Abstract
Preimplantation genetic testing (PGT) is presently the only option available for detecting genetic conditions in embryos created through in vitro fertilization (IVF) prior to implantation. Thus PGT eliminates the dilemma of pregnancy termination following unfavorable prenatal diagnosis test results. Complex chromosome rearrangements (CCRs), although very rarely reported in humans, represent a significant clinical problem for their carriers. CCRs include at least three breakpoints affecting two or more chromosomes. In three-breakpoint translocations, theoretically sixty-four different chromosomal sets are produced. Only two of them will be balanced and will lead to the birth of a phenotypically normal child. Therefore, CCR carriers are at increased risk of chromosomally unbalanced conceptions, resulting in spontaneous abortions or birth of offspring with intellectual disability and/or congenital anomalies. The reproductive options before such families are: natural conception followed by prenatal diagnosis; donor gametes; adoption or PGT. In this paper we discuss the current state of the PGT field and report on two PGT-cases for CCR in families with infertility and recurrent pregnancy losses. The families underwent IVF-PGT on day 3 and day 5 embryos and whole genome microarray analysis.
Introduction
Preimplantation genetic testing (PGT) is a method for genetic profiling of embryos or oocytes prior to implantation in the uterus. PGT is an addition to assisted reproduction, more specifically, to in vitro fertilization (IVF). The method consists of oocyte or embryo biopsy, DNA isolation, amplification and genetic analysis [Citation1]. The stages of PGT include: preparation for IVF, controlled ovarian stimulation, follicle puncture for oocyte retrieval, sperm collection, IVF or intracytoplasmic sperm injection (ICSI), embryo biopsy, DNA extraction and testing, and transfer of selected embryos into the uterus.
When used to screen for specific lethal genetic diseases, i.e. ones leading to stillbirth or early childhood death, PGT prevents the need for therapeutic abortion and/or any possible medical complications and psychological trauma associated with it. Thus, PGT enables the birth of a child free of the genetic disorder in question [Citation2]. The method is applicable in cases of life-threatening or chronic diseases with severe, multiple, often progressive physical and/or mental disabilities. It is also often used in cases with ineffective or cost-effective therapy [Citation3]. Furthermore, PGT is performed in couples with fertility problems in order to reduce the number of failed transfers, reduce the time to achieve pregnancy and to lower the miscarriage rate.
Over the last twenty years, diagnostic and research capabilities in the field of genetic diseases have improved considerably. More precise, reliable and efficient PGT techniques have emerged, such as array comparative genomic hybridization (aCGH), single-nucleotide polymorphism arrays, karyomapping, and next-generation sequencing (NGS) [Citation4]. Array CGH is still widely used to detect quantitative changes in the genome. It is informative for autosomal and gonosomal aneuploidies, deletions/duplications and high-level mosaicism. Currently, NGS is regarded as a PGT technique with superior accuracy for chromosomal mosaicism compared to aCGH, quantitative polymerase chain reaction (qPCR) and single nucleotide polymorphism (SNP) array. NGS has also been proven to be highly effective in identifying segmental losses/gains, with improved precision than previous methods [Citation5]. Furthermore, reports from IVF centers indicate increased implantation rate (71.6% vs. 64.6%) and live birth rate (62% and 54.4% %) after NGS-PGT compared to aCGH-PGT array [Citation6]. Biopsy for PGT can be performed on cells from different developmental stages: polar bodies, day 3 cleavage-stage embryos and trophectoderm cells [Citation7].
Currently, PGT is divided into three main categories: PGT for monogenic disorders (PGT-M), PGT for structural rearrangements (PGT-SR) and PGT for aneuploidy (PGT-A) [Citation8]. PGT-M is applied in families in which one or both parents are carriers of a known monogenic defect. The procedure identifies embryos carrying specific genetic mutations. A more recent application of PGT-M is to diagnose late-onset diseases and cancer predisposition syndromes [Citation9, Citation10]. Most of the monogenic inherited cancer syndromes have a very high penetrance. PGT-M has been successfully performed in cases of familial adenomatous polyposis, familial melanoma, juvenile polyposis syndrome, type I and type II neurofibromatosis, etc. Since affected individuals remain healthy until the onset of the disease, there is ongoing ethical concern on whether PGT-M is acceptable in these cases.
PGT-SR is applied in families where one or both parents have a structural chromosomal abnormality: balanced chromosomal aberration, deletion or duplication [Citation11]. Approximately 1.2–6.9% of infertile couples carry a balanced rearrangement [Citation12]. In most cases the carriers are phenotypically normal but are at a significantly increased risk of infertility, miscarriage, fetal developmental delay. It is also associated with an elevated risk of chromosomal abnormalities among offspring, as a result of the formation of unbalanced gametes during meiosis [Citation13]. PGT-SR ensures the selection of balanced/normal embryos and thus prevents the transmission of unbalanced chromosomal rearrangements to the offspring [Citation14].
PGT-A (formerly known as preimplantation genetic screening, PGS) identifies embryos with de novo aneuploidies, deletions or duplications. PGT-A is often recommended in cases of advanced maternal age, recurrent implantation failure, recurrent miscarriages, or severe male factor infertility [Citation15]. De novo chromosomal aberrations have been reported in up to 75% of cleavage-stage embryos and in 50% of blastocysts. The majority of these anomalies result in implantation failure or miscarriage [Citation16].
In this article we focus on two couples who underwent PGT-SR for CCRs. Phenotypically normal CCR subjects have significant risk of reproductive failure. They may suffer from infertility or subfertility, recurrent abortions and/or increased risk of chromosomally unbalanced offspring [Citation17]. The risk of recurrent spontaneous abortions is estimated to be from 50 to 100%, whereas the one for birth of chromosomally unbalanced offspring ranges from 20 to 90% [Citation18].
Subjects and methods
Subjects
We report on two couples with CCR carriers: one with history of recurrent miscarriages and one with infertility history and four failed IVF attempts. Both families underwent an IVF-PGT-SR procedure after extensive genetic counseling. Informed consent was obtained from all individual participants included in the study.
Sampling
Chromosomes were isolated from blood lymphocytes cultured in Chromosome P medium (EuroClone) using a standard protocol. In the first couple, chromosomal analysis was performed as part of the standard procedure for clinical and laboratory assessment of couples with recurrent miscarriages. In the second case, cytogenetic analysis was conducted after finding chromosomal aberrations in a relative.
Trophectoderm biopsy was carried out on a total of fifteen blastocyst and four cleavage-stage embryos. The cells were amplified by whole genome amplification through SurePlex Amplification kit (Illumina). ArrayCGH was performed by 24sure + microarrays (Illumina).
Results were analyzed by BlueFuseMulti software.
Results and discussion
In case I, fourteen embryos were tested. The analysis showed that three of them had a balanced profile and eleven had an unbalanced profile. The woman delivered a healthy male child. In case II, a total of five embryos were tested but no balanced embryos were available for transfer.
Case I
A non-consanguineous couple (a 35-year-old female and a 39-year-old male) with a history of recurrent miscarriages was referred for genetic counseling. The couple had suffered two first trimester miscarriages. The cause and genetic status of the products of conception were unknown. There was no evidence of any uterine abnormality or any other underlying condition that may lead to unsuccessful pregnancy.
The chromosomal analysis revealed a normal karyotype in the male partner - 46, XY, and an abnormal karyotype in the female partner 46, XХ,t(3;6;7)(3pter→3q13.2::7p22→7pter;6pter→6q21::3q13.2→3qter;7qter→7p22::6q21→6qter) - CCR between chromosomes 3, 6 and 7 (). First-degree family members were also referred for cytogenetic evaluation and the proband’s first cousin was found to carry the same translocation.
Figure 1. Karyotype of the female patient showing the three-way exchange involving chromosomes 3, 6 and 7 in Case I: 46, XХ,t(3;6;7)(3pter→3q13.2::7p22→7pter;6pter→6q21::3q13.2→3qter;7qter→7p22::6q21→6qter).
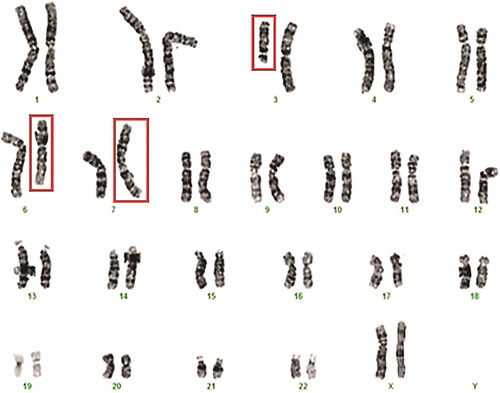
A total of thirty-six oocytes were harvested through a variety of ovarian stimulation protocols or natural IVF cycles, over a two-year period. Fourteen ICSI procedures were performed. The first four cycles resulted in retrieval of nine oocytes. A cleavage-stage biopsy was performed on four embryos. All of the embryos were abnormal/unbalanced (, ).
Figure 2. PGT results from all fourteen tested embryos in Case I. Eleven embryos were unbalanced (embryos 2, 3, 7, 8-1, 8-2, 11, 14, 17, 21, 24 and 27) and three embryos were balanced (embryos 10, 18 and 23).
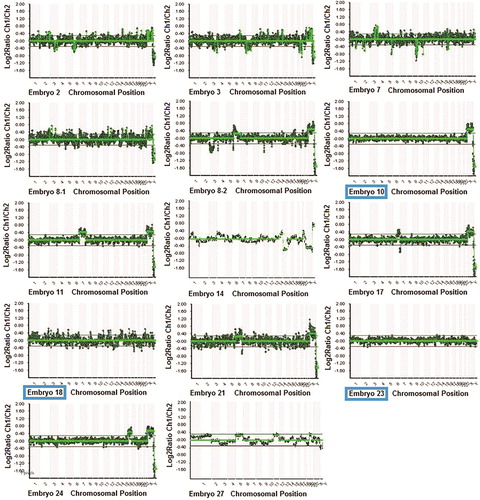
Table 1. PGT results from all fourteen tested embryos in Case I. Eleven embryos were unbalanced (embryos 2, 3, 7, 8-1, 8-2, 11, 14, 17, 21, 24 and 27), and three embryos were normal/balanced (embryos 10, 18 and 23).
A total of twenty-seven mature oocytes were retrieved from the next ten IVF cycles. Twenty five of them were successfully fertilized, and all embryos reached cleavage stage. Only ten embryos reached blastocyst stage and were biopsied. The results showed three balanced and seven unbalanced embryos (, ).
Two embryos were transferred on day 6 after fertilization. The woman successfully conceived. The couple was advised to do prenatal testing as part of the standard PGT follow-up procedure. However, the patient refused to undergo amniocentesis despite the recommendations of the prenatal and genetic specialists. Ultrasound scans during pregnancy did not reveal any apparent fetal anomalies. A male child with a normal phenotype and no visible congenital anomalies was delivered via Cesarean section due to maternal medical comorbidities (anxiety and depression disorder, Hashimoto’s thyroiditis, coagulopathy, varicose veins and advanced maternal age). Karyotyping revealed normal male karyotype 46, XY.
Case II
A non-consanguineous couple (a 27-year-old female and a 28-year-old male) with a history of infertility of unknown cause and four failed IVF attempts was referred for genetic counseling. The male patient’s sister was found to be carrier of a balanced three-way CCR between chromosomes 1, 8 and 11.
Chromosomal analysis revealed normal karyotype in the female partner - 46, XX, and abnormal karyotype in the male partner 46, XY,t(1;8;11)(1qter→1p22::8q11.1→8qter)(8pter→8q11.1::11q22→11qter)(11pter→11q22::1p22→1pter): CCR between chromosomes 1, 8 and 11 ().
Figure 3. Karyotype of the male patient showing the three-way exchange involving chromosomes 1, 8 and 11 in Case II: 46, XY,t(1;8;11)(1qter→1p22::8q11.1→8qter)(8pter→8q11.1::11q22→11qter)(11pter→11q22::1p22→1pter).
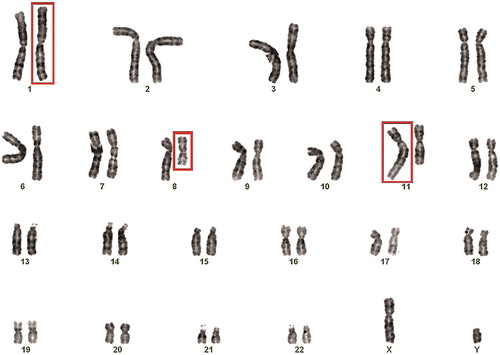
After a standard long protocol, nine oocytes were retrieved and the subsequent ICSI procedure was performed with fresh sperm. All oocytes were successfully fertilized and the resulting embryos reached cleavage stage. One day 3, one embryo was thawed from a past IVF attempt. Five embryos reached the blastocyst stage. All of them were diagnosed as unbalanced by PGT-SR analysis (, ).
Figure 4. PGT results from all five tested embryos in Case II. All five embryos were unbalanced (embryos 1, 3, 5, 8 and 10).
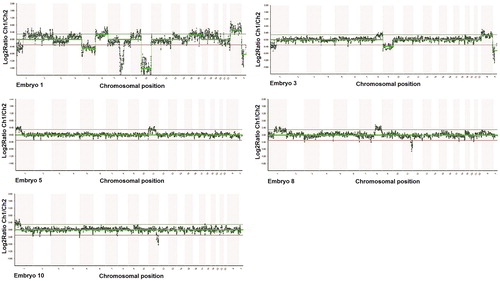
Table 2 PGT results from all five tested embryos in Case II. All embryos were unbalanced (embryos 1, 3, 5, 8 and 10).
CCRs: mechanisms of generation and transmission
The molecular mechanisms underlying the genesis of CCRs remain elusive even today. The use of fluorescence in situ hybridization (FISH) and molecular techniques for CCR characterization have revealed that chromosomal rearrangements might be more complex than initially presumed. Several mechanisms for the occurrence of CCRs have been proposed, including non-allelic homologous recombination (NAHR) and nonhomologous end-joining (NHEJ) [Citation19]. Both NAHR and NHEJ account for deleted or duplicated genomic segments. Other possible mechanisms for CCR formation are fork stalling, template switching (FoSTeS) and microhomology-mediated break-induced replication (MMBIR) [Citation19]. In recent studies, simultaneous double-strand DNA breaks induced by free radicals or ionizing radiation, followed by wrongful joining of the break fragments have been associated with CCRs [Citation20].
Cases with three-way exchange or four-way exchange involving three chromosomes, may lead to production of unbalanced gametes. During the first meiotic division, a hexavalent configuration is formed allowing four patterns of segregation: 3:3, 4:2, 5:1 and 6:0. Theoretically, sixty-four different gametic chromosomal combinations can occur. Out of those combinations, only two are normal/balanced: one normal haploid gamete and one balanced haploid gamete. The other sixty-two combinations have a wide variety of imbalances due to the chromosome malsegregation. If the meiotic disjunction is symmetrical (3:3 mode), twenty different gametic chromosomal combinations can occur: one normal, one balanced and eighteen unbalanced combinations. If the disjunction is asymmetric (4:2, 5:1 or 6:0 modes), forty-four differently unbalanced gametes are produced (thirty types for 4:2, twelve for 5:1 and two for 6:0, respectively).
The majority of documented CCR cases involve maternal transmission, indicating that human oogenesis is able to cope with the complexity of CCRs and produce phenotypically normal children. Most female CCR carriers are identified due to advanced maternal age, repeated spontaneous abortions, or birth of a child with a genetic abnormality [Citation21]. The majority of reported CCRs in males are detected due to infertility problems [Citation22, Citation23]. Typically, CCR male carriers tend to be infertile or subfertile because of hypospermatogenesis or spermatogenic arrest caused by complex meiotic configurations. Consequently, the paternal transmission of CCRs is considered to be scarce, although there are reports of a few rare cases [Citation24–26].
Conclusion
In spite of the improved prenatal diagnosis options, balanced CCR carriers still face serious challenges, such as inability to achieve pregnancy, recurrent miscarriages, pregnancy termination of an affected fetus, overcoming social, religious and ethical stigma associated with elective pregnancy termination. In couples with a CCR carrier, PGT could be considered as a suitable option, allowing the selection of balanced embryos, thus reducing the risk of unsuccessful implantation, and miscarriage.
Ethics statement
All procedures followed were in accordance with the ethical standards of the responsible committee on human experimentation (institutional and national) and with the Helsinki Declaration of 1975, as revised in 2000. Informed consent was obtained from all patients for being included in the study.
Disclosure statement
The authors report no conflict of interest.
References
- Harper JC, Wilton L, Traeger-Synodinos J, et al. The ESHRE PGD Consortium: 10 years of data collection. Hum Reprod Update. 2012;18(3):234–247.
- Spinella F, Fiorentino F, Biricik A, et al. Extent of chromosomal mosaicism influences the clinical outcome of in vitro fertilization treatments. Fertil Steril. 2018;109(1):77–83.
- EURODIS. Rare Diseases: understanding this Public Health Priority: EURODIS: European Organization for Rare Diseases; November 2005. Available from: www.eurordis.org.
- Garvin SE, Chatzicharalampous C, Puscheck E. Reflections on preimplantation genetic testing for aneuploidy and mosaicism: how did we get here, and what does it mean clinically? Fertil Steril. 2019;111(1):45–47.
- Victor AR, Griffin DK, Brake AJ, et al. Assessment of aneuploidy concordance between clinical trophectoderm biopsy and blastocyst. Hum Reprod. 2019;34(1):181–192.
- Friedenthal J, Maxwell SM, Munne S, et al. Next generation sequencing for preimplantation genetic screening improves pregnancy outcomes compared with array comparative genomic hybridization in single thawed euploid embryo transfer cycles. Fertil Steril. 2018;109(4):627–632.
- Poli M, Girardi L, Fabiani M, et al. Past, present, and future strategies for enhanced assessment of embryo's genome and reproductive competence in women of advanced reproductive age. Front Endocrinol (Lausanne). 2019;10:154.
- Rosenwaks Z, Handyside AH, Fiorentino F, et al. The pros and cons of preimplantation genetic testing for aneuploidy: clinical and laboratory perspectives. Fertil Steril. 2018;110(3):353–361.
- Rechistky S, Kuliev A. Preimplantation genetic testing (PGT) for borderline indications – PGT for cancer: Reproductive Biomedicine Online; 2018.
- Ethics Committee ASRA. Use of preimplantation genetic testing for monogenic defects (PGT-M) for adult-onset conditions: an Ethics Committee opinion. Fertility Sterility 2018;109(6):989–992.
- Hu L, Cheng D, Gong F, et al. Reciprocal Translocation Carrier Diagnosis in Preimplantation Human Embryos. EBioMedicine 2016;14:139–147.
- Gardner RJ, Sutherland G, Shaffer L. Chromosome abnormalities and genetic counseling. 4th ed. Oxford: Oxford University Press; 2012.
- Zhang L, Wei D, Zhu Y, et al. Interaction of acrocentric chromosome involved in translocation and sex of the carrier influences the proportion of alternate segregation in autosomal reciprocal translocations. Hum Reprod. 2019;34(2):380–387.
- Iews M, Tan J, Taskin O, et al. Does preimplantation genetic diagnosis improve reproductive outcome in couples with recurrent pregnancy loss owing to structural chromosomal rearrangement? A systematic review. Reprod Biomed Online. 2018;36(6):677–685.
- Practice Committee ASRMA. Practice Committee SART. The use of preimplantation genetic testing for aneuploidy (PGT-A): a committee opinion. Fertil Steril. 2018;09(3):429–436.
- Popovic M, Dhaenens L, Taelman J, et al. Extended in vitro culture of human embryos demonstrates the complex nature of diagnosing chromosomal mosaicism from a single trophectoderm biopsy. Hum Reprod. 2019;34(4):758–769.
- Pellestor F, Anahory T, Lefort G, et al. Complex chromosomal rearrangements: origin and meiotic behavior. Hum Reprod Update. 2011;17(4):476–494.
- Batista DA, Pai GS, Stetten G. Molecular analysis of a complex chromosomal rearrangement and a review of familial cases. Am J Med Genet. 1994;53(3):255–263.
- Gu W, Zhang F, Lupski JR. Mechanisms for human genomic rearrangements. Pathogenetics. 2008;1(1):4.
- Hemmat M, Yang X, Chan P, et al. Characterization of a complex chromosomal rearrangement using chromosome, FISH, and microarray assays in a girl with multiple congenital abnormalities and developmental delay. Mol Cytogenet. 2014;7(1):50.
- Zhang Y, Dai Y, Tu Z, et al. Array-CGH detection of three cryptic submicroscopic imbalances in a complex chromosome rearrangement. J Genet. 2009;88(3):369–372.
- Bartels I, Starke H, Argyriou L, et al. An exceptional complex chromosomal rearrangement (CCR) with eight breakpoints involving four chromosomes (1;3;9;14) in an azoospermic male with normal phenotype. Eur J Med Genet. 2007;50(2):133–138.
- Salahshourifar I, Shahrokhshahi N, Tavakolzadeh T, et al. Complex chromosomal rearrangement involving chromosomes 1, 4 and 22 in an infertile male: case report and literature review. J Appl Genet. 2009;50(1):69–72.
- Gruchy N, Barreau M, Kessler K, et al. A paternally transmitted complex chromosomal rearrangement (CCR) involving chromosomes 2, 6, and 18 includes eight breakpoints and five insertional translocations (ITs) through three generations. Am J Med Genet. 2010;152A(1):185–190.
- Aristidou C, Theodosiou A, Ketoni A, et al. Cryptic breakpoint identified by whole-genome mate-pair sequencing in a rare paternally inherited complex chromosomal rearrangement. Mol Cytogenet. 2018;11(1):34.
- Basinko A, Perrin A, Nguyen HA, et al. Balanced transmission of a paternal complex chromosomal rearrangement involving chromosomes 2, 3, and 18. Am J Med Genet. 2010;152A(10):2646–2650.