Abstract
A cellobiohydrolase gene was cloned and analyzed from Auricularia heimuer, and named A-cbh. The cDNA sequence was submitted to GenBank under the accession number MK620906. Sequence analysis showed that the cDNA comprised 1551 bp and encoded a putative polypeptide of 516 amino acids. The molecular weight of this protein was predicted as 54.44 kDa, and the theoretical isoelectric point was 5.24. The recombinant prokaryotic expression vector pET32a-A-cbh was constructed and transformed into Escherichia coli BL21. Sodium dodecyl sulphate polyacrylamide gel electrophoresis indicated that the recombinant protein had molecular size between 66.2 kDa and 94.0 kDa, which was consistent with our expectations because a tag protein of approximately 20 kDa was present in the vector of pET32a. The transcription levels of A-cbh in the mycelium were lower than those in the fruiting bodies. The highest level of A-cbh expression was observed in the primordium, which was 8.65-fold higher than that in the control (mycelia at 6 days). We speculated that A-cbh might be involved in the formation of primordia and the development of fruiting bodies. The results of the present study will form the basis for further research into the function of the cellobiohydrolase in A. heimuer.
Introduction
Edible mushrooms have been exploited for their nutritional and medicinal properties for centuries, and have been used as a significant therapeutic raw material in folk medicines [Citation1]. Auricularia heimuer [Citation2] is an edible and medicinal mushroom that has been widely cultivated for thousands of years in China [Citation3] and is acknowledged as a healthy food. The fruiting bodies contain a high content of carbohydrates, proteins, minerals, and many other nutrient components [Citation4]. In addition, the active polysaccharides of A. heimuer have been used as drugs with antioxidant, anticoagulant, anti-tumour, hypolipidaemic and hypoglycaemic activities [Citation5–9].
Cellulose is a linear homopolysaccharide consisting of glucose subunits linked by β-1,4-glycosidic bonds, comprising the major constituent of most plant cell walls [Citation10]. Cellulose molecules consist of highly crystalline regions and amorphous regions, and this complex structure of cellulose makes it difficult to degrade [Citation11]. The degradation of cellulose is accomplished by cellulase [Citation12], which is a complex enzyme, including endoglucanase (EC: 3.2.1.4), cellobiohydrolase (EC: 3.2.1.91), and β-glucosidases (EC: 3.2.1.21) [Citation13]. First, the endoglucanase binds to the cellulose polymer and cuts randomly in the non-crystalline zone to produce new sugar chain ends. The cellobiohydrolase then acts on the reductive ends or the non-reductive ends to produce cellobiose. Finally, under the action of β-glucosidase, cellobiose is hydrolyzed to glucose. Under the coordination of these three enzymes, cellulose is eventually hydrolyzed into small glucose molecules, which are then absorbed and utilized by microorganisms.
Cellobiohydrolase is classified into two glycoside hydrolase (GH) families (6 and 7). In fungi, cellobiohydrolases account for 70–80% of the total enzyme system, and the cellobiohydrolases of the GH7 family are considered as the main enzymes because they provide the majority of the hydrolytic power during the process of cellulose degradation [Citation14–16]. Cellobiohydrolases are key components in the multienzyme cellulase complex and have been extensively studied in Trichoderma reesei; however, research into the cellobiohydrolase in A. heimuer has not been reported.
In the present study, we cloned a cellobiohydrolase gene (A-cbh) that encodes a protein with the conserved domain of GH7 from A. heimuer. Expression of A-cbh in Escherichia coli BL21 produced a clear protein band on the sodium dodecyl sulphate polyacrylamide gel electrophoresis (SDS-PAGE) gel. In addition, the transcription levels of A-cbh in different stages of A. heimuer were investigated.
Materials and methods
Strain and culture conditions
The A. heimuer dikaryotic strain, heihouyuan, was provided by the Forest Protection Department of the Northeast Forestry University. The mycelia were maintained at 25 °C on potato dextrose (PD) agar plates and kept in the dark for 7 d. The mycelia were then inoculated into liquid PD medium and the cultures were allowed to grow in a shaking incubator at 180 r/min and 25 °C. Finally, A. heimuer was inoculated into sawdust culture medium (78% sawdust, 20% wheat bran, 1% bean flour, and 1% corn flour) to obtain fruiting bodies. The conditions for the formation of fruiting bodies were created by punching holes in the surface of the bag and placing them in a culture room at 25 °C, with a relative humidity of 90 ± 5%, and more than 400 lux of light.
E. coli DH5α and BL21 were cultured on Luria-Bertani (LB) medium at 37 °C.
RNA extraction and cDNA synthesis
Mycelia were collected after 7 d of growth in liquid PD medium and preserved at −80 °C. Total RNA of A. heimuer was isolated using an RNAprep pure plant kit (Tiangen, Beijing, China) according to the manufacturer’s instructions. The quality and quantity of RNA were assessed by agarose gel electrophoresis and checked using a BioPhotometer D30 (Eppendorf, Hamburg, Germany). cDNA was synthesized using a PrimeScript™ 1st strand cDNA synthesis kit (Takara, Dalian, China).
Cloning of the A-cbh gene
Combining the genomic data of A. heimuer present in the NCBI database (GenBank accession number: NEKD00000000.1) and the transcriptome data obtained in early stage by our laboratory, the sequence of the cellobiohydrolase gene was screened out. Specific primers A-cbhF and A-cbhR () were designed according to the full-length sequence, and the cDNA was used as the template. Premix Taq (Takara) was used in a 20 μL reaction mixture including 10 μL of 2 × premix Taq, 2 μL of 10 mmol/L primers, and an appropriate amount of template. The polymerase chain reaction (PCR) parameters were set as follows: predegeneration at 94 °C for 5 min; 38 cycles of denaturation at 94 °C for 30 s, annealing at 58 °C for 40 s, and extension at 72 °C for 40 s; and a final extension at 72 °C for 10 min (Bio-Rad, Hercules, CA, USA). The PCR product was purified and cloned into pMD18-T (Takara) for sequencing (performed by TSINGKE, Harbin, China).
Table 1. Primers used in this research.
Sequence analysis
ExPASy ProtParam (Swiss) (https://www.expasy.org/) was used to analyze the molecular weight and isoelectric point of the deduced protein. TMHMM Server v. 2.0 (Denmark) was used for transmembrane region analysis. The conserved area and protein family prediction were carried out using an NCBI (US) Conserved Domains search (https://www.ncbi.nlm.nih.gov/). The secondary structure of the protein was determined by Predictprotein tool (Germany) (https://www.predictprotein.org/), and the three-dimensional structure was predicted using Swissmodel (Swiss) (https://swissmodel.expasy.org/). Phylogenetic analysis was performed with MEGA 6.0 [Citation17].
Prokaryotic expression
The purified PCR products were ligated into to pMD18-T and transformed into E. coli DH5α competent cells. Positive clones were identified using PCR with primers RV-M and M13-47 (), and incubated in 30 mL of LB medium for 12 h, at 180 rpm and 37 °C. The plasmid was extracted using a plasmid mini kit I (Omega) and named pMD18-T-A-cbh. Plasmid pMD18-T-A-cbh was digested with BamH I and Hind III enzymes and ligated into vector pET-32a (Youbio, Changsha, China) that was digested with the same enzymes. Primers pET1 and pET2 () were used to screen positive clones, and the recombinant plasmid pET32a-A-cbh was recovered and transformed into E. coli BL21.
The transformed E. coli BL21 were cultured overnight in a rotary shaker incubator (180 rpm) at 37 °C in LB medium with a final concentration of 50 μg/mL of ampicillin. The overnight culture was inoculated into fresh LB medium at a ratio of 1:100, and then cultured under the same conditions to the exponential growth stage (optical density at 600 nm (OD600) of 0.5–0.8). Then, 1 mL of bacterial culture was sampled as the negative control and a bacterial culture of E. coli transformed with empty pET32a was used as the blank control. Thereafter, isopropyl β-D-thiogalactoside (IPTG) at a final concentration of 1 mmol/L was added to the remaining culture, which was incubated at 28 °C. Samples were taken at intervals of 2 h until 10 h. The samples were centrifuged at 13770 × g for 10 min to remove the supernatant and the pellets were stored at −20 °C.
Protein buffer (4×; Solarbio, Beijing, China) was used to dissolve the thallus, followed by incubation in a boiling water bath for 10 min and centrifugation at 13770 × g for 5 min. Finally, the supernatant was used for SDS-PAGE analysis.
Analysis of A-cbh expression in A. heimuer
In this study, eight different samples were collected to detect the expression of A-cbh. Mycelia were activated on PD agar medium and then inoculated in a 500-mL conical flask filled with 200 mL of liquid medium (100 g potatoes/L, 40 g wheat bran/L, 15 g brown sugar/L, 10 g dextrose/L, 2 g KH2PO4/L, 2 g peptone/L, 1 g MgSO4/L, and 10 mg VB1/L). The inoculated conical flasks were placed in a rotary shaker incubator (180 r/min) at 25 °C. The mycelia were harvested at 6, 8, 10, and 12 d [Citation18], and stored at −80 °C until RNA extraction. The remaining mycelia were used to inoculate sawdust medium, which comprised 78% sawdust, 20% wheat bran, 1% bean flour, and 1% corn flour. Primordia (1–3 mm) and fruit bodies in the stages of ear buds (5–10 mm), the immature period (10–20 mm), and the mature period (over 20 mm) were collected (), frozen in liquid nitrogen, and stored at −80 °C until RNA extraction.
Figure 1. Fruiting bodies stage of A. heimuer, including primordia (a), ear buds (b), immature period (c), and maturity period (d).
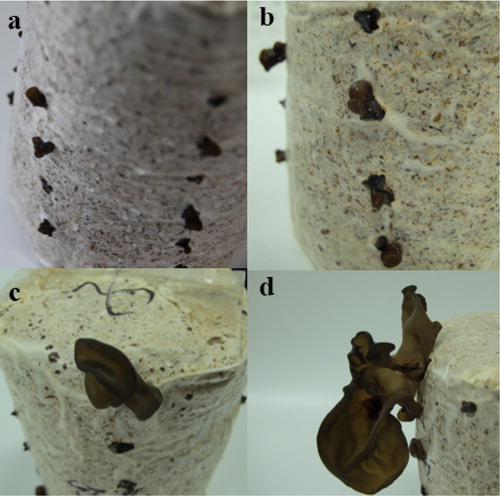
The process of total RNA extraction and evaluation of the quality and concentration of the RNA were performed as described previously. Afterwards, 1 μg of total RNA was reverse transcribed into cDNA using a PrimeScript™ RT reagent Kit (Takara), according to the manufacturer’s instructions. Finally, the cDNA was diluted 10-fold with deionized water and used as the template in a quantitative real-time PCR (qPCR) experiment. Primers A-cbh-qF and A-cbh-qR were used to detect the transcript levels of A-cbh, and β-actin gene was used as internal reference (). The reaction mixture for qPCR comprised 10 μL of 2 × TB Green Premix ExTaq II (Takara), 1 μL of cDNA template, 0.8 μL of primers, and 8.2 μL of deionized water. The qPCR reaction was carried out using a Mx3000P Sequence Detection System (Agilent Technologies, Santa Clara, CA, USA) under the following conditions: predegeneration at 95 °C for 10 min, followed by 35 cycles of denaturation at 95 °C for 30 s, annealing at 56 °C for 40 s, and extension at 72 °C for 40 s. The mycelia harvested at 6 d served as the reference sample (control), and the transcription levels of the A-cbh were evaluated using the 2−ΔΔCt method [Citation19].
Results and discussion
Cloning and amino acid sequence analysis of A-cbh
The A-cbh cDNA was obtained using RT-PCR, and the sequencing results showed that the cDNA sequence of A-cbh was 1551 bp, encoding a putative protein of 516 amino acids (). According to the amino acid sequence analysis (), the amino acid with the highest content in the protein was Gly (12.02%); the lowest content was His (0.97%). The molecular weight of the predicted protein was 54.44 kDa, and the theoretical isoelectric point was 5.24. The total number of negatively charged amino acids (Asp + Glu) was 45, whereas the total number of positively charged amino acids (Arg + Lys) was 37. The protein was predicted to be stable, with an instability index of 19.73, and the GRAVY value was −0.426, indicating a hydrophylic protein.
BlastP analysis indicated that the amino acid sequence of A-cbh contains putative conserved domains of GH family 7 and a cellulose-binding domain (). Scanning the transmembrane protein topology using the TMHMM tool revealed that all 516 amino acids of the protein would be located outside of cell membranes (), i.e. there was no predicted transmembrane region in the A-cbh protein. The secondary structure of the predicted protease consists of 10.85% alpha helix, 21.12% beta sheet, and 68.02% loop (coil), as predicted by PredictProtein. The three-dimensional structure of A-cbh was predicted using Swissmodel and the results are shown in , which was built using 1,4-beta-cellobiosidase (SMTL id: 2xsp.1.A) as the template.
As an important component of the cellulase system, the biochemical properties of A-cbh such as stability, optimal temperature, and pH will be investigated in a future study.
Phylogenetic analysis
Using the A-cbh sequence as a query, 16 homologous sequences () were selected from the GenBank database using the BLAST algorithm at NCBI. The evolutionary tree was constructed using MEGA 6.0 (). The phylogenetic analysis showed that A-cbh clustered with cellulases from other fungi, and A-cbh was most similar to cbh from Auricularia subglabra, which is from the same genus. However, there was a large phylogenetic distance to Paenibacillus terrae, which was used as an external reference.
Table 2. Homologous sequences of A-cbh protein.
Prokaryotic expression of A-cbh
The recombinant plasmid pET32a-A-cbh was successfully constructed, and then plasmids pET32a and pET32a-A-cbh were transferred into E. coli BL21 cells separately. After culture, samples were collected and gel electrophoresis was performed. Coomassie brilliant blue staining revealed a clear protein band with a molecular weight of approximately 74.44 kDa in the 10% SDS-PAGE gel (). Considering that pET32a expresses recombinant proteins with a 20 kDa peptide tag, the results indicated that A-cbh was successfully expressed in E. coli BL21.
Figure 8. Heterologous protein expression in E. coli after IPTG (1 mmol/L) induction. M: protein molecular weight marker (Tiangen, Beijing, China); Lane 1: E. coli transformed with the empty vector; Lane 2: E. coli transformed with pET32a-A-cbh induced with IPTG at 0 h; Lane 3: E. coli transformed with pET32a-A-cbh induced with IPTG at 2 h; Lane 4: E. coli transformed with pET32a-A-cbh induced with IPTG at 4 h; Lane 5: E. coli transformed with pET32a-A-cbh induced with IPTG at 6 h; Lane 6: E. coli transformed with pET32a-A-cbh induced with IPTG at 8 h; Lane 7: E. coli transformed with pET32a-A-cbh induced with IPTG at 10 h.
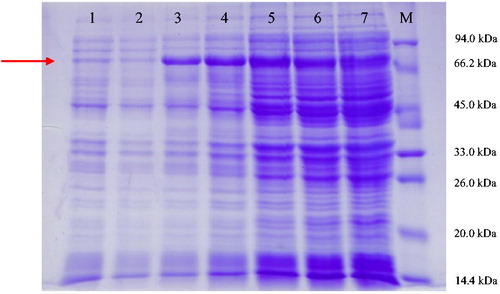
Vector pET-32a is a powerful tool for producing recombinant proteins in E. coli, which features a T7 lac promoter, 6 × His-tag and thioredoxin [Citation20]. Thioredoxin is included in the fusion protein not only to reduce digestion by bacterial proteases, but also to promote the expression of the recombinant fusion protein [Citation21]. Temperature is the main factor for the formation of inclusion body proteins, and E. coli reproduces rapidly at 37 °C, at which temperature proteins in the bacteria are synthesized too quickly to have enough time to fold; disulphide bonds cannot be properly coupled, and too many non-specific bonds between proteins are formed. Therefore, at the beginning of IPTG induction, the temperature was lowered to 28 °C to increase the expression of the correctly folded target protein.
Transcription levels of A-cbh in different stages
Quantitative PCR was used to detect the transcription of A-cbh at different growth stages of A. heimuer. The results () indicated that the transcription levels of A-cbh in the mycelium stage were lower than those in other stages, and the highest expression was observed at the primordium stage, which was 8.65-fold higher than that in the control (mycelium at 6 days). The transcription levels decreased during the ear buds period and then gradually increased to 7.87 times of control. Therefore, we speculated that the A-cbh protein plays a role in the formation of primordia and the development of fruiting bodies. A previous study showed that cellulase activity is closely related to the development of fruiting bodies in Agaricus bisporus [Citation22], in which the cellulase activity correlated positively with fruiting body yield. In addition, the β-glucosidase gene in Volvariella volvacea also has a regulatory effect on fruiting body development [Citation23]. In A. heimuer, the effect of the transcription levels of A-cbh on the fruiting entity yield remains to be further studied.
A. heimuer, the fourth most important cultivated mushroom worldwide, has been used by humans for many years [Citation24]. The functions of the active ingredients of A. heimuer, such as polysaccharides and melanin, have been studied intensively. However, less research has been done at the molecular level. The available genomic resources for A. heimuer have been of great help in research into A. heimuer’s wood degradation ability, secondary metabolite production capability, and biosynthesis of polysaccharides [Citation25].
Conclusions
In the present study, a cellobiohydrolase gene, A-cbh, was cloned and successfully expressed in E. coli. This protein contains the conserved structure of the glycoside hydrolase 7 family, members of which play an important role in the degradation of cellulose. The expression of A-cbh might promote the formation of primordium and the development of fruiting bodies. The results of our research provide valuable information for further functional studies of A-cbh, which may have potential value in breeding high-yielding strains of A. heimuer.
Acknowledgment
The authors are grateful to Professor Li Zou (Department of Forest Protection, University of Northeast Forestry, Harbin, China) for her guidance and help.
Disclosure statement
All the authors have no conflicts of interest.
Additional information
Funding
References
- Muszyńska B, Grzywacz-Kisielewska A, Kała K. Anti-inflammatory properties of edible mushrooms: a review. Food Chem. 2018;243:373–381.
- Wu F, Dai YC. Notes on the nomenclature of the Auricularia auricular-judae complex. Mycosystema (Chinese). 2015;34:604–611.
- Li L, Zhong CH, Bian YB. The molecular diversity analysis of Auricularia auricula-judae in China by nuclear ribosomal DNA intergenic spacer. Electron J Biotechn. 2014;17(1):27–33.
- Yang LQ, Zhao T, Wei H, et al. Carboxymethylation of polysaccharides from Auricularia auricula and their antioxidant activities in vitro. Int J Biol Macromol. 2011;49(5):1124–1130.
- Reza MA, Hossain MA, Lee SJ, et al. Dichlormethane extract of the jelly ear mushroom Auricularia auricula-judae (Higher Basidiomycetes) inhibits tumor cell growth in vitro. Int J Med Mushr. 2014;16(1):37–47.
- Khaskheli SG, Zheng W, Sheikh SA, et al. Characterization of Auricularia auricula polysaccharides and its antioxidant properties in fresh and pickled product. Int J Biol Macromol. 2015;81:387–395.
- Yuan Z, He P, Cui J, et al. Hypoglycemic effect of water-soluble polysaccharide from Auricularia auricula-judae Quel on genetically diabetic KK-A Y mice. Biosci Biotech Bioch. 1998;62(10):1898–1903.
- Luo YC, Chen G, Li B, et al. Evaluation of antioxidative and hypolipidemic properties of a novel functional diet formulation of Auricularia auricula and Hawthorn. Innov Food Sci Emerg. 2009;10(2):215–221.
- Yoon SJ, Yu MA, Pyun YR, et al. The nontoxic mushroom Auricularia auricula contains a polysaccharide with anticoagulant activity mediated by antithrombin. Thromb Res. 2003;112(3):151–158.
- Burchard W, Schulz L. Functionality of the β (1, 4) glycosidic linkage in polysaccharides. Macromol Symp.. 1995;99(1):57–69.
- Igarashi K, Uchihashi T, Koivula A, et al. Traffic jams reduce hydrolytic efficiency of cellulase on cellulose surface. Science. 2011;333(6047):1279–1282.
- Lynd LR, Weimer PJ, Zyl WHV, et al. Microbial cellulose utilization: fundamentals and biotechnology. Microbiol Mol Biol R. 2002;66(3):506–577.
- Lindenmuth BE, Mcdonald KA. Production and characterization of Acidothermus cellulolyticus endoglucanase in Pichia pastoris. Protein Expres Purif. 2011;77(2):153–158.
- Zoglowek M, Lubeck PS, Ahring BK, et al. Heterologous expression of cellobiohydrolases in filamentous fungi-An update on the current challenges, achievements and perspectives. Process Biochem. 2015;50(2):211–220.
- Teeri TT. Crystalline cellulose degradation: new insight into the function of cellobiohydrolases. Trends Biotechnol. 1997;15(5):160–167.
- Payne CM, Knott BC, Mayes HB, et al. Fungal cellulases. Chem Rev. 2015;115(3):1308–1448.
- Sanmukhiya VMR, Chellan Y, Soulange JG. Biochemical and phylogenetic analysis of Eugenia and Syzygium species from Mauritius. J Appl Res Med Aroma. 2019;12:21–29.
- Zou L, Sun TT, Yang YY, et al. Molecular characterization of a glycoside hydrolase family-51 α-L-arabinofuranosidase from Auricularia auricular. Biotechnol Biotecnol Equip. 2017;31(3):469–476.
- Yuan JS, Reed A, Chen F, et al. Statistical analysis of real-time PCR data. BMC Bioinformatics. 2006;7:85–96.
- Chang H, Cheng A, Wang M, et al. Cloning, expression and characterization of gE protein of duck plague virus. Virol J. 2010;7(1):120–130.
- Tatsuda D, Arimura H, Tokunaga H, et al. Expression and purification of cytokine receptor homology domain of human granulocyte-colony-stimulating factor receptor fusion protein in Escherichia coli. Protein Expres Purif. 2001;21(1):87–91.
- Claydon N, Allan M, Wood DA. Fruit body biomass regulated production of extracellular endocellulase during periodic fruiting by Agaricus bisporus. T Brit Mycol Soc. 1988;90(1):85–90.
- Ding S, Ge W, Buswell JA. Molecular cloning and transcriptional expression analysis of an intracellular beta-glucosidase, a family 3 glycosyl hydrolase, from the edible straw mushroom, Volvariella volvacea. Fems Microbiol Lett. 2007;267(2):221–229.
- Yan PS, Luo XC, Zhou Q. RAPD molecular differentiation of the cultivated strains of the jelly mushrooms, Auricularia auricula and A. polytricha. World J Microbiol Biotechnol. 2004;20(8):795–799.
- Yuan Y, Wu F, Si J, et al. Whole genome sequence of, Auricularia heimuer, (Basidiomycota, Fungi), the third most important cultivated mushroom worldwide. Genomics. 2019;111(1):50–58.