Abstract
The study aimed to identify key genes involved in cerebral vasospasm (CVS) after subarachnoid haemorrhage (SAH). GSE46696 of basilar arteries of SAH mice and normal controls were downloaded from the Gene Expression Omnibus (GEO). Integrated microarray analysis was performed to identify differentially expressed genes (DEGs). GO and KEGG pathway enrichment analyses of DEGs were performed with ClueGO. The protein–protein interaction (PPI) networks were constructed using Cytoscape software. A total of 4103 DEGs were identified; among them, 254 DEGs (63 up-regulated genes and 191 down-regulated genes) showed significant differences at p < 0.05. GO analysis showed that the identified DEGs were over-represented in 16 GO terms. KEGG pathway analysis showed that pathways in immune inflammation were significantly enriched pathways for DEGs. DEGs with relatively frequent interactions after CVS secondary to SAH included MIKI, Cmpk2, TIr3, Psmb9, Ddx58, Lgals9, Ifi44, Stat2, Rsad2, Oas2, Usp18, H28, Irf7 and als3bp. Multiple genes were involved in the regulation of the immune response in the pathogenesis of SAH, including Ripk3, Ifih1, IL10, Reg3g, SIc11a1, NF-κB, Tlr7, Parp9, Rab7b, Dhx58, Gpx2, Zbp1, Aim2, Rsad2, Lgals9, TLR4, Adar, Zc3hav1, KIrk1, Irf7, IL-1β, Trafd1, Ddx58 and Trim5. Our findings revealed the gene expression profiles of the cerebral arteries in SAH mouse models, and speculated that DHx58 gene plays an important role in the immune response through regulating inflammatory cytokines expression, which may be a potential target in the treatment of CVS after SAH. Our finding provided new clues for understanding the mechanism of SAH.
Introduction
Spontaneous subarachnoid haemorrhage (SAH) accounts for approximately 5% of stroke (containing cerebral haemorrhage and cerebral ischaemia). It has been well recognized that the most common cause for SAH is the rupture of intracranial aneurysms, accounting for 75%-85%. SAH is characterized by high disability rate and high mortality in the clinic [Citation1]. Cerebral vasospasm (CVS) is considered to be one of the most important cerebrovascular changes in early brain injury (EBI) after SAH. CVS usually occurs within two weeks after SAH and has a high incidence of 70%, which is characterized by local cerebral ischaemia and infarction or changes in neurological function of the brain caused by delayed or long-term contraction of cerebral arteries [Citation2]. CVS is the main cause for poor prognosis in SAH patients [Citation3]. Current studies suggest that vascular stenosis, hemodynamically mechanical stimulation, increased intracranial pressure and oxidative stress of vascular wall caused by various factors such as atherosclerotic plaque are all associated with the occurrence of CVS. However, the precise pathological mechanism of CVS is still unclear. Previous studies have shown that during SAH combined with CVS, immune-inflammatory factors can present waterfall-like release effect, induce dysfunction of vascular endothelial cells, and then promote the occurrence of CVS [Citation4]. With the deep understanding of immune-inflammatory factors in the study of CVS, the important role of immune-inflammatory factors in the formation, occurrence and development of CVS is observed. Highly expressed inflammatory factors that are consistent with the onset time of CVS include interleukin-6 (IL-6), intercellular adhesion molecule-1 (ICAM-1) and interleukin-1α (IL-1α), which indicated that the occurrence of CVS is associated with inflammatory response [Citation5]. Several clinical trials have shown that the release of inflammatory factors associated with inflammatory activation after SAH is very common, and is strongly associated with the progression of disease severity. The inflammatory response runs through SAH. The heavier the early inflammatory response, the worse the prognosis, and the heavier the vasospasm. Anti-inflammatory drugs and appropriate doses of hormones can improve prognosis and mitigate CVS [Citation6].
Therefore, it is very important for the management of SAH patients to further explore the pathogenesis of CVS, identify the role of inflammatory factors in CVS and effectively prevent CVS. This study analysed the genes of basilar artery in SAH model mice and normal mice by integrated microarray analysis, searched for key target genes, explored the function of immune-inflammatory factors in the occurrence and development of disease, and aimed to further reveal the potential pathogenesis of CVS.
Materials and methods
Microarray expression profiling in GEO
Microarray datasets (GSE46696) of basilar arteries of SAH mice and normal controls were downloaded from the Gene Expression Omnibus (GEO, http://www.ncbi.nlm.nih.gov/geo). Brain basilar artery specimens from six male mice were included. Microarray analysis was used to analyse gene changes in model group (6 h after SAH) and control group (Sham) ().
Table 1. Specimen numbers.
The affy package was installed in Bioconductor software. GSE46696-RAW data were imported, decompressed and read. After inputting the code, datasets were annotated and normalized. Differentially expressed genes (DEGs) were identified with p < 0.05 and false discovery rate (FDR) < 0.05. In accordance with log fold change > 2 or log fold change < −2, up-and down-regulated DEGs were identified.
GEO2R software was used to make a box plot of chip specimens for homogeneity of the specimens. Furthermore, we used Heml 1.0 software to conduct unsupervised hierarchical clustering analysis of specimens in GSE46696.
Bioinformatics analysis
Kyoto Encyclopedia of Genes and Genomes (KEGG) pathway enrichment analysis and Gene Ontology (GO) enrichment analysis were performed with ClueGO (Cytoscape plug-in) to interpret the biological functions of DEGs. The function and pathway of DEGs were screened according to p < 0.05 and the number of enriched genes per Term.
Ethics statement
The study was approved by the Animal research Ethics Committee of Tianjin Medical University (License Number: IRB2012-028-02).
Establishment of mouse SAH model
Twelve male C57BL mice (10-12 weeks), weighing 20-23 g were purchased from the Department of Experimental Animal Science of Tianjin Medical University, and were randomly divided into SAH model and sham groups, with 6 mice in each group. Mice were anesthetized by intramuscular injection of ketamine (40 mg/kg). On day 0, 0.5 mL of cerebrospinal fluid was aspirated percutaneously from the cisterna magna using a 23-gauge butterfly needle, and then the unheparinized autologous arterial blood obtained from caudal artery was injected into the cisterna magna over 1 min through an intravenous pump. Mice were maintained in a prone position with head-down tilt of 30° for 30 min. No blood clot was found in the syringe during this process. On day 3, after the first haemorrhage, heparin (400 U/kg) was injected into mice, and mice were euthanatized by an intravenous overdose of sodium pentobarbital (120 mg/kg) and exsanguinated from the carotid artery. After exposure of the brain, the formation of blood clots on the surface of the pons and basilar artery was observed in the SAH mice model.
Brain tissue sample preparation
The brain tissue of mice was separated after perfusion with ice-cold phosphate buffered saline (PBS), and transferred to ice-cold PBS. Brain tissues were then ground with a syringe core, filtered through a 200-mesh filter. After adding PBS to 40 mL, brain tissues were centrifuged at 252 g for 10 min. Cells were resuspended in 5 mL of 70% percoll (Sigma), and transfered to a 15 mL centrifuge tube, 5 mL of 30% percoll were then added slowly to the centrifuge tube. After centrifugation at 400 g for 20–30min, the upper residue was aspirated, 1 mL of the upper and bottom layers was aspirated, transferred into another clean centrifuge tube, and washed 1–2 times with PBS, and once with DMEM.
Western blotting analysis
Three days after establishment of the mouse SAH model, the expression levels of inflammatory cytokines, IL-1β, TNF-α and TLR4/NF-κB inflammatory signalling pathway-related proteins (TLR4, NF-κB) in brain tissues of mice in each group were detected by western blotting analysis. Equal amounts of the protein samples were subjected to 10% sodium dodecyl sulphate polyacrylamide gel electrophoresis (SDS-PAGE), separating the proteins at 80 V for 30 min and then raising the voltage to 120 V for 60 min. After electrophoresis, the proteins were transferred onto a 0.45-μm-thick nitrocellulose membrane, which was then blocked with skim milk for 2 h at room temperature. Subsequently, the membranes were incubated with diluted primary antibodies at 4 °C overnight: anti-IL-1β (1:1000, ab9722, Abcam, UK), anti-NF-κB P65 (1:1000, 8242S, CST, USA), anti-TNF-α (1:1000, 7124, cell signal, USA), anti-TLR4 (1:100, 48-2300, ThermoFisher, USA) and anti-β-actin (1:2000, 4970S, CST, USA). The blot bands were quantitated by ImageJ software (National Institutes of Health, USA), and the data were normalized to β-actin.
Statistical processing
Data were analysed with SPSS 18.0 software. Measurement data were expressed as mean values with standard deviation (± SD). The difference between groups was compared using t-test. Differences were considered statistically significant a level of p < 0.05.
Results and discussion
SAH is a common haemorrhagic cerebrovascular disease in clinical practice. The condition of SAH is often dangerous or even life-threatening. CVS is an important cause for poor prognosis, high mortality and disability after SAH. A previous study [Citation7] has confirmed that the occurrence of CVS is the secondary immune-inflammatory response of the cerebrovascular wall and plays a promoting role in the course of the disease. The release of a large number of inflammatory factors can trigger the inflammatory response and vascular oxidative stress, and also activate the migration of leukocytes to the damaged areas of brain tissue in the early stage. After infiltration of the cerebral artery wall, various stimulating factors may cause diastolic and systolic dysfunction of cerebrovascular endothelial cells, thereby resulting in CVS [Citation8]. The effect of immune-inflammatory factors on the pathological process of CVS secondary to SAH has been gradually proven by researchers, and some immune-inflammatory factors have been reported. A study has shown that after administration of anti-inflammatory drugs in a rat SAH model, CVS in rats was significantly relieved, indicating that the balance of oxidants and antioxidants in the brain was broken after SAH, while anti-inflammatory drugs reduced the occurrence of oxidative stress disorder, inhibited the release of pro-inflammatory factors and prevented the release of endogenous antioxidant factors, thereby exerting the inhibitory effect on CVS [Citation9]. In SAH rat models, the heavier the CVS was, the higher the MCP-1 expression was, and MCP-1 promoted inflammation by recruiting macrophages [Citation10]. IL-6 is an important immune-inflammatory factor, which is also an inflammatory marker for evaluating CVS after SAH. Large amount of IL-6 released after SAH leads to endothelial dysfunction, induces and aggravates CVS [Citation11]. Rasmussen et al. [Citation12] found that the occurrence of CVS in SAH animal models was associated with the increase of tumour necrosis factor-α (TNF-α) level. Inhibition of the TNF-α level rescued the brain injury and alleviated the occurrence of cerebrovascular events [Citation13]. Therefore, TNF-α may be a new intervention target for the prevention and treatment of CVS [Citation14].
Microarray results
A box plot of six chip specimens was made by GEO2R software. The results showed good homogeneity of the six specimens (), indicating that the specimens were well taken. The integrated microarray analysis displayed 4353 DEGs. A total of 4103 DEGs (2132 up-regulated genes and 1971 down-regulated genes) were found with a P-value of less than 0.05. Furthermore, 254 DEGs were identified with p < 0.05, adj. p < 0.05. There were 63 up-regulated genes. Twenty-one genes that were up-regulated by more than one fold included Ldb2, Mas1l, P2rx5, Abcg4, RT1-CE1, RT1-CE4, Rapgef3, Epsti1, Idh2, Adcy5, Trim30c, Col6a6, Csrp2, Shmt2, Trim25, Tspan2, Lipg, Glp2r, Xaf1, Mthfd2 and Cacna1d. One gene that was up-regulated by more than two folds was Trex1. There were 191 down-regulated genes, including 109 genes that were down-regulated by more than one fold. Forty-one genes that were down-regulated by more than two folds included Stat2, Lgals3bp, Lgals9, Isg20, Vcan, Ifi44, Tmem163, Ifit2, Irf7, Slc2a4, Mitd1, Parp12, Ifih1, Tdrd7, Slamf8, Oas1k, F10, Ddx58, Ascc3, Ifit3, Isg15, Cbx4, Tle4, MGC108823, Slfn13, Tcirg1, Trim5, Nmt2, Slco2b1, Znfx1, Rnf114, Apol3, Fndc3a, Mx1 and RGD1563091. Seven genes were down-regulated by more than three folds. Of them, Apol9a, Rsad2, RGD1305184, Iqsec1, Zbp1, Cmpk2 and Ifi47 were down-regulated by 3.89-fold, 3.61-fold, 3.48-fold, 3.46-fold, 3.37-fold, 3.31-fold and 3.25-fold, respectively. The unsupervised hierarchical clustering analysis of six specimens in GSE46696 was conducted with Heml 1.0 software, including up- and down-regulated genes and clustering, as shown in , red indicated up-regulation and green indicated down-regulation.
GO functional enrichment analysis
GO enrichment analysis of 4130 DEGs was performed by using the online software Cytoscape (ClueGO plug-in), 16 functional annotation clustering results were obtained. The over-represented functions consisted of defense response, response to virus, response to interferon-beta, positive regulation of cation transmembrane transport, innate immune response, regulation of antigen processing and presentation, cytokine production, response to other organism, response to cytokine, response to type I interferon, negative regulation of binding, positive regulation of NF-κB transcription factor activity, positive regulation of endopeptidase activity, positive regulation of JAK-STAT cascade and response to interferon-alpha (). The ClueGO plug-in in Cytoscape software was utilized to make a pie chart to show the related biological functions of 4103 genes. These functions may play a role in the pathological process of CVS secondary to SAH.
KEGG pathway enrichment analysis
KEGG pathway enrichment analysis of DEGs was performed with the ClueGO plug-in of the online software Cytoscape. Screening results with p < 0.05 found that 4130 DEGs participated in eight potential signalling pathways, including influenza A, ABC transporters, Toll-like receptor signalling pathway, toxoplasmosis, TNF signalling pathway, cytosolic DNA-sensing pathway, RIG-I-like receptor signalling pathway and proteasome. These signalling pathways may be associated with the pathological process of CVS secondary to SAH. The KEGG pathway enrichment analysis results of DEGs are shown in . KEGG pathway enrichment analysis indicates that further investigation of some signalling pathways can provide some guidance for the study of the occurrence and development of cerebral arterial spasm after SAH.
A previous study has suggested that the Akt/GSK3β signalling pathway plays a role in the early stage of SAH by regulating endothelial cell apoptosis [Citation15]. An animal model study of the pathological process of SAH found that insulin-like growth factor-1 remarkably increased pAkt expression, and then reduced the percentage of apoptotic cells after SAH, indicating that insulin-like growth factor-1 played a protective role by activating the PI3K signalling pathway, and administration of Ly294002 (PI3K pathway inhibitor) notably induced the increase of apoptotic cells[Citation16]. The Akt/GSK3β signalling pathway plays a role in reducing brain nerve injury after SAH [Citation17]. In the PI3K-AKT signalling pathway, MSR1 can inhibit the progress of chronic myelogenous leukaemia by regulating PI3K-AKT signalling pathway and β-catenin expression [Citation18]. In 2000, Inage et al. [Citation19] found that glutamate transporters play a role in the pathogenesis of neonatal SAH by regulating ABC signalling transduction pathway. TLR4, as a member of the TLR family and plays an important role in innate immunity. The TLR4 expression was increased in the arterial wall in an animal model of SAH [Citation20]. TLR4 can activate the NF-κB and MAPK signalling pathways through MyD88 signal transmission in the injured brain caused by CVS secondary to SAH [Citation21].
Gene interaction analysis
Gene–gene interaction analysis of DEGs was performed using Cytoscape software. As shown in , more gene interaction lines in the gene–gene interaction network diagram represented greater interaction frequency in biological process, and greater importance of the gene. DEGs with relatively frequent interactions after CVS secondary to SAH included MIKI, Cmpk2, TIr3, Psmb9, Ddx58, Lgals9, Ifi44, Stat2, Rsad2, Oas2, Usp18, H28, Irf7 and als3bp.
Analysis of genes involved in immune response regulation
Functional enrichment analysis of DEGs revealed that genes involved in immune response regulation played a role in the pathological process of SAH with CVS. Therefore, we separately analysed the genes involved in immune response. The network diagram () showed that multiple genes were involved in the regulation of immune response in the pathogenesis of SAH, including Ripk3, Ifih1, IL10, Reg3g, SIc11a1, NF-κB, Tlr7, Parp9, Rab7b, Dhx58, Gpx2, Zbp1, Aim2, Rsad2, Lgals9, TLR4, Adar, Zc3hav1, KIrk1, Irf7, IL-1β, Trafd1, Ddx58 and Trim5. IL-10 is an anti-inflammatory cytokine synthesized by immunocompetent cells. A comparative study of 30 rabbits in the SAH group and SAH + IL-10 intervention group (4 μg/kg, intraperitoneal injection) showed that IL-10 markedly alleviated CVS induced by SAH. Our results demonstrated that IL-10 expression was down-regulated after SAH. Therefore, up-regulation of IL-10 expression may be a potential therapy for treating CVS. The DHx58 gene belongs to retinoic acid-inducible gene 1 like receptor family, and plays a role in initiating innate immunity and helping the body clear invasive pathogens [Citation22]. The abnormal expression of the DHx58 gene in cerebrovascular tissue was verified in viral infection models of Atlantic cod, which proved that DHx58 was strongly associated with inflammatory factors in regulating immune response [Citation23]. DHx58 is also a high-frequency gene in the gene–gene interaction network and a gene related to immune response regulation. Therefore, we speculate that DHx58 may be a potential target in the treatment of CVS after SAH.
Western blotting analysis
A previous study confirmed that after SAH, the body's immuno-inflammatory factors were released in a waterfall-like release effect, and a lot of inflammatory cells infiltrated the arterial wall, including neutrophils, macrophages and T cells, and the levels of inflammatory cytokines, tumour necrosis factor-alpha (TNF-α), interleukin-1 (IL-1, IL-1α, IL-1β), interleukin-6 (IL-6) and Toll-like receptor-4 (TLR-4) were increased [Citation24]. During CVS, inflammatory cells release IL-1β and TNF-α, which in turn lead to the occurrence and aggravation of secondary inflammatory response, thus triggering the release of various immuno-inflammatory factors in the body. This release is a cascade reaction, a waterfall-like burst, and further activates the migration of leukocytes to the damaged parts of the body (including brain tissue), resulting in the infiltration of inflammatory factors in the vascular wall and aggravation of vasospasm. Using integrated microarray analysis of specimens of CVS secondary to SAH, four immuno-inflammatory factors with relatively significant changes were obtained through GO functional enrichment analysis, KEGG pathway enrichment analysis and gene–gene interaction analysis, including IL-1β, TNF-a, NF-kB and TLR4.
The expression levels of inflammatory cytokines, IL-1β, TNF-α and TLR4/NF-κB inflammatory signalling pathway-related proteins, TLR4, NF-κB in brain tissues were detected by western blotting analysis. The results showed that on day 3 after model establishment, the expression levels of IL-1β, TNF-α, NF-κB and TLR4 in brain tissues of SAH mice were significantly increased as compared to those in the sham group (all p < 0.05, ) .
Figure 7. Western blotting analysis of IL-1β, TNF-α, NF-κB and TLR4 in brain tissues of SAH mice. Three days after model establishment, the expression levels of inflammatory cytokines IL-1β, TNF-α and TLR4/NF-κB inflammatory signalling pathway-related proteins, NF-κB, TLR4 were statistically higher in the brain tissues of mice in the SAH model group than in the sham group (all p < 0.05).
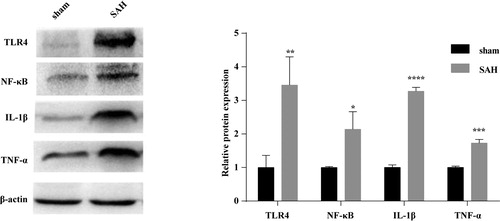
A study used Enzyme-linked immunosorbent assay (ELISA) to detect IL-1β expression in different periods of SAH models, and found that the expression level of IL-1B was associated with the duration of CVS [Citation25]. The higher the IL-1β expression level was, the earlier the occurrence of CVS and the longer the duration was [Citation26]. The expression levels of IL-1β, IL-6 and TNF-a in SAH patients were correlated with the prognosis of SAH; the earlier the release time of endogenous inflammatory factors was, the higher the release level and the worse the prognosis was [Citation27, Citation28]. Results from real-time PCR, western blot assay, immunohistochemistry and ELISA in SAH models showed that TLR4/NF-xo3BA signalling pathway was activated in early SAH, triggered inflammation and promoted CVS, and inhibition of TLR4/NF-xo3BA signalling pathway alleviated the degree of CVS [Citation29]. The TLR4/NF-kB signalling pathway played an important role in the pathological process of SAH with CVS; targeted control of inflammatory factors expression effectively alleviated the occurrence of CVS secondary to SAH, thereby remarkably improving the prognosis of patients [Citation30].
Inflammatory factors, as an indispensable part of immune response, are involved in many processes of CVS secondary to SAH, involving multiple pathways and the functional regulation of related genes. Immuno-inflammatory response is a complex network, and the efficacy of treatment of CVS by only inhibiting the release of pro-inflammatory cytokines is limited. In this study, we identified DEGs from specimens using integrated microarray analysis, analysed the enriched GO terms and KEGG pathways of DEGs, and predicted that the DHx58 gene plays an important role in immune response through regulating the expression of inflammatory cytokines. At present, there are few reports concerning the effect of DHx58 gene on regulating inflammatory factors. It is assumed that blocking the expression of upstream genes of these inflammatory factors, while inhibiting the release of pro-inflammatory mediators can play a combined effect to effectively control CVS caused by SAH. This is not only a new treatment strategy, but also a new research idea for the effective prevention and treatment of CVS secondary to SAH and the improvement of prognosis.
Conclusions
In conclusion, our results revealed the gene expression profiles of the cerebral arteries in SAH mouse models, and DEGs with relatively frequent interactions after CVS secondary to SAH included MIKI, Cmpk2, TIr3, Psmb9, Ddx58, Lgals9, Ifi44, Stat2, Rsad2, Oas2, Usp18, H28, Irf7 and als3bp. Multiple genes were identified to be involved in the regulation of the immune response in the pathogenesis of SAH, including Ripk3, Ifih1, IL10, Reg3g, SIc11a1, NF-κB, Tlr7, Parp9, Rab7b, Dhx58, Gpx2, Zbp1, Aim2, Rsad2, Lgals9, TLR4, Adar, Zc3hav1, KIrk1, Irf7, IL-1β, Trafd1, Ddx58 and Trim5. The DHx58 gene may be a potential target in the treatment of CVS after SAH, which needs to be further validated by extensive experiments.
Author contributions
YW and YT designed the study, checked the data, and prepared the manuscript; ZH, YF, YL, MY, SZ, HM, ZW, JZ performed data collection and statistical analysis. All authors have approved the final version of the manuscript to be published.
Disclosure statement
No potential conflict of interest was reported by the author(s).
Additional information
Funding
References
- Price WH, Steers AJ, Wilson J. Subarachnoid hemorrhage and Klinefelter’s syndrome. Lancet. 1982;320(8294):380. [cited 2020 Mar 20]
- Li K, Barras CD, Chandra RV, et al. A review of the management of cerebral vasospasm after aneurysmal subarachnoid hemorrhage. World Neurosurg. 2019;126:513–527.
- Penn DL, Witte SR, Komotar RJ Jr., et al. Pathological mechanisms underlying aneurysmal subarachnoid haemorrhage and vasospasm. J Clin Neurosci. 2015;22(1):1–5.
- Chaudhry SR, Kahlert UD, Kinfe TM, et al. Elevated systemic IL-10 levels indicate immunodepression leading to nosocomial infections after aneurysmal subarachnoid hemorrhage (SAH) in patients. IJMS. 2020;21(5):1569. [cited 2020 Mar 20]
- Akkaya E, Evran Ş, Çalış F, et al. Effects of intrathecal verapamil on cerebral vasospasm in experimental rat study. World Neurosurg. 2019;127:e1104–e1111.
- Huang Q, Wang G, Hu YL, et al. Study on the expression and mechanism of inflammatory factors in the brain of rats with cerebral vasospasm. Eur Rev Med Pharmacol Sci. 2017;21(12):2887–2894.
- Mohney N, Williamson CA, Rothman E, et al. A propensity score analysis of the impact of dexamethasone use on delayed cerebral ischemia and poor functional outcomes after subarachnoid hemorrhage. World Neurosurg. 2018;109:e655–e661.
- de Oliveira Manoel AL, Macdonald RL. Neuroinflammation as a target for intervention in subarachnoid hemorrhage. Front Neurol. 2018;9(292)[cited 2020 Mar 20]
- Vadokas G, Koehler S, Weiland J, et al. Early antiinflammatory therapy attenuates brain damage after SAH in rats. Transl Neurosci. 2019;10:104–111.
- Chou SH. Inflammation, cerebral vasospasm, and brain injury in subarachnoid hemorrhage-a shifting paradigm and a new beginning. Crit Care Med. 2018;46(11):1883–1885.
- Peeyush Kumar T, McBride DW, Dash PK, et al. Endothelial cell dysfunction and injury in subarachnoid hemorrhage. Mol Neurobiol. 2019;56(3):1992–2006.
- Wu LY, Ye ZN, Zhuang Z, et al. Biochanin a reduces inflammatory injury and neuronal apoptosis following subarachnoid hemorrhage via suppression of the TLRs/TIRAP/MyD88/NF-κB pathway. Behav Neurol. 2018;2018:1960106–1962020. [cited Mar 20]
- Fan Y, Yan G, Liu F, et al. Potential role of poly (ADP-ribose) polymerase in delayed cerebral vasospasm following subarachnoid hemorrhage in rats. Exp Ther Med. 2019;17(2):1290–1299.
- Gris T, Laplante P, Thebault P, Canadian Critical Care Translational Biology Group, et al. Innate immunity activation in the early brain injury period following subarachnoid hemorrhage. J Neuroinflammation. 2019;16(1):253–2020. ;. [cited Mar 20]
- Rasmussen R, Bache S, Stavngaard T, et al. Plasma levels of IL-6, IL-8, IL-10, ICAM-1, VCAM-1, IFNγ, and TNFα are not associated with delayed cerebral ischemia, cerebral vasospasm, or clinical outcome in patients with subarachnoid hemorrhage. World Neurosurg. 2019;128:e1131–e1136.
- Hao S, Song C, Shang L, et al. Phosphorylation of Akt by SC79 prevents iron accumulation and ameliorates early brain injury in a model of experimental subarachnoid hemorrhage. Molecules. 2016;21(3):325–2020. [cited Mar 20]
- Okada T, Enkhjargal B, Travis ZD, et al. FGF-2 Attenuates neuronal apoptosis via FGFR3/PI3k/Akt signaling pathway after subarachnoid hemorrhage. Mol Neurobiol. 2019;56(12):8203–8219.
- Zhuang Z, Zhao X, Wu Y, et al. The anti-apoptotic effect of PI3K-Akt signaling pathway after subarachnoid hemorrhage in rats. Ann Clin Lab Sci. 2011;41(4):364–372.
- Inage YW, Itoh M, Wada K, et al. Glutamate transporters in neonatal cerebellar subarachnoid hemorrhage. Pediatr Neurol. 2000;23(1):42–48.
- Chen Y, Sullivan C, Peng C, et al. A tumor suppressor function of the Msr1 gene in leukemia stem cells of chronic myeloid leukemia. Blood. 2011;118(2):390–400.
- Hong Y, Shao A, Wang J, et al. Neuroprotective effect of hydrogen-rich saline against neurologic damage and apoptosis in early brain injury following subarachnoid hemorrhage: possible role of the Akt/GSK3β signaling pathway. PLoS One. 2014;9(4):e96212[cited 2020 Mar 20]
- Zhou CH, Wang CX, Xie GB, et al. Fisetin alleviates early brain injury following experimental subarachnoid hemorrhage in rats possibly by suppressing TLR 4/NF-κB signaling pathway. Brain Res. 2015;1629:250–259.
- Ma CX, Yin WN, Cai BW, et al. Toll-like receptor 4/nuclear factor-kappa B signaling detected in brain after early subarachnoid hemorrhage. Chin Med J. 2009;122(13):1575–1581.
- Zhou ML, Wu W, Ding YS, et al. Expression of Toll-like receptor 4 in the basilar artery after experimental subarachnoid hemorrhage in rabbits: a preliminary study. Brain Res. 2007;1173:110–116.
- Lu Y, Zhang XS, Zhang ZH, et al. Peroxiredoxin 2 activates microglia by interacting with Toll-like receptor 4 after subarachnoid hemorrhage. J Neuroinflammation. 2018;15(1):87–2020. [cited Mar 20]
- Fadakar K, Dadkhahfar S, Esmaeili A, et al. The role of Toll-like receptors (TLRs) in stroke. Rev Neurosci. 2014;25(5):699–712.
- Lemos de Matos A, McFadden G, Esteves PJ. Evolution of viral sensing RIG-I-like receptor genes in Leporidae genera Oryctolagus, Sylvilagus, and Lepus. Immunogenetics. 2014;66(1):43–52.
- Chaudhry SR, Stoffel-Wagner B, Kinfe TM, et al. Elevated systemic IL-6 levels in patients with aneurysmal subarachnoid hemorrhage is an unspecific marker for post-SAH complications. IJMS. 2017;18(12)pii:2580. [cited 2020 Mar 20]
- Rise ML, Hall JR, Rise M, et al. Impact of asymptomatic nodavirus carrier state and intraperitoneal viral mimic injection on brain transcript expression in Atlantic cod (Gadus morhua). Physiol Genomics. 2010;42(2):266–280.
- Lv SY, Wu Q, Liu JP, et al. Levels of interleukin-1β, interleukin-18, and tumor necrosis factor-α in cerebrospinal fluid of aneurysmal subarachnoid hemorrhage patients may be predictors of early brain injury and clinical prognosis. World Neurosurg. 2018;111:e362–e373.