Abstract
Shiraia bambusicola, as a well-known medicinal and edible macro-fungus, is generally located on the branches of bamboo and plays an important role in herbal treatment. Furthermore, the natural products from its fruiting bodies, hypocrellins, are attracting increasing amounts of attention due to their notable bioactivities. In this report, we focused on constructing a fast and universal transgenic system for S. bambusicola, which could easily regulate the biosynthesis of hypocrellins by altering gene expression. By the consideration of the broadened application of the mycelial transformation, it was determined to implement the Agrobacterium tumefaciens-mediated transformation (ATMT) technique, and a transcription factor (SbTF1) was employed to activate the downstream genes in different producing strains. After real-time polymerase chain reaction analysis, gene SbTF1 was verified to be overexpressed in all of the transgenic strains, and the yield of hypocrellins was significantly improved in strain CNU103846. To confirm the regulated function, the transcript levels of the downstream genes and the corresponding products at different stages were screened. In this study, we developed a stable and easily available assay for genetic transformation of S. bambusicola and employed the method to demonstrate that one transcription factor SbTF1 could effectively increase the yield of the final products.
Introduction
Shiraia bambusicola P. Henn. is hosted on the branches of bamboos and is widespread over the southern region of China. As a traditional herbal medicine, fruiting bodies of S. bambusicola have been used to treat rheumatic arthritis, pertussis, tracheitis and skin diseases for several centuries. Hypocrellins, including hypocrellin A (HA), hypocrellin B (HB), hypocrellin C (HC) and hypocrellin D (HD), are the dominant secondary metabolites in S. bambusicola [Citation1, Citation2]. Currently, these components have emerged as notably potent novel therapeutic medicine, especially in photodynamic therapy (PDT), with antifungal, antibacterial, antiviral and antitumor activities [Citation3–6]. However, the low yield of produced hypocrellins has become a considerable bottleneck for further improvement.
Therefore, when considering hypocrellin production, the construction of an effective transgenic assay and the improvement of the fermentation yield by regulating gene expression are attractive. For the Shiraia species, protoplasts derived from conidia have been used for transformation, which yielded transgenic lines with stable inheritance [Citation7]. Unfortunately, most of the industrial strains could not effectively produce the corresponding spores, and furthermore, the higher-yield stains usually had weaker sporulation. Among the generally available methods, the Agrobacterium tumefaciens-mediated transformation (ATMT) technique is an easy and flexible transgenic assay and has been previously used in many plants, from the leaves of Arabidopsis thaliana to the callus of Zea mays (maize). Several species of Fusarium and other fungi have also smoothly produced transgenic fragmented mycelia by the same technique [Citation8–10].
In fungi, the functional genes are always clustered into neighboring locations and produce the metabolites in an integrated fashion. In certain instances, a specific transcription factor hidden in the gene cluster can directly regulate the other corresponding genes. AcstuA was identified from Acremonium chrysogenum as a transcriptional regulatory gene, and its disruption drastically reduced the cephalosporin production and blocked the expression of downstream genes [Citation11]. The transcription factor GaaR is needed for the expression of the genes required for D-galacturonic acid biosynthesis in Aspergillus niger, and the overexpression of GaaR resulted in an increased transcription of the D-galacturonic acid transporter [Citation12]. The putative gene cluster for the synthesis of hypocrellins was identified from the S. bambusicola genome [Citation13], and one putative transcription factor also appeared among them.
In this study, we aimed to optimize the genetic transformation of fragmented mycelia from S. bambusicola using ATMT assay, and furthermore, to make use of one specific transcription factor to increase the yield of hypocrellins.
Materials and methods
Organisms and plasmids
The original strains of S. bambusicola were preserved in the China Forestry Culture Collection Center (CFCC), including zzz816, GZ4-6, CNU103846 and B18. The strains were used as the wild-type recipients in the transformation experiments. Before transformation, these strains were incubated in PDB (potato dextrose broth) for two days. Agrobacterium tumefaciens strain AGL-1 (provided by Gang Liu, Institute of Microbiology, Chinese Academy of Sciences) was stored at −80 °C and revitalized in YEB (yeast extract beef) medium containing kanamycin before use. Escherichia coli DH5α (Mei5 Biotechnology Co., Ltd) was used in vector construction. A binary vector was constructed on the backbone of pAg1-H3 [Citation14] (provided by Gang Liu, Institute of Microbiology, Chinese Academy of Sciences), which harbors a hygromycin B resistance gene as a selection marker under the control of the fungal promoter PtrpC and terminator TtrpC. A 1.3-kb fragment of the transcription factor SbTF1 sequence, which was amplified from the genome of S. bambusicola, was incorporated into the plasmid driven by the PgpdA promoter. pAg1-OE was linearized by endonucleases, and the SbTF1 sequence was subsequently ligated to the linear vector using a ClonExpressTM II One Step Cloning Kit (Vazyme Biotech Co., Ltd.). The structures of pAg1-OE and pAg1-TF1 are shown in , and the primers used in this investigation appear in .
Figure 1. Construction of the binary vectors pAg1-OE (a) and pAg1-TF1 (b).
Note: The plasmids were constructed for the ATMT of Shiraia bambusicola with the SbTF1 gene placed under the strong promoter PgpdA from Aspergillus nidulans and the terminator TtrpC.
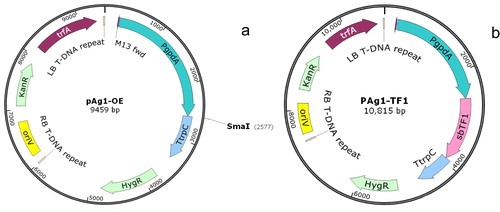
Table 1. Primers used in this investigation.
Fungal transformation
S. bambusicola (inoculated with a 2-day-old culture) were grown in 150 mL PDB under shake-culture conditions at 26 °C and 180 rpm for 4 days. The new culture was filtered through sterilized gauze to gather small mycelial pellets. Next, the mycelial pellets were gently washed with 20 mL of 0.5 mol/L MgSO4 and 0.05 mol/L maleic acid (pH 5.9) [Citation10]. The pellets were later milled with quartz sand in sorbitol solution, and the small mycelium, suspended on the face of the liquid, was picked up to soak on the filter for the transformation operation. A. tumefaciens strain AGL-1 was grown in minimal medium (MM) supplemented with kanamycin (100 μg/mL) at 28 °C for 2 days and was diluted to optical density at 600 nm (OD600) of 0.2 in induction medium [Citation15] in the presence of 200 μmol/L acetosyringone. The cells were incubated for an additional 6–8 h in a shaker at 28 °C until reaching OD600 = 0.6. Then MMP (minimal mycelial pellets) and bacteria were co-cultured at 24 °C for 2 h. The co-culture was dried out on filter paper and transferred to induction medium (200 μmol/L acetosyringone) and incubated for 48 h at 26 °C. After co-cultivation, the mycelial pellets were washed by cephalosporin at 100 μg/mL, transferred to PDA containing hygromycin (300 μg/mL) and cephalosporin (100 μg/mL), and subsequently incubated at 26 °C for 4 days to select the correct mutants. We randomly identified mycelia that were growing on the selective medium by polymerase chain reaction (PCR). Transformants were grown on PDA medium with hygromycin B together with the wild type as the control. In the sensitivity analysis the following were employed in selection media for ATMT: hygromycin B with different concentrations (0, 100, 150, 200, 250 and 300 μg/mL), benomyl (0, 100, 150, 200, 250 and 300 μg/mL), nourseothricin (0, 100, 150, 200, 250 and 300 μg/mL), glufosinate (0, 100, 150, 200, 250 and 300 μg/mL).
RNA isolation and RTFQ-PCR
RNA was isolated from the wild type strain and transformants, which were cultured in PDB medium without hygromycin at 26 °C for 96 h and 144 h, respectively, by M5 Plant RNeasy Mini Kit (Mei5 Biotechnology Co., Ltd). PrimeScriptTM RT reagent Kit with gDNA Eraser (Takara Biomedical Technology Co., Ltd.) was used to synthesise first-strand cDNA. To analyze the relative gene expression, real-time fluorescence quantitative PCR (RTFQ-PCR) was performed using a LightCycler 480II/96 (F. Hoffmann-La Roche Ltd). The primers used for RTFQ-PCR are shown in , including those for PKSI, SbTF1 and SbSAS1. We studied the reference gene selection and the relative expression from S. bambusicola CNU103846. SbSAS1 was identified as the most stable gene among the candidate reference genes [Citation16], and therefore, it was chosen as the reference gene to normalize the data for the analysis of the relative gene expression. The relative expression level of each gene was calculated using the 2-ΔΔCT method.
High performance liquid chromatography (HPLC)
Extraction of cell-associated hypocrellins from S. bambusicola was done as described by Shen et al. [Citation17]. The content of the extracts was analyzed by an Agilent 1200 Series HPLC system equipped with a Kromasil 100-5 C18 (250 × 4.6 mm). The content of elsinochrome was also detected by the same technique.
Data analysis
Data are presented as mean values with standard deviation (±SD) from three independent measurements.
Results and discussion
Sensitivity analysis for the chosen selection agents and concentrations
Four common selection agents, benomyl, nourseothricin, glufosinate and hygromycin B, were applied for the sensitivity analysis of S. bambusicola 816. In view of the popularity of different industrial strains, we decided to focus on the fragmented mycelia for transformation, and therefore, the results were dependent on the diameter of the colonies. As shown in , hygromycin B (300 μg/mL) and nourseothricin (200 μg/mL) displayed high bioactivity against the growth of mycelial strains; the others were incompatible with this species.
Table 2. Diameter of colonies incubated in increasing concentrations of hygromycin and nourseothricin.
Mycelial transformation by Agrobacterium tumefaciens and protoplasts
With the rapid improvement in the hypocrellins industry, more and more attention has been paid to molecular modification of the relevant strains. There have been studies for improvement of the genetic transformation techniques in recent years, mainly depending on protoplast transformation from spores [Citation18–21]. It is common for filamentous fungi to make use of spores as methods of reproduction, but this technique is not ideal to produce diverse strains for producing hypocrellins, especially since the higher yield strains have weaker sporulation. To remove this obstacle, we focused on the transformation of fragmented mycelia and even abandoned the optimized protoplast system by polyethylene glycol-mediation. The more convenient and robust ATMT tool was selected for further study, and this transformation system was able to guide the genes into the diverged strains. Breaking away from the limit of weak sporulation, almost all of the culturable strains from S. bambusicola successfully accepted the molecular modification, and similar species could also be modified with this reliable tool for genetic engineering.
Both the protoplasts and ATMT mediation were directly collected from the fragmented mycelia. An orthogonal array design (OAD) was employed for the optimization of protoplast separation. The maximum yield (production rate of protoplast 3.61 × 107/mL) was found at 18 h after treatment with 5 mg/L lallzyme + 4 mg/L Snail enzymes at 30 °C for 2.5 h and 50 rpm/min. After the PEG-CaCl2 mediated transformation, we achieved the production of transgenic strains and obtained marker genes through PCR analysis. By using the ATMT assay, the transgenic strains were also obtained, and the marker genes were easily confirmed. Between the PEG-CaCl2-mediated transformation and ATMT, the transformation efficiency varied from 5% to around 20%. ATMT’s versatility prevailed, and this approach was singled out for further development. Genomic DNA of both transformants and the wild type were extracted using the CTAB method. The hph gene was identified by PCR using primers Hyg500F/Hyg500R, which amplified a 500-kb fragment of the internal region of the hph gene.
Cloning and characterization of the putative transcription factor SbTF1 in S. bambusicola
The specific transcript factor SbTF1 was identified through homology searches with the BLASTP algorithm and it was located on the putative gene cluster for the hypocrellins synthesis pathway. The factor had a close relationship with the transcriptional activator of the sterigmatocystin biosynthesis gene cluster in Emericella nidulans and the cercosporin biosynthesis gene cluster in Cercospora nicotianae. RT-PCR analysis confirmed that the expression of SbTF1 was up-regulated during the fermentation stage but not very strongly or for long.
Analysis of different transformants using SbTF1 activation
Through the ATMT assay, the full length of the SbTF1 gene was added into plasmid vector pAg1-OE for high-efficiency expression and was transformed into different strains of S. bambusicola including CNU103846, zzz816, B18 and gangzhu4-6. Under routine testing, these transformations were finished successfully, and the transcript levels of SbTF1 were improved considerably. By the analysis of phenotype and HPLC results, the yield of hypocrellins was clearly boosted in CNU103846 (), but not in the other species (data not shown).
Regulation of SbTF1 in hypocrellin and elsinochrome production
The relevant functional genes, covering, mono and hyd, have been employed to improve the yield of hypocrelins [Citation19], and some amylase genes could also assist to produce the natural products [Citation20, Citation21]. The cluster-specific transcription factor, which usually appears in the biosynthetic gene cluster for natural products and could directly regulate the expression of the adjacent genes, was not involved [Citation22]. Among the similar perylenequinoid compounds, EfPKS1 and CTB1 have been respectively found in the gene cluster for elsinochrome and cercosporin, and gene knockout demonstrated their regulatory effects [Citation23–25]. However, none of them was reported to increase the yield of the relevant pigments.
In this study, we explored the putative transcription factor SbTF1 for hypocrellins and uniquely employed it to enhance perylenequinone production for the first time. By a trial of various strains with different productivity, we observed that the modified strain CNU103846 had made great progress in the synthesis of hypocrellins, considerably higher than the former strains, either the original CNU103846 or the high yield zzz816 strains. The yield of elsinochrome also displayed a similar variation trend but not so clearly. Even among the present studies with diverse modified strains, such as S. bambusicola zzz816 and Shiraia sp. Slf14, the strain CNU103846 was still at the top of the list [Citation17, Citation26]. It was noticeable that the premature overexpression of SbTF1 hindered the normal growth of mycelia; therefore, the final mass was significantly lower than in the primary strain. In the next step, we plan to improve the induction of the promoter and further re-optimize the process of submerged fermentation.
In the transgenic strain CNU103846, the period of hypocrellin production was shortened, which was consistent with the alteration of SbTF1. At a prior stage of submerged fermentation, the output per unit of hypocrellins had reached its peak, and it remained steady during the process. Although the early overexpression of SbTF1 played a negative role in mycelial growth, it is still worthy to note that the final yield of hypocrellins increased to 210.65% of the original strain and even exceeded that of the high yield strain zzz816 ().
Moreover, the key downstream gene SbPKSI was also analyzed by RTFQ-PCR, and its abnormal overexpression demonstrated that SbTF1 had up-regulated the putative gene cluster for hypocrellins synthesis. On the basis of the elevated production efficiency (), the results from also supported the close association of the gene cluster with production of hypocrellins.
Figure 3. Relative expression levels of SbTF1 and SbPKSI by real-time PCR.
Note: Each sample was detected after 96 h and 144 h of incubation.
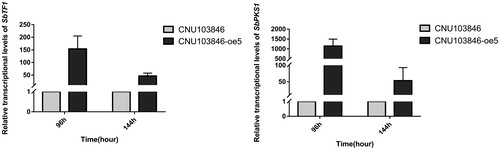
During submerged fermentation, the industrial strains were not always able to maintain the high-yield of hypocrellins, where the culture conditions and media components both might interfere with the process directly. In the general, the PDA medium is adapted to the S. bambusicola stains, but some important strains only produce hypocrellins in addition to Triton X-100 [Citation27,Citation28]. The employment of other media would receive more restrictions and would often eliminate the red pigments, especially including the natural components [Citation17, Citation29, Citation30]. The wave length and intensity of light could also determine the biosynthetic efficiency, so the increase of the production has to be limited to the update of equipment [Citation31–33]. These requirements are very likely to increase the cost of large-scale production and hamper the further development of the corresponding industries. In our study, the overexpression of SbTF1 was able to activate the relevant gene cluster and ensure the stability of hypocrellin production under the more compatible conditions. Thereof, the edited strains in this study were anticipated to have the great adaptability of various cultural conditions, and we would greatly advance the production of hypocrellins through the broader range of medium components and the more permissive way for fermentation.
Conclusions
In this study, the ATMT technique was employed to build a stable and easily available technique for genetic transformation of S. bambusicola, which could be used in the mycelial transformation for industrial strains. By this method, the cluster-specific transcription factor SbTF1 was overexpressed, and it improved the yield of the hypocrellins, up to 210.65% of the original strain. The stable performance of the fermentation process indicated that the modified strain would have an extensive application in the corresponding industry.
Disclosure statement
The authors declare that the research was conducted in the absence of any commercial or financial relationships that could be construed as a potential conflict of interest.
Additional information
Funding
References
- Fang LZ, Qing C, Shao HJ, et al. Hypocrellin D, a cytotoxic fungal pigment from fruiting bodies of the ascomycete Shiraia bambusicola. J Antibiot. 2006;59(6):351–354.
- Kishi T, Tahara S, Taniguchi N, et al. New perylenequinones from Shiraia bambusicola. Planta Med. 1991;57(4):376–379.
- Deininger MH, Weinschenk T, Morgalla MH, et al. Release of regulators of angiogenesis following Hypocrellin-A and -B photodynamic therapy of human brain tumor cells. Biochem Biophys Res Commun. 2002;298(4):520–530.
- Ma G, Khan SI, Jacob MR, et al. Antimicrobial and antileishmanial activities of hypocrellins A and B. Antimicrob Agents Ch. 2004;48(11):4450–4452.
- Su Y, Sun J, Rao S, et al. Photodynamic antimicrobial activity of hypocrellin A. J Photochem Photobiol B, Biol. 2011;103(1):29–34.
- Zhang S, Qiu D, Liu J, et al. Active components of fungus Shiraia bambusiscola can specifically induce BGC823 gastric cancer cell apoptosis. Cell J. 2016;18(2):149–158.
- Deng HX, Gao RJ, Chen JJ, et al. An efficient polyethylene glycol-mediated transformation system of lentiviral vector inShiraia bambusicola. Process Biochem. 2016;51(10):1357–1362.
- Combier JP, Melayah D, Raffier C, et al. Agrobacterium tumefaciens-mediated transformation as a tool for insertional mutagenesis in the symbiotic ectomycorrhizal fungus Hebeloma cylindrosporum. Fems Microbiol Lett. 2003;220(1):141–148.
- Pardo AG, Kemppainen M, Valdemoros D, et al. T-DNA transfer from Agrobacterium tumefaciens to the ectomycorrhizal fungus Pisolithus microcarpus. Rev Argent Microbiol. 2005;37(2):69–72.
- Sharma KK, Gupta S, Kuhad RC. Agrobacterium-mediated delivery of marker genes to Phanerochaete chrysosporium mycelial pellets: a model transformation system for white-rot fungi. Biotechnol Appl Biochem. 2016;43(3):181–186.
- Pengjie H, Ying W, Jun Z, et al. AcstuA, which encodes an APSES transcription regulator, is involved in conidiation, cephalosporin biosynthesis and cell wall integrity of Acremonium chrysogenum. Fungal Genet Biol. 2015;83:26–40.
- Alazi E, Knetsch T, Di Falco M, et al. Inducer-independent production of pectinases in Aspergillus niger by overexpression of the D-galacturonic acid-responsive transcription factor gaaR. Appl Microbiol Biotechnol. 2018;102(6):2723–2736.
- Zhao N, Lin X, Qi SS, et al. De Novo Transcriptome assembly in Shiraia bambusicola to investigate putative genes involved in the biosynthesis of Hypocrellin A. IJMS. 2016;17(3):311–325.
- Zhang A, Lu P, Dahl-Roshak AM, et al. Efficient disruption of a polyketide synthase gene (pks1) required for melanin synthesis through Agrobacterium-mediated transformation of Glarealozoyeasis. Mol Genet Genomics. 2003;268(5):645–655.
- Sørensen LQ, Lysøe E, Larsen JE, et al. Genetic transformation of Fusarium avenaceum by Agrobacterium tumefaciens mediated transformation and the development of a USER-Brick vector construction system. Bmc Mol Biol. (1) 2014;15:15–30.
- Zhang C, Li T, Hou CL, et al. Selection of reference genes from Shiraia bambusicola for RT-qPCR analysis under different culturing conditions. AMB Expr. 2017;7(1):14.
- Shen XY, Hu YJ, Song L, et al. Improvement of hypocrellin production by a new fungal source and optimization of cultivation conditions. Biotechnol Biotec. 2016;30(4):819–826.
- Deng H, Gao R, Liao X, et al. Genome editing in Shiraia bambusicola using CRISPR-Cas9 system. J Biotechnol. 2017;259:228–234.
- Li D, Zhao N, Guo BJ, et al. Gentic overexpression increases production of hypocrellin A in Shiraia bambusicola S4201. J Microbiol. 2019;57(2):154–162.
- Gao R, Deng H, Guan Z, et al. Enhanced hypocrellin production via coexpression of alpha-amylase and hemoglobin genes in Shiraia bambusicola. AMB Expr. 2018;8(1):71.
- Gao R, Xu Z, Deng H, et al. Enhanced hypocrellin production of Shiraia sp. SUPER-H168 by overexpression of alpha-amylase gene. PLoS One. 2018;13(5):e0196519. [cited 2019 Aug 18]; [22 p.].
- Keller NP. Fungal secondary metabolism: regulation, function and drug discovery. Nat Rev Microbiol. 2019;17(3):167–180.
- Hoffmeister D, Keller NP. Natural products of filamentous fungi: enzymes, genes, and their regulation. Nat Prod Rep. 2007;24(2):393–416.
- Newman AG, Townsend CA. Molecular characterization of the cercosporin biosynthetic pathway in the fungal plant pathogen Cercospora nicotianae. J Am Chem Soc. 2016;138(12):4219–4228.
- Yang SL, Chung KR. Transcriptional regulation of Elsinochrome phytotoxin biosynthesis by an EfSTE12 activator in the citrus scab pathogen Elsinoe fawcettii. Fungal Biol. 2010;114(1):64–73.
- Tong Z, Mao L, Liang H, et al. Simultaneous determination of six perylenequinones in Shiraiaia sp.Slf14 by HPLC. J Liq Chromatogr R T. 2017;40(10):536–540.
- Cai Y, Liao X, Liang X, et al. Induction of hypocrellin production by Triton X-100 under submerged fermentation with Shiraia sp. SUPER-H168. N Biotechnol. 2011;28(6):588–592.
- Lei XY, Zhang MY, Ma YJ, et al. Transcriptomic responses involved in enhanced production of hypocrellin A by addition of Triton X-100 in submerged cultures of Shiraia bambusicola. J Ind Microbiol Biotechnol. 2017;44(10):1415–1429.
- Liu B, Bao J, Zhang Z, et al. Enhanced production of perylenequinones in the endophytic fungus Shiraia sp. Slf14 by calcium/calmodulin signal transduction. Appl Microbiol Biotechnol. 2018;102(1):153–163.
- Ma YJ, Lu CS, Wang JW. Effects of 5-Azacytidine on growth and hypocrellin production of Shiraia bambusicola. Front Microbiol. 2018;9:2508. [cited 2019 Aug 18]; [14 p.]
- Gao R, Xu Z, Deng H, et al. Influences of light on growth, reproduction and hypocrellin production by Shiraia sp. SUPER-H168. Arch Microbiol. 2018;200(8):1217–1225.
- Ma YJ, Sun CX, Wang JW. Enhanced production of Hypocrellin A in submerged cultures of Shiraia bambusicola by red light. Photochem Photobiol. 2018;95(3):100–107.
- Sun CX, Ma YJ, Wang JW. Improved hypocrellin A production in Shiraia bambusicola by light-dark shift. J Photochem Photobiol B. 2018;182:100–107.