Abstract
This study investigated the expression of plasminogen activator inhibitor-1 (PAI-1) and microRNA (miRNA or miR)-30b in tumor tissues, blood and urine from pancreatic cancer (PC) patients with diabetes mellitus. The effect of culture supernatant of PC cells with up-regulated expression of miR-30b on β cells was also investigated. Thirty-one PC patients with diabetes mellitus and 22 PC patients without diabetes mellitus were included. PC tissues and tumor-adjacent tissues, peripheral blood and urine were collected from all PC patients. Quantitative real-time polymerase chain reaction was used to determine the mRNA and miR-30b expression; Western blotting, to determine the expression of PAI-1 protein in tissues; enzyme-linked immunosorbent assay, to determine the PAI-1 content in serum or urine and dual luciferase reporter assay, to identify direct interaction between miR-30b and PAI-1 mRNA. The proliferation of INS-1 cells was determined by MTT assay. The results showed that PAI-1 mRNA and protein expression was elevated in PC patients with diabetes in contrast to that in PC patients without diabetes. By contrast, miR-30b expression was decreased in PC patients with diabetes. miR-30b could bind with the 3′-UTR seed region of PAI-1 mRNA to regulate its expression. Overexpression of miR-30b inhibited the expression of PAI-1, altered the microenvironment surrounding PC cells and promoted the proliferation of islet β-cells. These results suggest that the expression of PAI-1 in PC patients with diabetes is up-regulated, being correlated with the reduced expression of miR-30b. miR-30b is involved in the pathological process of diabetes mellitus induced by PC by regulating PAI-1.
Introduction
Pancreatic cancer (PC) has a very high morbidity and mortality rate in the United States [Citation1,Citation2]. In China, the incidence of PC is also increasing year by year [Citation3]. PC is often associated with malignant diseases such as diabetes mellitus. It is reported that more than 80% of PC patients are complicated by diabetes mellitus [Citation4], which has long been considered both a cause and a consequence of PC [Citation5]. PC cells alter the function of islet cells and glycometabolism of surrounding tissues (such as liver and muscle tissues) by secreting soluble factors, which may be a factor inducing diabetes mellitus [Citation6,Citation7]. Monitoring changes in the genome or proteome of pancreatic islets or surrounding tissues will be of great significance for the disease development in patients with early PC [Citation8].
Plasminogen activator inhibitor-1 (PAI-1) is a kind of serine protease inhibitor that can inactivate plasminogen activators t-PA and u-PA, inhibit the process of intravascular fibrinolysis, cause changes in hemorheology and aggravate ischemic injury [Citation9–11]. Studies show that microRNA (miRNA or miR) can directly target mRNA to regulate gene expression in organisms [Citation12,Citation13]. In addition, microRNA in circulating blood is also a reliable biological marker associated with many diseases including hypoxic-ischemic encephalopathy [Citation14,Citation15]. It is also reported that miR-30b regulates the proliferation and apoptosis of gastric cancer cells by targeting PAI-1 gene [Citation16]. However, the mechanism of miR-30b regulating PAI-1 in PC patients with diabetes mellitus has not been reported.
In the present study, we determined the expression of PAI-1 and miR-30b in tumor tissues, blood and urine from PC patients with diabetes mellitus. In addition, the effect of culture supernatant of PC cells with up-regulated expression of miR-30b on β-cells was also investigated.
Subjects and methods
Ethics statement
All procedures performed in the present study were approved by the Ethics Committee of Jinzhou Medical University. Written informed consent was obtained from all patients or their families.
Subjects
A total of 31 PC patients with diabetes mellitus who received surgical treatment at our hospital between January 2016 and June 2018 were included into the experimental group, whereas 22 PC patients without diabetes mellitus who were treated surgically in our hospital during the same period were included into the control group. PC tissues and tumor-adjacent tissues were collected from all PC patients. Fasting peripheral blood (5 mL) and urine (5 mL) were collected from all patients and stored at –20 °C before use. Among the 31 PC patients in the experimental group, 18 were males and 13 were females, with an age range of 33 to 69 years and a median age of 52.5 years. Among the 22 PC patients in the control group, 12 were males and 10 were females, with an age range of 31 to 71 years and a median age of 53.3 years. TNM staging was performed according to previously published staging criteria [Citation17]. Among the PC patients with diabetes mellitus, 2 cases were at stage I, 1 case was at stage II, 8 cases were at stage III and 20 cases were at stage IV. Among the PC patients without diabetes mellitus, 2 cases were at stage I, 2 cases were at stage II, 5 cases were at stage III and 13 cases were at stage IV.
Quantitative real-time polymerase chain reaction (qRT-PCR)
Tissues (100 mg) were ground into powder in liquid nitrogen, and lysed using 1 mL TRIzol reagent following the manufacturer’s manual (Thermo Fisher Scientific, Waltham, MA, USA). Liquid samples (100 μL) were directly lysed using 1 mL TRIzol reagent following the manufacturer’s manual (Thermo Fisher Scientific, Waltham, MA, USA). Total RNA was extracted using the phenol/chloroform method. The concentration and quality of RNA was measured using ultraviolet spectrophotometry (Nanodrop ND2000, Thermo Scientific, Waltham, MA, USA). Then, cDNA was obtained by reverse transcription from 1 μg RNA and stored at –20 °C. Reverse transcription of mRNA was performed using TIANScript II cDNA First Strand Synthesis Kit (Tiangen, Beijing, China), and reverse transcription of miRNA was carried out using miRcute miRNA cDNA First Strand Synthesis Kit (Tiangen, Beijing, China).
SuperReal PreMix (SYBR Green) qRT-PCR kit (Tiangen, Beijing, China) was used to detect mRNA expression of PAI-1, using GAPDH (glyceraldehyde 3-phosphate dehydrogenase) as internal reference. The primer sequences for PAI-1 were 5′- AATGACTGGGTGAAGACACACACA -3′(forward) and 5′- TTCCACTGGCCGTTGAAGTAGA -3′ (reverse). The primer sequences for GAPDH were 5′- AAGGCTGTGGGCAAGG -3′ (forward) and 5′- TGGAGGAGTGGGTGTCG -3′ (reverse). The reaction system (20 μL) was composed of 10 μL SYBR Premix EXTaq, 0.5 μL upstream primer, 0.5 μL downstream primer, 2 μL cDNA and 7 μL ddH2O. The PCR condition was: initial denaturation at 95 °C for 2 min; denaturation at 95 °C for 25 s and annealing at 55 °C for 30 s (40 cycles); elongation at 72 °C for 30 s (iQ5; Bio-Rad, Hercules, CA, USA). The 2–ΔΔCt method [Citation17] was used to calculate the relative expression of PAI-1 mRNA against GAPDH. Each sample was tested in triplicate.
The expression of miR-30b was determined by miRcute miRNA RT-PCR Kit (Tiangen, Beijing, China), using U6 as internal reference. The sequences of miR-30b primers were 5′- CGCGCTGTAAACATCCTACAC -3′(forward), and 5′- GTGCAGGGTCCGAGGT -3′ (reverse). The sequences of U6 primers were 5′- GCTTCGGCAGCACATATACTAAAAT -3' (forward) and 5'- CGCTTCACGAATTTGCGTGTCAT -3′ (reverse). The reaction system (20 μL) contained 10 μL qRT-PCR-Mix, 0.5 μL upstream primer, 0.5 μL downstream primer, 2 μL cDNA and 7 μL ddH2O. The reaction protocol was: initial denaturation at 95 °C for 5 min; 95 °C for 10 s and 60 °C for 20 s (40 cycles); elongation at 72 °C for 10 s (iQ5; Bio-Rad, Hercules, CA, USA). The 2–ΔΔCt method [Citation17] was used to calculate the relative expression of miR-30b against U6. Each sample was tested in triplicate.
Western blotting
Before lysis, tissues (100 mg) were ground into powder. Then, tissue samples were lysed with precooled Radio-Immunoprecipitation Assay (RIPA) lysis buffer (600 μL; 50 mmol/L Tris-base, 1 mmol/L ethylenediaminetetraacetic acid, 150 mmol/L NaCl, 0.1% sodium dodecyl sulfate, 1% TritonX-100, 1% sodium deoxycholate; Beyotime Institute of Biotechnology, Shanghai, China) for 30 min on ice. The mixture was centrifuged at 10,000 rpm and 4 °C for 10 min. The supernatant was used to determine the protein concentration by a bicinchoninic acid (BCA) protein concentration determination kit (RTP7102, Real-Times Biotechnology Co., Ltd., Beijing, China). The samples were then mixed with 5× sodium dodecyl sulfate loading buffer before denaturation in boiling water bath for 5 min. Afterwards, the samples (20 µg) were subjected to 10% sodium dodecyl sulfate-polyacrylamide gel electrophoresis at 100 V. The resolved proteins were transferred to polyvinylidene difluoride membranes on ice (100 V, 2 h) and blocked with 5% skimmed milk at room temperature for 1 h. Then, the membranes were incubated with rabbit anti-human PAI-1 (1:1000; ab125687; Abcam, Cambridge, UK) or β-actin (1:2500; ab8227; Abcam, Cambridge, UK) polyclonal primary antibodies at 4 °C overnight. After extensive washing with phosphate-buffered saline with Tween 20 (3 times of 15 min), the membranes were incubated with goat anti-rabbit horseradish peroxidase-conjugated secondary antibody (1:3000; ab6721; Abcam, Cambridge, UK) for 1 h at room temperature before washing with phosphate-buffered saline with Tween 20 (3 times of 15 min). Then, the membrane was developed with an enhanced chemiluminescence detection kit (ab65623; Abcam, Cambridge, UK) for imaging. Image lab v3.0 software (Bio-Rad, Hercules, CA, USA) was used to acquire and analyze the imaging signals. The relative content of target protein was expressed against β-actin.
Enzyme-linked immunosorbent assay (ELISA)
Contents of PAI-1 in serum and urine were tested by ELISA using a PAI-1 ELISA kit (ab184863; Abcam, Cambridge, UK). In microplates, standards (50 μL) and samples (10 μL serum and 40 μL diluent) were added into predefined wells, while blank wells were left empty. In the wells for standards and samples, horseradish peroxidase-labelled conjugates (100 μL) were added before sealing the plates for incubation at 37 °C for 1 h. After washing the plates 5 times, substrates A (50 μL) and B (50 μL) were added into each well. After incubation at 37 °C for 15 min, stop solution (50 μL) was added into each well, and the absorbance of each well was measured at 450 nm within 15 min.
Bioinformatics
Bioinformatics prediction is a powerful tool for the study of the functions of miRNAs. We used miRanda (http://34.236.212.39/microrna/home.do) to predict genes that might regulate PAI-1.
Dual luciferase reporter assay
According to the bioinformatics results, wild-type (WT) and mutant seed regions of miR-30b in the 3′-UTR (untranslated region) of PAI-1 gene were chemically synthesized in vitro. Then, their two ends were attached with Spe-1 and HindIII restriction sites, and then cloned into pMIR-REPORT luciferase reporter plasmids. Plasmids (0.8 μg) with WT or mutant 3′-UTR sequences were co-transfected with agomiR-30b (100 nmol/L; forward, 5′- UGUAAACAUCCUACACUCAGCU -3′; reverse, 3′- ACAUUUGUAGGAUGUGAGUCGA -5′; Sangon Biotech, Shanghai, China) into 293 T cells (Type Culture Collection, Chinese Academy of Sciences, Shanghai, China). For control, 293 T cells were transfected with agomiR-negative control (NC; forward, 5′- UUCUCCGAACGUGUCACGUTT -3′; reverse, 3′- TTAAGAGGCUUGCACAGUGCA -5′; Sangon Biotech, Shanghai, China). After cultivation for 24 h, the cells were lysed using dual luciferase reporter assay kit (E1980; Promega, Fitchburg, WI, USA) according to the manufacturer’s manual, and luminescence intensity was measured using GloMax 20/20 luminometer (Promega, Fitchburg, WI, USA). Using renilla luminescence activity as internal reference, the luminescence values of each group of cells were measured.
Cells
To transfect PANC-1 cells (Type Culture Collection, Chinese Academy of Sciences, Shanghai, China) with miR-30b mimics, the cells (3 × 105) in logarithmic growth were seeded onto 24-well plates one day before transfection, and cultured in antibiotics-free F12/DMEM medium supplemented with 10% fetal bovine serum until reaching 70% confluency. In the first vial, 1.5 µL agomiR-negative control (20 pmol/µL; miR-NC group; forward, 5′- UUCUCCGAACGUGUCACGUTT -3′; reverse, 3′- TTAAGAGGCUUGCACAGUGCA -5′) or agomiR-30b (20 pmol/µL; miR-30b mimics group; forward, 5′- UGUAAACAUCCUACACUCAGCU -3′; reverse, 3′- ACAUUUGUAGGAUGUGAGUCGA -5′) (Sangon Biotech, Shanghai, China) was mixed with 50 µL Opti Mem medium (Thermo Fisher Scientific, Waltham, MA, USA). In the second vial, 1 µL Lipofectamine 2000 (Thermo Fisher Scientific, Waltham, MA, USA) was mixed with 50 µL Opti Mem medium. After standing still for 5 min, the two vials were combined for additional waiting at room temperature for 20 min. Then, the mixtures were added onto cells in respective groups. Six hours later, the medium was replaced with F12/DMEM medium containing 10% fetal bovine serum. After cultivation for 48 h, the cells were collected for further assays.
MTT assay
After transfection, islet INS-1 β-cells (Type Culture Collection, Chinese Academy of Sciences, Shanghai, China) were seeded into 96-well plates at a density of 2 × 103 cells per well. Each condition was tested in triplicate wells. At 24, 48 and 72 h after transfection, 20 µL MTT (5 g/L; JRDC000003, JRDUN Biotechnology, Shanghai, China) was added to each well, followed by incubation for 4 h at 37˚C. After aspiration of medium, dimethyl sulfoxide (DMSO; 150 µL per well) was added to dissolve purple crystals. Then, the absorbance of each well was measured at 490 nm with a microplate reader (Bio-Rad Laboratories, Inc.) and cell proliferation curves were plotted.
Statistical analysis
The results were analyzed using SPSS 18.0 statistical software (IBM, Armonk, NY, USA). The data were expressed as means with standard deviations (±SD). Data were tested for normality. Multigroup measurement data were analyzed using one-way analysis of variance (ANOVA). In case of homogeneity of variance, Least Significant Difference and Student-Newman-Keuls methods were used; in case of heterogeneity of variance, Tamhane’s T2 or Dunnett’s T3 method was used. Comparison between two groups was carried out using Student’s t-test. p < 0.05 indicated statistically significant differences.
Results and discussion
PAI-1 mRNA expression is elevated in PC patients with diabetes
The occurrence and development of PC is a complex multistep process involving multiple genes and accompanied by epigenetic changes. With the development of biotherapy, it is very important to elucidate the pathogenesis of PC and find new therapeutic gene targets. Invasion and metastasis of tumors are closely related to the degradation of extracellular matrix by autocrine proteases, in which fibrinolytic enzymes and their inhibitors are important degradation enzymes involved in invasion and metastasis of tumors [Citation18]. uPA promotes mitosis in vitro and indirectly increases the possibility of invasion and metastasis of tumors [Citation19,Citation20]. PAI-1 is a protease inhibitor that can inhibit the action of uPA by forming a strong complex with uPA. However, synchronized overexpression of uPA and PAI-1 in breast and endometrial cancers is associated with the progression of cancer, suggesting the complexity of the mechanism of PAI-1 in tumorigenesis and development [Citation21,Citation22].
To determine the expression of PAI-1 mRNA, qRT-PCR was performed. The data showed that the levels of PAI-1 mRNA in tumor tissues from PC patients with or without diabetes mellitus were significantly higher than that from tumor-adjacent tissues from all PC patients (p < 0.05), whereas that from PC patients with diabetes was significantly higher than that from PC patients without diabetes (p < 0.05) (. Moreover, the levels of PAI-1 mRNA in serum and urine from PC patients with diabetes were higher than those from PC patients without diabetes (p < 0.05 for both) (). The results suggest that PAI-1 mRNA expression is elevated in PC patients with diabetes.
Figure 1. Relative expression of PAI-1 mRNA in PC. (A) Relative expression of PAI-1 mRNA in tumor tissues from PC patients with or without diabetes and tumor-adjacent tissues from all PC patients. *p < 0.05 and **p < 0.01 compared with tumor-adjacent tissues. #p < 0.05 compared with tumor tissues from PC patients without diabetes. Relative expression of PAI-1 mRNA in serum (B) and urine (C) from PC patients with or without diabetes. *p < 0.05 and **p < 0.01 compared with PC patients without diabetes. qRT-PCR was used to determine the expression of mRNA.
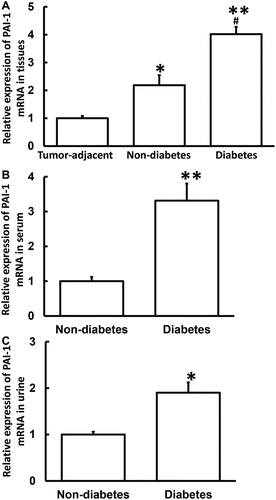
PAI-1 protein expression is increased in tumor tissues from PC patients with diabetes
To determine the expression of PAI-1 protein in tissues, Western blotting was carried out. The data showed that PAI-1 protein expression in tumor tissues from PC patients with or without diabetes was significantly higher than that from tumor-adjacent tissues from all PC patients (p < 0.05), whereas that from PC patients with diabetes was significantly higher than that from PC patients without diabetes (p < 0.05) (). The result indicates that PAI-1 protein expression is increased in tumor tissues from PC patients with diabetes.
Figure 2. Relative expression of PAI-1 protein in tumor tissues from PC patients with or without diabetes and tumor-adjacent tissues from all PC patients. Western blotting was used to determine the expression of protein in tissues. *p < 0.05 and **p < 0.01 compared with tumor-adjacent tissues. ##p < 0.01 compared with tumor tissues from PC patients without diabetes.
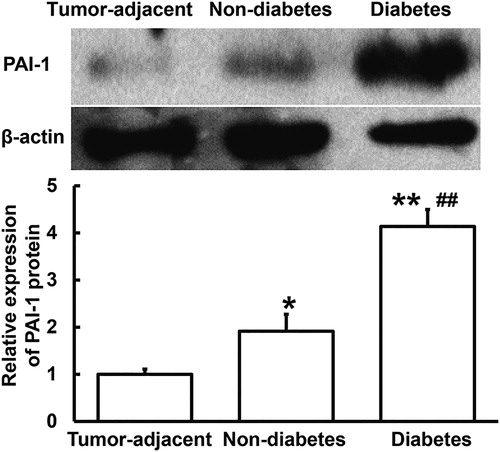
Secretion of PAI-1 protein to blood and urine is increased in PC patients with diabetes
To determine the content of PAI-1 protein in serum and urine, ELISA was used. The data showed that the PAI-1 protein contents in serum and urine from PC patients with diabetes were significantly elevated (p < 0.05 for both) compared to those from PC patients without diabetes (). The results suggest that secretion of PAI-1 protein to blood and urine is increased in PC patients with diabetes. It is reported that PAI-1 exerts its biological function mainly by interacting with cell surface molecules [Citation23,Citation24]. The change of plasma PAI-1 concentration has certain clinical significance in the diagnosis of PC with diabetes mellitus, and can be used as one of the auxiliary diagnostic indicators.
miR-30b expression is decreased in PC patients with diabetes
The regulation of the transcription and expression of mRNA is a complex process involving multiple factors. We used bioinformatics to predict the upstream genes regulating PAI-1. In the meantime, we referred to related literature for microRNAs that can regulate PAI-1, and found that miR-30b may be one of the upstream target genes regulating PAI-1 [Citation25]. MicroRNAs can achieve negative feedback regulation of their target genes by cutting the mRNA and inhibiting their translation. They are important regulators in normal development, physiology and disease state. In addition, many microRNAs have become biomarkers of various diseases [Citation26,Citation27]. miR-30b is a member of the miR-30 family [Citation28], and is involved in many processes, such as inflammatory reaction, malignant behavior of tumors and epithelial-mesenchymal transformation [Citation29–32]. It is reported that miR-30b affects breast development and degeneration [Citation33], and regulates human endometrial receptivity [Citation34]. In addition, miR-30b is also associated with the development of human neural tube cells and the occurrence and development of tumors, and its expression is also associated with schizophrenia [Citation35]. In melanoma, miR-30b promotes metastasis by regulating the expression of acetylglucosaminyltransferase [Citation36].
To determine the expression of miR-30b, qRT-PCR was performed. The data showed that the level of miR-30b in tumor tissues from PC patients with or without diabetes mellitus was significantly lower than that from tumor-adjacent tissues from all PC patients (p < 0.05), wheras that from PC patients with diabetes was significantly lower than that from PC patients without diabetes (p < 0.05) (. In addition, the level of miR-30b in serum and urine from PC patients with diabetes was lower than that from PC patients without diabetes (p < 0.05 for both) (). The results suggest that miR-30b expression is decreased in PC patients with diabetes.
Figure 4. Relative expression of miR-30b in PC. (A) Relative expression of miR-30b in tumor tissues from PC patients with or without diabetes and tumor-adjacent tissues from all PC patients. *p < 0.05 compared with tumor-adjacent tissues. #p < 0.05 compared with tumor tissues from PC patients without diabetes. Relative expression of miR-30b in serum (B) and urine (C) from PC patients with or without diabetes. *p < 0.05 and **p < 0.01 compared with PC patients without diabetes. qRT-PCR was used to determine the expression of miR-30b.
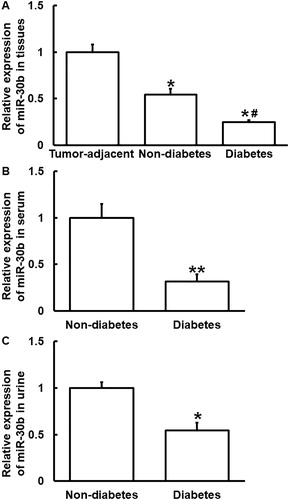
miR-30b can bind with the 3′-UTR seed region of PAI-1 mRNA to regulate its expression
To predict genes that might regulate PAI-1, miRanda (http://34.236.212.39/microrna/home.do) was used. The data showed that miR-30b was a potential gene that might regulate PAI-1, and UGUUUAC was the potential seed region of miR-30b (. To identify the interaction between miR-30b and the 3′-UTR of PAI-1 mRNA, dual luciferase reporter assay was performed. The luminescence value of 293 T cells co-transfected with agomiR-30b and pMIR-REPORT-WT luciferase reporter plasmids was significantly lower than that in the negative control group (p < 0.05). By contrast, the luminescence value of cells co-transfected with agomiR-30b and pMIR-REPORT-mutant luciferase reporter plasmids was not significantly different from that in the negative control group (p > 0.05) (. The results indicate that miR-30b can bind with the 3′-UTR seed region of PAI-1 mRNA to regulate its expression.
Figure 5. Identification of interaction between miR-30b and PAI-1 mRNA. (A) Bioinformatics prediction (miRanda) of genes that might regulate PAI-1. (B) Dual luciferase reporter assay. Plasmids (0.8 μg) with wild-type or mutant 3′-UTR sequences were co-transfected with agomiR-30b into 293 T cells. For control, 293 T cells were transfected with agomiR-negative control (NC) according to the manufacturer’s manual, and luminescence intensity was measured using GloMax 20/20 luminometer (Promega). Renilla luminescence activity was used as internal reference. **p < 0.01 compared with NC group.
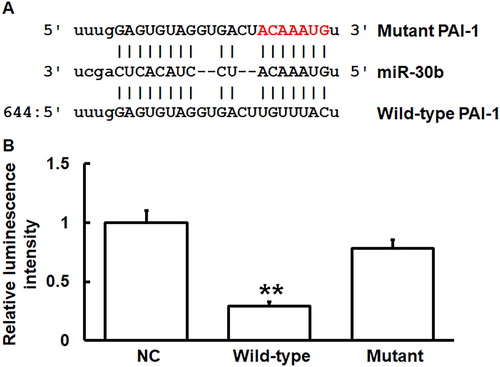
miR-30b overexpression inhibits the expression of PAI-1, alters the microenvironment surrounding PC cells, and promotes the proliferation of islet β-cells
To examine how miR-30b regulates the expression of PAI-1 and affects the proliferation of islet β-cells (INS-1), PANC-1 cells were transfected with agomiR-30b or agomiR-NC and their culture supernatants were used to stimulate INS-1 cells. qRT-PCR showed that the expression of miR-30b in PANC-1 cells transfected with agomiR-30b was significantly higher than that in the agomiR-NC group (p < 0.01) (. In addition, the expression of PAI-1 mRNA and protein in PANC-1 cells transfected with agomiR-30b was significantly lower than that in agomiR-NC group (p < 0.05) (). MTT assay showed that the proliferation of INS-1 cells treated with supernatant of PANC-1 cells that were transfected with agomiR-30b was significantly higher than that in the agomiR-NC group (p < 0.05 at 72 h) (. The results suggest that miR-30b overexpression inhibits the expression of PAI-1, alters the microenvironment surrounding PC cells, and promotes the proliferation of islet β-cells.
Figure 6. Effect of miR-30b overexpression on the expression of PAI-1 in PC cells (PANC-1) and the proliferation of islet β-cells (INS-1). (A) Expression of miR-30b in PANC-1 cells after transfection with agomiR-NC or agomiR-30b. **p < 0.01 compared with agomiR-NC group. Expression of PAI-1 mRNA (B) and protein (C) in PANC-1 cells after transfection with agomiR-NC or agomiR-30b. *p < 0.05 compared with agomiR-NC group. (D) Proliferation of INS-1 cells after stimulation by culture supernatant of PANC-1 cells transfected with agomiR-NC or agomiR-30b. MTT assay was used to determine cell proliferation. *p < 0.05 compared with agomiR-NC group.
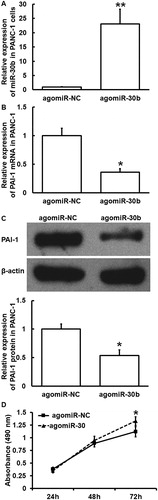
Conclusions
The present study suggests that reduced expression of miR-30b in PC patients with diabetes promotes the increased expression of PAI-1. By affecting the proliferation of islet β-cells, miR-30b and PAI-1 are involved in the pathological process of diabetes mellitus induced by PC.
Author contributions
WY and XL contributed to the design of the study. WY, HZ, JD, CL and ZZ performed the experiments. WY, CL and ZZ analyzed the data. WY, HZ, JD and XL interpreted results and prepared the manuscript. The final version of the manuscript has been read and approved by all authors.
Ethical approval and consent to participate
All procedures performed in the present study were approved by the Ethics Committee of Jinzhou Medical University. Written informed consent was obtained from all patients or their families.
Consent for publication
Written informed consents for publication of any associated data and accompanying images were obtained from all patients or their parents, guardians or next of kin.
Acknowledgements
The authors wish to thank their department and research team for their help and dedication.
Disclosure statement
The authors declare that they have no competing interests.
Additional information
Funding
References
- Siegel RL, Miller KD, Jemal A. Cancer statistics, 2019. CA Cancer J Clin. 2019;69(1):7–34.
- Siegel R, Naishadham D, Jemal A. Cancer statistics, 2012. CA Cancer J Clin. 2012;62(1):10–29.
- Chen W, Zheng R, Zeng H, et al. The updated incidences and mortalities of major cancers in China, 2011. Chin J Cancer. 2015;34(3):502–507.
- Andersen DK, Korc M, Petersen GM, et al. Diabetes, pancreatogenic diabetes, and pancreatic cancer. Diabetes 2017;66(5):1103–1110.
- Korc M. Pathogenesis of pancreatic cancer-related diabetes mellitus: Quo vadis? Pancreas. 2019;48(5):594–597.
- Basso D, Greco E, Fogar P, et al. Pancreatic cancer-derived S-100A8 N-terminal peptide: a diabetes cause? Clin Chim Acta. 2006;372(1–2):120–128.
- Basso D, Greco E, Fogar P, et al. Pancreatic cancer-associated diabetes mellitus: an open field for proteomic applications. Clin Chim Acta. 2005;357(2):184–189.
- Valerio A, Basso D, Fogar P, et al. Maldi-TOF analysis of portal sera of pancreatic cancer patients: identification of diabetogenic and antidiabetogenic peptides. Clin Chim Acta. 2004;343(1–2):119–127.
- Wang J, Yuan Y, Cai R, et al. Association between plasma levels of PAI-1, tPA/PAI-1 molar ratio, and mild cognitive impairment in Chinese patients with type 2 diabetes mellitus. J Alzheimers Dis. 2018;63(2):835–845.
- Pieters M, Barnard SA, Loots DT, et al. The effects of residual platelets in plasma on plasminogen activator inhibitor-1 and plasminogen activator inhibitor-1-related assays. PloS One. 2017 [cited 2019 Sep 18];12(2):e0171271.
- Deng ZY, Shan WG, Wang SF, et al. Effects of astaxanthin on blood coagulation, fibrinolysis and platelet aggregation in hyperlipidemic rats. Pharm Biol. 2017;55(1):663–672.
- Inoue K. [MicroRNA function in animal development]. Tanpakushitsu kakusan koso Protein, nucleic acid, enzyme. 2007;52(3):197–204.
- Looney AM, Ahearne CE, Hallberg B, et al. Downstream mRNA target analysis in neonatal hypoxic-ischaemic encephalopathy identifies novel marker of severe injury: a proof of concept paper. Mol Neurobiol. 2017;54(10):8420–8428.
- Ponnusamy V, Kapellou O, Yip E, et al. A study of microRNAs from dried blood spots in newborns after perinatal asphyxia: a simple and feasible biosampling method. Pediatr Res. 2016;79(5):799–805.
- Looney AM, Walsh BH, Moloney G, et al. Downregulation of umbilical cord blood levels of miR-374a in neonatal hypoxic ischemic encephalopathy. J Pediatr. 2015;167(2):269–273.e2.
- Zhu ED, Li N, Li BS, et al. miR-30b, down-regulated in gastric cancer, promotes apoptosis and suppresses tumor growth by targeting plasminogen activator inhibitor-1. PloS One. 2014 [cited 2019 Sep 18];9(8):e106049.
- Livak KJ, Schmittgen TD. Analysis of relative gene expression data using real-time quantitative PCR and the 2–ΔΔCT method. Methods. 2001;25(4):402–408.
- Kwaan HC, Lindholm PF. Fibrin and fibrinolysis in cancer. Semin Thromb Hemost. 2019;45(4):413–422.
- Conn EM, Botkjaer KA, Kupriyanova TA, et al. Comparative analysis of metastasis variants derived from human prostate carcinoma cells: roles in intravasation of VEGF-mediated angiogenesis and uPA-mediated invasion. Am J Pathol. 2009;175(4):1638–1652.
- Wei X, Li S, He J, et al. Tumor-secreted PAI-1 promotes breast cancer metastasis via the induction of adipocyte-derived collagen remodeling. Cell Commun Signal. 2019 [cited 2019 Sep 18];17(1):58.
- Gerstein ES, Gritsaenko EV, Shcherbakov ME, et al. [Vascular endothelial growth factor and plasminogen activators in endometrial carcinoma and hyperplasia]. Vopr Onkol. 2003;49(6):725–729.
- Hansen S, Overgaard J, Rose C, et al. Independent prognostic value of angiogenesis and the level of plasminogen activator inhibitor type 1 in breast cancer patients. Br J Cancer. 2003;88(1):102–108.
- Andreasen PA. PAI-1 - a potential therapeutic target in cancer. Curr Drug Targets. 2007;8(9):1030–1041.
- Durand MK, Bodker JS, Christensen A, et al. Plasminogen activator inhibitor-I and tumour growth, invasion, and metastasis. Thromb Haemost. 2004;91(03):438–449.
- Li X, Gao Y, Meng Z, et al. Regulatory role of microRNA-30b and plasminogen activator inhibitor-1 in the pathogenesis of cognitive impairment. Exp Ther Med. 2016;11(5):1993–1998.
- Liz J, Esteller M. lncRNAs and microRNAs with a role in cancer development. Biochim Biophys Acta. 2016;1859(1):169–176.
- Varshney J, Subramanian S. MicroRNAs as potential target in human bone and soft tissue sarcoma therapeutics. Front Mol Biosci. 2015 [cited 2019 Sep 18];2:31.
- Ouzounova M, Vuong T, Ancey PB, et al. MicroRNA miR-30 family regulates non-attachment growth of breast cancer cells. BMC Genomics. 2013 [cited 2019 Sep 18];14(1):139.
- Donate PB, Fornari TA, Macedo C, et al. T cell post-transcriptional miRNA-mRNA interaction networks identify targets associated with susceptibility/resistance to collagen-induced arthritis. PloS One. 2013 [cited 2019 Sep 18];8(1):e54803.
- He J, Jiang S, Li FL, et al. MicroRNA-30b-5p is involved in the regulation of cardiac hypertrophy by targeting CaMKIIdelta. J Investig Med. 2013;61(3):604–612.
- Braun J, Hoang-Vu C, Dralle H, et al. Downregulation of microRNAs directs the EMT and invasive potential of anaplastic thyroid carcinomas. Oncogene 2010;29(29):4237–4244.
- Yu F, Deng H, Yao H, et al. Mir-30 reduction maintains self-renewal and inhibits apoptosis in breast tumor-initiating cells. Oncogene. 2010;29(29):4194–4204.
- Le Guillou S, Sdassi N, Laubier J, et al. Overexpression of miR-30b in the developing mouse mammary gland causes a lactation defect and delays involution. PloS One. 2012 [cited 2019 Sep 18];7(9):e45727.
- Altmae S, Martinez-Conejero JA, Esteban FJ, et al. MicroRNAs miR-30b, miR-30d, and miR-494 regulate human endometrial receptivity. Reprod Sci. 2013;20(3):308–317.
- Song PP, Hu Y, Liu CM, et al. Embryonic ectoderm development protein is regulated by microRNAs in human neural tube defects. Am J Obstetr Gynecol. 2011 [cited 2019 Sep 18];204(6):544.e9-17.
- Gaziel-Sovran A, Segura MF, Di Micco R, et al. miR-30b/30d regulation of GalNAc transferases enhances invasion and immunosuppression during metastasis. Cancer Cell. 2011;20(1):104–118.