produce a peak with the same retention time (), indicating that the unknown peak after fermentation came from G. chinensis.
Figure 5. Determination of composition changes before and after fermentation by HPLC. (A) Chromatographic superposition of organic acids in TCMs before and after fermentation. Black: 0111 bacterial solution; Pink: TCM before fermentation; Blue: TCM after fermentation. (B) Chromatographic superposition diagram of single or mixed TCM water extracts. Black: mixed TCM; Pink: Galla chinensis; Blue: Herba taraxaci; Brown: Fructus mume. (C) Chromatographic superposition diagram of individual TCM fermentation broths and mixed TCM fermentation broth. Black: mixed TCM; Pink: Galla chinensis; Blue: Herba taraxaci; Brown: Fructus mume.
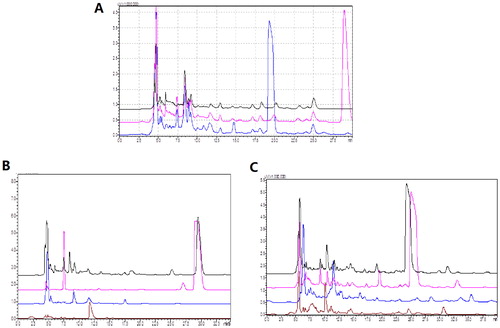
Conclusions
TCMs fermented with Lactobacillus strain 0111 exhibited the ability to inhibit the growth of ETEC K88 more efficiently compared to either the TCM water extractions or Lactobacillus 0111 culture alone, indicating the occurrence of changes in TCMs resulting from fermentation. HPLC was performed to identify the components before and after fermentation. One of the most obvious changes was the generation of a novel peak after fermentation and the loss of a peak that was present before fermentation. To further track the original source of this peak, we compared the three individual extracts of TCMs fermented with Lactobacillus strain 0111, with the results demonstrating that a similar peak was presented in the fermented G. chinensis extract, indicating that Lactobacillus strain 0111 could alter the G. chinensis components during fermentation and may be responsible for the observed increased antibacterial ability.
Disclosure statement
No potential conflict of interest was reported by the authors.
Additional information
Funding
References
- Luppi A, Gibellini M, Gin T, et al. Prevalence of virulence factors in enterotoxigenic Escherichia coli isolated from pigs with post-weaning diarrhoea in Europe. Porc Health Manag. 2016;2:20.
- Do T, Stephens C, Townsend K, et al. Rapid identification of virulence genes in enterotoxigenic Escherichia coli isolates associated with diarrhoea in Queensland piggeries. Aust Vet J. 2005;83:293–299.[pubmedMismatch]
- Moon HW, Hoffman LJ, Cornick NA, et al. Prevalences of some virulence genes among Escherichia coli isolates from swine presented to a diagnostic laboratory in Iowa. J Vet Diagn Invest. 1999;11:557–560.
- Kwon D, Choi C, Jung T, et al. Genotypic prevalence of the fimbrial adhesins (F4, F5, F6, F41 and F18) and toxins (LT, STa, STb and STx2e) in Escherichia coli isolated from postweaning pigs with diarrhoea or oedema disease in Korea. Vet Rec. 2002;150:35–37.
- Bovill R, Bew J, Robinson S. Comparison of selective media for the recovery and enumeration of probiotic yeasts from animal feed. Int J Food Microbiol. 2001;67:55–61.
- Oyofo BA, DeLoach JR, Corrier DE, et al. Prevention of Salmonella typhimurium colonization of broilers with D-mannose. Poult Sci. 1989;68:1357–1360.[pubmedMismatch]
- De Angelis M, Siragusa S, Berloco M, et al. Selection of potential probiotic lactobacilli from pig feces to be used as additives in pelleted feeding. Res Microbiol. 2006;157:792–801.[pubmedMismatch]
- Jin LZ, Zhao X. Intestinal receptors for adhesive fimbriae of enterotoxigenic Escherichia coli (ETEC) K88 in swine-a review. Appl Microbiol Biotechnol. 2000;54:311–318.
- Joller D, Jorgensen CB, Bertschinger HU, et al. Refined localization of the Escherichia coli F4ab/F4ac receptor locus on pig chromosome 13. Anim Genet. 2009;40:749–752. Oct
- Muthukumarasamy P, Holley RA. Survival of Escherichia coli O157:H7 in dry fermented sausages containing micro-encapsulated probiotic lactic acid bacteria. Food Microbiol. 2007;24:82–88.
- Sudo N, Sawamura S, Tanaka K, et al. The requirement of intestinal bacterial flora for the development of an IgE production system fully susceptible to oral tolerance induction. J Immunol. 1997;159:1739–1745.
- Kirjavainen PV, Apostolou E, Salminen SJ, et al. New aspects of probiotics-a novel approach in the management of food allergy. Allergy. 1999;54:909–915.
- Li XB, Wu WX, Su D, et al. Pharmacokinetics and bioavailability of Cefquinome in healthy piglets. J Vet Pharmacol Ther. 2008;31:523–527.[pubmedMismatch]
- Liu X, Liu F, Ma Y, et al. Effect of puerarin, baicalin and berberine hydrochloride on the regulation of IPEC-J2 Cells Infected with enterotoxigenic Escherichia coli. Evid Based Complement Altern Med. 2019;2019:7438593.
- Ding Y, Zeng L, Li R, et al. The Chinese prescription lianhuaqingwen capsule exerts anti-influenza activity through the inhibition of viral propagation and impacts immune function. BMC Complement Altern Med. 2017;17:130.
- Jang SH, Park J, Kim SH, et al. Red ginseng powder fermented with probiotics exerts antidiabetic effects in the streptozotocin-induced mouse diabetes model. Pharm Biol. 2017;55:317–323.
- Xing Y, Cai L, Yin TP, et al. Improving the antioxidant activity and enriching salvianolic acids by the fermentation of Salvia miltiorrhizae with Geomyces luteus. J Zhejiang Univ Sci B. 2016;17:391–398.
- Bakke I, De Schryver P, Boon N, et al. PCR-based community structure studies of bacteria associated with eukaryotic organisms: a simple PCR strategy to avoid co-amplification of eukaryotic DNA. J Microbiol Methods. 2011;84:349–351.[pubmedMismatch]
- van den Broek MFL, De Boeck I, Claes IJJ, et al. Multifactorial inhibition of lactobacilli against the respiratory tract pathogen Moraxella catarrhalis. Benef. Microbes. 2018;9:429–439.
- Plaza-Diaz J, Ruiz-Ojeda FJ, Gil-Campos M, et al. Mechanisms of action of probiotics. Adv Nutr. 2019;10(suppl):S49–S66.
- Yang Q, Wang L, Gao J, et al. Tannin-rich fraction from pomegranate rind inhibits quorum sensing in Chromobacterium violaceum and biofilm formation in Escherichia coli. Foodborne Pathog Dis. 2016;13:28–35.
- Wang S, Fan M, Bian Z. Experimental study of bacteriostatic activity of Chinese herbal medicines on primary cariogenic bacteria in vitro. Zhonghua Kou Qiang Yi Xue Za Zhi. 2001;36:385–387.
- Kwon HA, Kwon YJ, Kwon DY, et al. Evaluation of antibacterial effects of a combination of Coptidis rhizoma, Mume fructus, and Schizandrae fructus against Salmonella. Int J Food Microbiol. 2008;127:180–383. 30
- Pernin A, Bosc V, Maillard MN, et al. Ferulic acid and eugenol have different abilities to maintain their inhibitory activity against Listeria monocytogenes in emulsified systems. Front Microbiol. 2019;10:137.
- Dasagrandhi C, Park S, Jung W-K, et al. Antibacterial and biofilm modulating potential of ferulic acid-grafted chitosan against human pathogenic bacteria. IJMS. 2018;19:2157. DOI:10.3390/ijms19082157
- Borges A, Ferreira C, Saavedra MJ, et al. Antibacterial activity and mode of action of ferulic and gallic acids against pathogenic bacteria. Microb Drug Resist. 2013;19:256–265.
- Tsiloyiannis VK, Kyriakis SC, Vlemmas J, et al. The effect of organic acids on the control of post-weaning oedema disease of piglets. Res Vet Sci. 2001;70:281–285.[pubmedMismatch]
- Ogawa M, Shimizu K, Nomoto K, et al. Inhibition of in vitro growth of Shiga toxin-producing Escherichia coli O157:H7 by probiotic Lactobacillus strains due to production of lactic acid. Int J Food Microbiol. 2001;68:135–140.
- Li Y, Zheng B, Tian H, et al. Yupingfeng Powder relieves the immune suppression induced by dexamethasone in mice. J Ethnopharmacol. 2017;200:117–123.
- Xie Y, Wang L, Sun H, et al. Immunomodulatory, antioxidant and intestinal morphology-regulating activities of alfalfa polysaccharides in mice. Int J Biol Macromol. 2019;133:1107–1114.
- Fu Y-P, Feng B, Zhu Z-K, et al. The polysaccharides from Codonopsis pilosula modulates the immunity and intestinal microbiota of cyclophosphamide-treated immunosuppressed mice. Molecules. 2018;23:1801.
- Tang C, Sun J, Zhou B, et al. Effects of polysaccharides from purple sweet potatoes on immune response and gut microbiota composition in normal and cyclophosphamide treated mice. Food Funct. 2018;9:937–950.
- Wang M, Xie Z, Li L, et al. Supplementation with compound polysaccharides contributes to the development and metabolic activity of young rat intestinal microbiota. Food Funct. 2019;10:2658–2675.[pubmedMismatch]
- Shen H, Gao XJ, Li T, et al. Ginseng polysaccharides enhanced ginsenoside Rb1 and microbial metabolites exposure through enhancing intestinal absorption and affecting gut microbial metabolism. J Ethnopharmacol. 2018;216:47–56.