Abstract
The aim of this study was to investigate the effects of cyclophilin B on MC3T3-E1 cells and the underlying mechanism. CCK-8 assay was used to evaluate the cell activity, polymerase chain reaction to verify the overexpression of cyclophilin B, western blot to detect the protein levels, and clone formation assay to evaluate the proliferation ability. Alkaline phosphatase (ALP) activity was assessed by ALP assessment kit. The results showed that compared with the control group/negative control (NC) group, the cells in the cyclophilin B overexpression group possessed enhanced proliferation ability, higher ALP activity and enhanced mineralization. Compared with the control group, the levels of Oct4, Sox2, Runx2, collagen I, BMP2 and BMPR-II, as differentiation-related proteins, were increased by cyclophilin B. Compared to the NC group, the expression levels of p-JAK2 and p-STAT3 in the cyclophilin B overexpression group were higher, suggesting that cyclophilin B can activate the JAK2/STAT3 signaling pathway. The expression levels of p-JAK2 and p-STAT3 in the cyclophilin B overexpression group were higher than that in the cyclophilin B overexpression + JAK2 inhibitor group, further confirming the hypothesis that the JAK2/STAT3 signaling pathway was activated by cyclophilin B. Taken together, the results suggest that cyclophilin B promotes the proliferation and differentiation of MC3T3-E1 cells via the JAK2/STAT3 signaling pathway and may be a promising therapeutic target for bone injury.
Introduction
Bone diseases remain a significant problem and are also complications of many diseases including nephropathy, diabetes and cancer [Citation1–3]. To investigate the causes of bone diseases, it is beneficial to illuminate the mechanism of bone metabolism. Bone metabolism is a complicated process that is influenced by many factors such as gender, age, disease factors, minerals and so forth. In normal conditions, bone metabolism is at an equilibrium state of bone resorption and formation [Citation4]. Once the balance is broken, bone diseases caused by progressive bone loss occur.
Bone regeneration, as a promising avenue for treatment of bone diseases, has aroused widespread attention in recent years. Since most exogenous synthetic drugs have side effects for treatment of bone diseases, it is pressing to seek out the vital endogenous substances which participate in the proliferation and differentiation of osteocytes without negative consequences. Many studies have been conducted into the mechanisms of bone regeneration and homeostasis, including studies of DNA methylation, microRNA regulation and histone modifications [Citation5–8]. Additionally, many reports have focused on some key regulatory substances in osteogenic differentiation, such as proteins, lipopolysaccharides and other low-molecular-weight compounds [Citation9–11]. Bone morphogenetic proteins (BMPs) are reported to be the main inducing factor in bone repair, wherein the BMP2 subtype has better effects on inducing osteogenesis [Citation12]. BMP2 is reported to bind with type II receptor (BMPR-II) and phosphorylating type I receptor (BMPR-1). Then, BMPR-1 further phosphorylates Smads protein which is BMP2 specific. After that, Smads proteins enter the nucleus and promote release of alkaline phosphatase (ALP) by increasing the expression of the specific transcription factor (Runx2). Finally, the osteoblast differentiation is enhanced [Citation13, Citation14].
Cyclophilin B, as the prototypical member of the cyclophilin family, is one of the receptor proteins of intracellular cyclosporine A [Citation15]. Cyclophilin B mainly exists in the endoplasmic reticulum of all cell types and has been reported to be involved in many pathological and physiological processes, including hepatitis virus replication, immunosuppression, prolactin signaling and osteogenesis imperfecta [Citation16–19]. Cyclophilin B has peptidyl-prolyl cis-trans isomerase enzymatic activity, accelerating protein folding, and proper protein folding is vital for normal physiological functions of cells. Consistent with this opinion, studies of osteogenesis imperfecta have shown that cyclophilin B plays a critical role in facilitating proper collagen formation and bone formation [Citation20]. Type I collagen post-translational modification is reported to be abnormal in cyclophilin B knock-out mice of recessive osteogenesis imperfecta [Citation21]. Mutations in the cyclophilin B encoding gene are reported to cause osteogenesis imperfecta as well [Citation22].
As previous studies have suggested a series of vital functions of cyclophilin B in bone formation, in this study, we investigated the effects of cyclophilin B on the proliferation and differentiation of MC3T3-E1 cells and the underlying mechanism.
Materials and methods
Materials
Cell Counting Kit was obtained from Sigma-Aldrich (Merck, Darmstadt, Germany); fetal bovine serum (FBS) was purchased from Gibco BRL (Thermo Fisher Scientific, Waltham, MA). All the regents used here were of analytical grade. All antibodies were purchased from Abcam company (Cambridge, MA, USA).
Cell culture
MC3T3-E1 cells purchased from ATCC (Manassas VA)) were cultured in 96-well culture plates (1 × 104 cells/well), in α-MEM complete medium containing 10% fetal bovine serum, 100 μg/mL streptomycin, and 100 U/mL penicillin. MC3T3-E1 cells were cultured at 37 °C in a cell incubator with 5% CO2. The cells at 80% confluence were sub-cultured every 3 days. For clone formation assay, ALP activity detection, alizarin red assay, and verification of signaling pathway, the cells were cultured for 14 days.
Transient transfection of MC3T3-E1 cells with cyclophilin B plasmid
The cyclophilin B plasmid was constructed as described before [Citation23]. Empty vector, was added to the MC3T3-E1 cell culture dish. Cells transfected with the empty vector served as the control group, whereas non-transfected cells served as the negative control (NC) group. The transfection solution was added to another cell-culture dish. After transfection for 72 h, polymerase chain reaction (PCR) and western blot were applied to verify the cyclophilin B overexpression. PCR was performed as described in the literature [Citation23].
Cell viability
After transfection, 10 μL CCK-8 solution was added to each well of 96-well plates in the experimental group, control group and negative control (NC) group at 24 h, 48 h and 72 h. The cells were incubated at 37 °C in a cell incubator with 5% CO2 for 4 h. The absorbance at 490 nm was measured by a microplate reader.
Western blot
The cells in each group were lysed in sodium dodecyl sulfate (SDS) sample buffer (150 mmol/L NaCl, 50 mmol/L Tris-HCl (pH 7.0), and 0.1% Triton X-100). The lysates in each group were harvested by centrifugation at 10,000 g for 15 min. The protein expression levels were detected as described before [Citation24]. The total proteins were separated by sodium dodecyl sulfate-polyacrylamide gel electrophoresis (SDS-PAGE, 10%), and then the proteins were transferred to a polyvinylidene fluoride (PVDF) membrane, which then was blocked in Tween 20/TBS (TTBS) and incubated with the primary antibodies at 4 °C overnight, followed by secondary antibody for 1 h. The following primary antibodies were used: Anti-Cyclophilin B antibody (#ab16045), Anti-Oct4 antibody (#ab181557), Anti-RUNX2 antibody (#ab192256), Anti-SOX2 antibody (#ab97959), Anti-Collagen I antibody (#ab34710), Anti-BMP2 antibody (#ab14933), Anti-BMPR II antibody (#ab130206), Anti-P-JAK2 antibody (#ab32101), Anti-JAK2 antibody (#ab108596), Anti-p-STAT3 antibody (#ab76315) and Anti-STAT3 antibody (#ab119352). The secondary antibody was horseradish peroxidase-conjugated secondary antibodies (#ab205718). Glyceraldehyde 3-phosphate dehydrogenase (GAPDH) (ab181602) was used as the internal reference.
Clone formation assay
The monolayer cells in the logarithmic phase in each group were harvested by using 0.25% trypsin and were mechanically blown to obtain single-cell suspensions. Then, the cell suspension solution in each group was diluted and plated in the cell culture dish. Next, the cells were incubated in the incubator with 5% CO2. Then the supernatant of each culture dish was removed. After that, the cells in each group were washed with PBS, and 5 mL methyl alcohol was added. After 15 min, the cells were stained by Giemsa for 30 min, then washed and dried.
Alkaline phosphatase (ALP) activity detection
ALP assay kit (Abcam, Cambridge, UK) was used to determine the alkaline phosphatase activity according to the manufacturer’s instructions. Measurements were done three times, at 405 nm wavelength, by using a microplate reader, and the average value in each group was obtained as the final value.
Alizarin red staining assay (mineralization)
After fixation with 10% formalin, the cells of each group were washed, and then incubated with working solution of Alizarin Red S for 8 min. Then each sample was washed three times with distilled water. Inverted fluorescence microscopy (Zeiss Axiovert 100 M, Pleasanton, CA was applied to observe the calcium deposits.
Verification of signaling pathway
To determine the possible signaling pathway, 100 μm FM-A13 as the JAK2 inhibitor was added into the cyclophilin B overexpression group. Then the cells of all the groups were incubated for 24 h, and the expression levels of BMP2, BMPRII, p-JAK2, p-STAT, t-JAK2 and t- STAT3 were evaluated by western blot assay as described above.
Statistical analysis
Statistical analysis was performed with SPSS software (IBM Corps) and GraphPad prism 5.0. The data shown are expressed as mean values with standard deviation (±SD). Significant differences were determined by one-way or two-way analysis of variance with Dunnett’s test.
Results and discussion
Effects of cyclophilin B overexpression on the proliferation of MC3T3-E1 cells
The results showed that in the cyclophilin B overexpression group, the level of cyclophilin B was higher than that in the control group or NC group (. The PCR results in were in accord with those in , further demonstrating that the MC3T3-E1 cells with cyclophilin B overexpression were obtained successfully. Subsequently, the CCK-8 kit was used to evaluate the proliferation ability in each group. As shown in , the proliferation level increased in a time-dependent manner (24 h, 48 h and 72 h), and the growth of MC3T3-E1 cells in the cyclophilin B overexpression group increased more rapidly than that in the control group. The clone formation assay further confirmed the results above. In , the MC3T3-E1 cells in the cyclophilin B overexpression group possessed enhanced proliferation ability. Taken together, all the results suggested that cyclophilin B was able to promote the proliferation of MC3T3-E1 cells.
Figure 1. Cyclophilin B expression (a, b) and cell proliferation (c, d) in MC3T3-E1 cells with or without cyclophilin B overexpression. Cyclophilin B expression was evaluated by western blot (a) and cyclophilin B mRNA level (b); and cell proliferation was assayed by cell viability assay (c) and clone formation assay (d). Note: CypB, cyclophilin B; NC, negative control (non-transfected cells). ### P < 0.001 vs. NC; ***P < 0.001 vs. control.
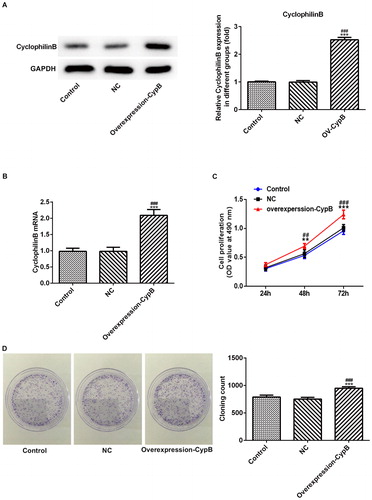
Effects of cyclophilin B overexpression on the differentiation of MC3T3-E1 cells
To evaluate the differentiation of MC3T3-E1 cells in different groups, we determined the alkaline phosphatase activity (ALP) as a differentiation marker. As shown in , the ALP activity was higher in the cyclophilin B overexpression group in comparison with the control group/NC group. The differences in the ALP activity between the cyclophilin B overexpression group and the control group/NC group were statistically significant. Matrix mineralization as the final step of osteoblastic differentiation was further investigated by Alizarin red S staining assay. As seen in , the cells in the cyclophilin B overexpression group had enhanced mineralization in comparison with the control group/NC group.
Effects of cyclophilin B overexpression on the expression levels of Oct4, Sox2, Runx2 and Collagen I
Oct4, Sox2, Runx2 and Collagen I are osteoblast differentiation markers [Citation25–27]. To further investigate the effects of cyclophilin B on the cell differentiation, we evaluated their expression levels by western blot assay. Oct4, Sox2, Runx2 and Collagen I were all up-regulated in the cyclophilin B overexpression group, in comparison with the control group (), indicating that enhanced cell differentiation was associated with cyclophilin B overexpression.
Effects of cyclophilin B on the expression levels of BMP2 and BMPR-II
BMP2 and BMPR-II, as important growth factors controlling osteoblast differentiation, were evaluated herein via western blot and immunofluorescence. Compared with the control group (), both BMP2 and BMPR-II were up-regulated in the cyclophilin B overexpression group, suggesting that cyclophilin B can promote MC3T3-E1 cells differentiation by up-regulating BMP2 and BMPR-II.
Cyclophilin B overexpression was associated with up-regulated expression of BMP2 and BMPR-II via the JAK2/STAT3 signaling pathway
Since JAK2/STAT3 signaling pathway is an important part of bone metabolism, the relationship between the JAK2/STAT3 signaling pathway and cyclophilin B were investigated herein. Compared with the NC group, the expression levels of BMP2, BMPR-II, p-JAK2 and p-STAT3 in the cyclophilin B overexpression group were increased (), whereas the total expression levels of JAK2 and STAT3 in the cyclophilin B overexpression group remained unchanged. In the cyclophilin B overexpression group with FM-A13 added, the expression levels of BMP2, BMPR-II, p-JAK2 and p-STAT3 were decreased, in contrast to the cyclophilin B overexpression group, indicating that JAK2/STAT3 signaling pathway was activated by cyclophilin B. All the results indicated that the proliferation and differentiation of MC3T3-E1 cells was enhanced by cyclophilin B via the JAK2/STAT3 signaling pathway.
Figure 4. Expression levels of proteins from the JAK2/STAT3 signaling pathway, evaluated by western blot, in MC3T3-E1 cells with or without cyclophilin B overexpression and FM-A13 JAK2 inhibitor. Note: CypB, cyclophilin B; NC, negative control (non-transfected cells); OV-CypB, overexpressed cyclophilin B group; OV-CypB + FM-A13, overexpressed cyclophilin B and FM-A13 JAK2 inhibitor group. *** P < 0.001, ** P < 0.01 and *P < 0.05 vs. NC.
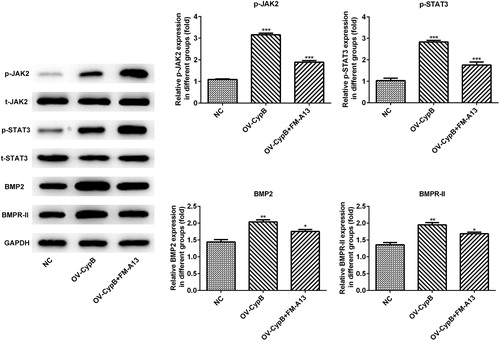
These results are in agreement with current knowledge that cyclophilin B is an important protein in a range of physiological processes including cancer, virus replication, HIV-1 infection, and proximal tubular cell injury [Citation23, Citation28–30]. However, the effects of cyclophilin B on MC3T3-E1 cells were not investigated before. Cyclophilin B was reported to increase with bergenin treatment, resulting in enhanced osteogenic differentiation, but an in-depth study on the effect of cyclophilin B in osteogenic cells was not performed [Citation31]. Based on that, we conducted the in-depth study on the effects of cyclophilin B in MC3T3-E1 cells.
Imbalance of bone resorption and bone formation contributes to net bone loss or osteoporosis. There are mainly two broad approaches for therapeutics of osteoporosis: osteoclast activity inhibition/bone loss suppression and promotion of bone formation. When bone loss has already occurred in patients with osteoporosis, promotion of bone formation may be a better choice for treatment of osteoporosis. MC3T3-E1 cells are fundamental components in the process of bone formation. Therefore, studies on the proliferation and differentiation of MC3T3-E1 cells, are extremely important for developing osteoporosis treatment strategies. In the present research, the proliferation of MC3T3-E1 cells was progressively enhanced by cyclophilin B overexpression at 24 h, 48 h and 72 h. ALP and collagen I served as early cell differentiation markers. ALP activity and collagen I were up-regulated in cells overexpressing cyclophilin B, indicating that cyclophilin B promoted the early differentiation of MC3T3-E1 cells. The levels of Oct4, Sox2 and Runx2, which are closely related to cell differentiation, were up-regulated in association with cyclophilin B overexpression, providing further evidence that the differentiation was likely enhanced by cyclophilin B. As shown by the results of the Alizarin red staining assay, mineralization was enhanced in cells overexpressing cyclophilin B, indicating that cyclophilin B promoted the differentiation of MC3T3-E1 cells in the late phase. Taken together, all the results discussed above provided evidence that cyclophilin B most likely promoted MC3T3-E1 cell differentiation by participating in the early and late cell differentiation process.
Cell differentiation is important for bone repair. Illumination of the mechanism of action of some endogenous substances in cell differentiation is crucial to bone regeneration therapy by facilitating the search for therapeutic targets and the development of corresponding therapeutic drugs. Cyclophilin B has been reported to increase collagen folding and increase MC3T3-E1 differentiation [Citation32]. JAK2/STAT3 as the common signaling pathway is reported to be activated in cell differentiation. IL-17A regulates osteoblast activity and differentiation by JAK2/STAT3 signaling pathway [Citation33]. By activating the JAK2/STAT3 signaling pathway, HIF-1α promoted the expression of RANKL, resulting in enhanced osteocyte-mediated osteoclastic differentiation [Citation34].
The role of JAK2/STAT3 signaling pathway in osteogenic differentiation in vitro, bone regeneration in vivo and bone defect healing were investigated in previous reports, wherein, the proliferation and osteogenic differentiation of bone marrow stromal cells were decreased by JAK2 inhibitor [Citation35]. Herein, we speculate that it was via the JAK2/STAT3 signaling pathway that cyclophilin B promoted the proliferation and differentiation of MC3T3-E1 cells. The data showed that compared with the NC group, the expression levels of p-JAK2, p-STAT3, BMP2 and BMPRII in the cyclophilin B overexpression were higher, suggesting that cyclophilin B enhanced the levels of BMP2 and BMPRII by activating the JAK2/STAT3 signaling pathway. The expression levels of p-JAK2, p-STAT3, BMP2 and BMPRII in the cyclophilin B overexpression group treated with JAK2 inhibitor were decreased in comparation to the cyclophilin B overexpression group. Furthermore, the total expression levels of JAK2 and STAT3 among all the groups remained unchanged. All these results indicated that the proliferation and differentiation of MC3T3-E1 cells were most likely enhanced by cyclophilin B through the JAK2/STAT3 signaling pathway. Noteworthily, the JAK2/STAT3 signaling pathway is not the only signaling pathway that has been suggested to be involved in osteoblast differentiation. Other findings have suggested that the Erk-Runx2 and BMP-Smad pathways are more important and classical in osteoblast differentiation [Citation36, Citation37]. There may exist cross-talk among some signaling pathways in osteoblast differentiation. Thus, it would be interesting to investigate the potential interactions between these alternative mechanisms in future studies.
Conclusions
The obtained results showed that cyclophilin B most likely promoted the proliferation and differentiation of MC3T3-E1 cells via the JAK2/STAT3 signaling pathway and is a potential therapeutic target for bone healing. The findings in this study shed light on the mechanism of osteoblast differentiation and provide a rational theory for further research.
Acknowledgements
The authors thank Shen Liya (the Sixth Hospital of Wuhan Affiliated Hospital of Jianghan University) for useful suggestions provided.
Disclosure statement
No potential conflict of interest was reported by the authors.
References
- Shanbhogue VV, Hansen S, Frost M, et al. Bone disease in diabetes: another manifestation of microvascular disease? Lancet Diabetes Endocrinol. 2017;5(10):827–838.
- Yee AJ, Raje NS. Denosumab for the treatment of bone disease in solid tumors and multiple myeloma. Future Oncol. 2018;14(3):195–203.
- Hasegawa K, Motoyama O, Shishido S, et al. Mineral disorders in pediatric pre-emptive kidney transplantation. Pediatr Int. 2019;61(6):587–594.
- Chen Y-S, Guo Q, Guo L-J, et al. GDF8 inhibits bone formation and promotes bone resorption in mice. Clin Exp Pharmacol Physiol. 2017;44(4):500–508.
- Liu Y, Zhang X-L, Chen L, et al. Epigenetic mechanisms of bone regeneration and homeostasis. Prog Biophys Mol Biol. 2016;122(2):85–92.
- de Andrés MC, Kingham E, Imagawa K, et al. Epigenetic regulation during fetal femur development: DNA methylation matters. PLoS One. 2013;8(1):e54957.
- Ye L, Fan Z, Yu B, et al. Histone Demethylases KDM4B and KDM6B Promotes Osteogenic Differentiation of Human MSCs. Cell Stem Cell. 2012;11(1):50–61.
- Zhang Y, Xie R-L, Croce CM, et al. A program of microRNAs controls osteogenic lineage progression by targeting transcription factor Runx2. Proc Natl Acad Sci U S A. 2011;108(24):9863–9868.
- Ma JY, Wong KL, Xu ZY, et al. N16, a nacreous protein, inhibits osteoclast differentiation and enhances osteogenesis. J Nat Prod. 2016;79(1):204–212.
- Herzmann N, Salamon A, Fiedler T, et al. Lipopolysaccharide induces proliferation and osteogenic differentiation of adipose-derived mesenchymal stromal cells in vitro via TLR4 activation. Exp Cell Res. 2017;350(1):115–122.
- Chen XJ, Shen YS, He MC, et al. Polydatin promotes the osteogenic differentiation of human bone mesenchymal stem cells by activating the BMP2-Wnt/beta-catenin signaling pathway. Biomed Pharmacother. 2019;112:108746.
- Salazar VS, Gamer LW, Rosen V. BMP signalling in skeletal development, disease and repair [Review Article]. Nat Rev Endocrinol. 2016;12(4):203.
- Li W, Dunmore BJ, Morrell NW. Bone morphogenetic protein type II receptor mutations causing protein misfolding in heritable pulmonary arterial hypertension. Proc Am Thoracic Soc. 2010;7(6):395–398.
- Conidi A, Cazzola S, Beets K, et al. Few Smad proteins and many Smad-interacting proteins yield multiple functions and action modes in TGFbeta/BMP signaling in vivo. Cytokine Growth Factor Rev. 2011;22(5–6):287–300.
- Handschumacher RE, Harding MW, Rice J, et al. Cyclophilin: a specific cytosolic binding protein for cyclosporin A. Science. 1984;226(4674):544–547.
- Watashi K, Ishii N, Hijikata M, et al. Cyclophilin B is a functional regulator of hepatitis C virus RNA polymerase. Mol Cell. 2005;19(1):111–122.
- Flisiak R, Horban A, Gallay P, et al. The cyclophilin inhibitor Debio-025 shows potent anti-hepatitis C effect in patients coinfected with hepatitis C and human immunodeficiency virus. Hepatology. 2008;47(3):817–826.
- Price ER, Zydowsky LD, Jin MJ, et al. Human cyclophilin B: a second cyclophilin gene encodes a peptidyl-prolyl isomerase with a signal sequence. Proc Natl Acad Sci U S A. 1991;88(5):1903–1907.
- Rycyzyn MA, Reilly SC, O’Malley K, et al. Role of cyclophilin B in prolactin signal transduction and nuclear retrotranslocation. Mol Endocrinol. 2000;14(8):1175–1186.
- Choi JW, Sutor SL, Lindquist L, et al. Severe osteogenesis imperfecta in cyclophilin B-deficient mice. PLoS Genet. 2009;5(12):e1000750.
- Cabral WA, Perdivara I, Weis M, et al. Abnormal type I collagen post-translational modification and crosslinking in a cyclophilin B KO mouse model of recessive osteogenesis imperfecta. PLoS Genet. 2014;10(6):e1004465.
- van Dijk FS, Nesbitt IM, Zwikstra EH, et al. PPIB mutations cause severe osteogenesis imperfecta. Am J Hum Genet. 2009;85(4):521–527.
- Wang B, Lin L, Wang H, et al. Overexpressed cyclophilin B suppresses aldosterone-induced proximal tubular cell injury both in vitro and in vivo. Oncotarget 2016;7(43):69309–69320.
- Oh Y, Kim EY, Kim Y, et al. Neuroprotective effects of overexpressed cyclophilin B against Aximal tubular cell injury both in vitro and in vivo. Biol Med. 2011;51(4):905–920.
- Kim IS, Jeong SJ, Kim SH, et al. Special AT-rich sequence-binding protein 2 and its related genes play key roles in the differentiation of MC3T3-E1 osteoblast like cells. Biochem Biophys Res Commun. 2012;417(2):697–703.
- Mu W, Wang Z, Ma C, et al. Metformin promotes the proliferation and differentiation of murine preosteoblast by regulating the expression of sirt6 and oct4. Pharmacol Res. 2018;129:462–474.
- Shuang Y, Yizhen L, Zhang Y, et al. In vitro characterization of an osteoinductive biphasic calcium phosphate in combination with recombinant BMP2. BMC Oral Health. 2017;17(1):35.
- Teng MR, Huang JA, Zhu ZT, et al. Cyclophilin B promotes cell proliferation, migration, invasion and angiogenesis via regulating the STAT3 pathway in non-small cell lung cancer. Pathol Res Pract. 2019;215(6):152417.
- DeBoer J, Madson CJ, Belshan M. Cyclophilin B enhances HIV-1 infection. Virology 2016;489:282–291.
- Zhao K, Li J, He W, et al. Cyclophilin B facilitates the replication of Orf virus. Virol J. 2017;14(1):114.
- Suh KS, Chon S, Choi EM. Bergenin increases osteogenic differentiation and prevents methylglyoxal-induced cytotoxicity in MC3T3-E1 osteoblasts. Cytotechnology 2018;70(1):215–224.
- Barnes AM, Carter EM, Cabral WA, et al. Lack of cyclophilin B in osteogenesis imperfecta with normal collagen folding. N Engl J Med. 2010;362(6):521–528.
- Jo S, Wang SE, Lee YL, et al. IL-17A induces osteoblast differentiation by activating JAK2/STAT3 in ankylosing spondylitis. Arthritis Res Ther. 2018;20(1):115.
- Zhu J, Tang Y, Wu Q, et al. HIF-1alpha facilitates osteocyte-mediated osteoclastogenesis by activating JAK2/STAT3 pathway in vitro. J Cell Physiol. 2019; 234(11):21182–21192.
- Yu X, Li Z, Wan Q, et al. Inhibition of JAK2/STAT3 signaling suppresses bone marrow stromal cells proliferation and osteogenic differentiation, and impairs bone defect healing. Biol Chem. 2018;399(11):1313–1323.
- Zhang Y, Su J, Teng Y, et al. Nrp1, a neuronal regulator, enhances DDR2-ERK-Runx2 cascade in osteoblast differentiation via suppression of DDR2 degradation. Cell Physiol Biochem. 2015;36(1):75–84.
- Liu DB, Sui C, Wu TT, et al. Association of bone morphogenetic protein (BMP)/Smad signaling pathway with fracture healing and osteogenic ability in senile osteoporotic fracture in humans and rats. Med Sci Monit. 2018;24:4363–4371.