Abstract
The calcium-dependent protein kinases (CDPKs, also termed CPKs) are a large, diverse protein family that play significant roles in biotic and abiotic stress responses in plants. However, CDPKs in citrus are relatively unknown, as are their responses to drought stress (abiotic stress) and arbuscular mycorrhizal fungi (AMF) colonization. In this study, the citrus CDPKs were characterized by analyzing the presence of EF-hand and Pkinase domains and searching the citrus genome. Twenty-nine Citrus sinensis CDPKs (CsCDPKs) were identified on at least nine chromosomes in the citrus genome. Three clusters were found on chromosome 4 with three or two CsCDPKs, and 17 CsCDPKs showed syntenic relationships with Arabidopsis thaliana CPKs (AtCPKs). Twenty-nine CsCDPKs were expressed in the roots, and the regulation of the CsCDPKs was not always consistent with the cis-elements in their promoters. CsCDPK1, 2, 5, 6, 7, 8, 9, 13, 17, 18, 19, 21, 24, 25, 26, 27 and 29 were upregulated by AMF colonization, and CsCDPK3, 7 and 28 were downregulated. Drought-repressed expression of CsCDPK10, 11, 12, 15 and 16 induced CsCDPK20 and 22. Furthermore, AMF colonization and drought stress exerted synergistic effects; the expression levels of CsCDPK20 and CsCDPK22 were repressed in W_AMF treatment (well-watered with AMF) but induced in D_AMF treatment (drought stress with AMF). Together, these results provide a basis for further functional studies of the CsCDPK proteins and their responses to AMF and drought stress.
Introduction
Plants use calcium (Ca2+) as a secondary messenger to regulate several biological processes in response to biotic and abiotic stress [Citation1]. The calcium-dependent protein kinases (CDPKs, also termed CPKs) have four domains: the N-terminal variable region, a Ser/Thr kinase catalytic domain, an autoregulatory/autoinhibitory domain and a calmodulin-like domain [Citation2]. They are also well-known plant Ca2+-sensor protein kinases [Citation3]. The N-terminal domain has myristoylation or palmitoylation sites that are associated with subcellular targeting [Citation4]. The protein kinase domain is the catalytic domain with an ATP binding site, followed by the autoinhibitory junction domain [Citation5], and the calmodulin-like domain, which contains EF-hands for binding to calcium [Citation4]. The N- and C-terminal domains vary in length and amino acid composition. The CDPKs are activated by calcium binding at the calmodulin-like domain, resulting in protein conformational changes that drive detachment of the junction domain from the protein kinase domain. Activated CDPKs can mediate Ca2+ signalling by phosphorylating their substrate proteins [Citation3], which play an important role in signal transduction during abiotic stress and microbe interactions [Citation6].
A subset of plant CDPKs exhibit inducible expression patterns that play pivotal roles in the plant response to abiotic stresses such as drought [Citation7–9]. A key plant response to drought stress is stomatal closure to limit water loss, mediated by abscisic acid (ABA) [Citation6]. Twenty Arabidopsis CPKs (AtCPKs) have been detected in the guard cells, with variable expression levels [Citation6]. These genes may have a superposition effect; cpk3, cpk6 and cpk23 single mutants had a weak or no stomatal phenotype on isolated epidermal strips, whereas the cpk3 and cpk6 double mutant and the cpk5, 6, 11 and 23 quadruple mutant had impaired ABA-induced stomatal closure [Citation10]. In addition to AtCPK3, 6, 23, drought-inducing AtCPK4, 11 expressions, and cpk4, 11 displayed reduced stomatal ABA responsiveness for drought responding [Citation11]. The CDPK genes are also involved in the rice drought response. Over-expression of OsCDPK7 or OsCDPK13 enhanced the drought stress tolerance [Citation7]. OsCPK9 transcription is induced by ABA; OsCPK9 over-expression enhanced rice drought tolerance, whereas OsCPK9 RNA interference decreased drought tolerance [Citation12]. CDPKs also function in response to microbe interactions, such as pathogen infection [Citation13–15]. Arabidopsis CPKs are vitally important to the immune signalling pathways; for example, AtCPK1 activates NADPH oxidase, resulting in an oxidative burst [Citation16], and the phosphorylation of Phenylalanine ammonia-lyase results in salicylic acid accumulation [Citation17]. AtCPK4, 5, 6 and 11 phosphorylates a specific subgroup of the WRKY transcription factors (WRKY8, 28 and 48) that are involved in the NLR-dependent restriction of pathogen growth. AtCPK5 also activates RBOH to induce a reactive oxygen species burst [Citation18,Citation19]. Similar to Arabidopsis CPKs, tobacco CDPK-silenced plants displayed a reduced and delayed hypersensitive response to a fungal Avr9 elicitor [Citation20], and rice OsCPK10 expression was induced following treatment with a Magnaporthe grisea elicitor [Citation15]. However, information on the CDPK response to arbuscular mycorrhizal fungi (AMF) colonization is limited, despite its symbiotic effects on drought tolerance [Citation3].
The CDPK family has been characterized in several plants, including Arabidopsis [Citation4], rice [Citation21], wheat [Citation22], Populus [Citation23], cotton [Citation24], Capsicum annuum [Citation25], grape [Citation26], pineapple [Citation27] and banana [Citation28]. CDPKs play important roles in drought stress and microbe interactions and may function in the citrus drought stress response. However, citrus-specific CDPK studies are lacking. In the present study, the sweet orange CDPK gene family members were identified via bioinformatics tools, and their expression patterns in response to drought stress and/or AMF colonization were characterized. This study provides basic information on the protein structures, subfamily divisions, chromosome localization in the citrus genome, and expression patterns of the CDPK genes after drought stress and/or AMF colonization.
Materials and methods
CsCDPK searching and characteristics
The complete genome assembly of citrus (Citrus sinensis ‘Valencia’) was downloaded from the Citrus Genome Database (https://www.citrusgenomedb.org/organism/Citrus/sinensis; C. sinensis genome v2.0). Twenty-eight Arabidopsis thaliana CPK protein sequences (AtCPKs) were obtained from the Arabidopsis Information Resource (TAIR) (https://www.arabidopsis.org/) [Citation29]. Candidate citrus CDPK protein sequences (CsCDPKs) were selected by comparison to the Arabidopsis query sequences via BLASTP. Redundant proteins were manually deleted based on their E-values. The molecular weight (MW) and isoelectric point (pI) of the candidate protein sequences were determined by ExPASy (https://web.expasy.org/compute_pi/) [Citation30]. Conserved EF-hand and Pkinase domains were verified for all potential CDPK proteins using the NCBI Batch CD-Search program (https://www.ncbi.nlm.nih.gov/Structure/bwrpsb/bwrpsb.cgi).
Sequence alignment and phylogenetic analysis
The full-length CDPK protein sequences from Arabidopsis and citrus were aligned via muscle in MEGA version 7.0, with default parameters [Citation31,Citation32]. A neighbour-joining (NJ) tree was also generated with bootstrapping (1000 replicates). The phylogenetic relationships among the citrus CDPKs were estimated from another phylogenetic tree based on the alignment of citrus proteins only.
Conserved motifs and gene structure analysis
Motif analysis was conducted on the MEME website (http://meme-suite.org/tools/meme) to identify conserved motifs with the following optimized parameters: zero or one occurrence per sequence, a maximum of 10 motifs and an optimum motif width between 6 and 50 residues. The default settings were used for all other parameters. The structure of CsCDPKs was determined with TBtools by comparing the coding sequences and the corresponding genomic sequences [Citation33].
The Citrus Generic Feature Format (GFF) file was downloaded from the Citrus Genome Database and used to elucidate the structure information of the CDPK gene. An illustration of the CsCDPK protein motifs, conserved domain, gene structures and a phylogenetic tree was also constructed in TBtools [Citation33].
Chromosomal distribution, gene duplication and collinearity
The chromosome locations of the candidate citrus CDPK genes were analyzed from the GFF information and visualized by TBtools [Citation33]. Gene duplication events of the CsCDPKs and collinearity between the Arabidopsis CPK protein sequences and citrus CDPK protein sequences were investigated by MCScanX [Citation34]. The results were visualized in TBtools [Citation33].
CsCDPK expression in response to AMF colonization and drought
A two-factor, completely randomized block experiment was performed with different watering regimes (i.e. drought stress) and AMF treatments. The protocols of sample preparation and treatment were adapted from our previous researches of Zhang et al. [Citation35] and Luo et al. [Citation36]. The citrus seedlings were well-watered or experienced drought stress and were grown with or without Funneliformis mosseae, resulting in four treatment groups: well-watered with no AMF (W_Non), well-watered with AMF (W_AMF), drought stress with no AMF (D_Non) and drought stress with AMF (D_AMF). Each treatment had three biological replicates of nine pots with three seedlings per pot, and three pots per replicate. The transcriptome data from seedling roots was analyzed as described by Shu et al. [Citation37]. Twelve libraries of the seedling roots were sequenced on the Illumina HiSeq 2000 system, and the low-quality reads (more than 50% of the bases with a quality value ≤5) and reads containing adaptors or more than 10% unknown nucleotides were removed to obtain uncontaminated sequences based on the raw data. The uncontaminated sequences were mapped onto the sweet orange C. sinensis genome (status: draft, version2draft, version2) for annotation. The transcriptome data were uploaded to the NCBI Sequence Read Archive as SRR10413223, SRR10413224, SRR10413225 and SRR10413226 in our previous study [Citation36]. Gene expression was analyzed from the transcriptome data, and the transcript abundance of the CsCDPKs was calculated as the fragments per kilobase of exon model per million mapped reads (FPKM) via the Cufflinks package (cuffdiff version 2.2.1). The FPKM value of the W_Non treatment was used as the control, and heat maps were created in TBtools with the log2 (FPKM + 1) transformed data [Citation33].
Quantitative real-time polymerase chain reaction (qRT-PCR) was performed as in Shu et al. [Citation38] on three independent biological root samples from each treatment, with three technical replications per biological sample. Nine genes were selected for RNA-seq verification, and the qRT-PCR primers are provided in Supplemental Table S1. Relative gene expression was calculated using the 2−△△Ct method described by Livak and Schmittgen [Citation39], with the β-actin gene as the internal reference gene. The measured transcripts were normalized to the relative expression of the W_Non treatment group. Significant differences between the treatments were determined by Duncan’s multiple range tests at p = .05 in SAS (SAS Institute, Inc., Cary, NC, USA). Different letters indicate statistically significant differences.
Cis-acting elements of the CsCDPKs
The 2000 bp sequences upstream of the transcription initiation site of the candidate genes were extracted from the citrus genome sequences. The PlantCARE software (http://bioinformatics.psb.ugent.be/webtools/plantcare/html/) was used to search for cis-acting elements [Citation40], and the results were visualized in TBtools [Citation33].
Results and discussion
Identification and characteristics of the CDPKs in the citrus genome
Twenty-nine CDPK genes were identified in the citrus genome after searching for EF-hand and Pkinase domain sequences. The CsCDPK protein lengths ranged from 377 aa (CsCDPK17) to 910 aa (CsCDPK7), and the pI was usually less than 7 but ranged from 5.05 (CsCDPK14) to 9.23 (CsCDPK12). The molecular weight ranged from 42.50 kDa (CsCDPK17) to 100.61 kDa (CsCDPK7). All of the CsCDPKs were predicted to contain at least one EF-hand, 17 CsCDPKs were predicted to contain myristoylation sites, and 24 CsCDPKs were predicted to contain palmitoylation sites. CsCDPK1 did not have myristoylation and palmitoylation sites (). Plant CDPKs are a multigene family; there are 34 CDPKs in Arabidopsis [Citation41], 29 CDPKs in rice [Citation42], 20 CDPKs in wheat [Citation22], 41 CDPKs in diploid cotton [Citation24], 30 CDPKs in poplar [Citation23], 17 CDPKs in grapevine [Citation43], 44 CDPKs in banana [Citation28] and 17 CDPKs in pineapple [Citation27]. Although the size of citrus genome (380 Mb) (https://www.citrusgenomedb.org/organism/Citrus/sinensis) was larger than the genome of Arabidopsis (125 Mb) (https://www.arabidopsis.org), the total number of citrus CDPK genes was similar to the number of these genes in Arabidopsis.
Table 1. Basic information of CDPK genes identified in Citrus sinensis.
Chromosomal distribution and synteny analysis of the CDPKs in A. thaliana and C. sinensis
The CsCDPKs were mapped on the citrus chromosomes, and 28 of the 29 CsCDPKs were located between chromosomes 1 and 9, and only CsCDPK29 did not map on these nine chromosomes (). The largest number of CsCDPK genes (13 genes) were located on chromosome 4, followed by chromosomes 2 and 6 (three genes each), and then chromosomes 1 and 3 (two genes each). Gene duplication and divergence are important in gene family expansion and in the evolution of novel functions. Three clusters were obtained from the gene cluster analysis, all three were on chromosome 4. Two clusters contained three genes (CsCDPK10, 11, 12 and CsCDPK15, 16, 17), whereas the other cluster had two genes (CsCDPK19 and 20). These clusters likely arose from tandem duplications, suggesting gene family expansion during evolution. There were no segmental duplications in the CsCDPK genes (). To further investigate the phylogenetic patterns of the CsCDPKs, a comparative syntenic map of citrus and A. thaliana was constructed. Seventeen of the 29 CsCDPKs showed syntenic relationships with the AtCPKs, localized on chromosomes 2, 5, 6, 7, 8 and 9 (). Some CsCDPKs corresponded to a single AtCDPK (CsCDPK1, 5, 7, 8, 10, 12, 21, 23, 25 and 26) and some CsCDPKs corresponded to two or three AtCDPKs (CsCDPK2, 3, 4, 6, 22, 27 and 28), suggesting that the 17 CDPKs may have played an important role in the CDPK gene family evolution. These results provide insights that will assist in the prediction of the functions of citrus CDPKs (; Supplemental Table S2).
Figure 1. Chromosomal distribution of the CsCDPK genes (A) and synteny analysis of the CDPKs in Arabidopsis thaliana and Citrus sinensis (B). Note: In (A), chromosome numbers are provided at the top of each chromosome with the approximate size. The CsCDPKs were named CsCDPK1 to CsCDPK29 based on their order on the chromosomes. Red lines indicate the gene clusters with tandem duplications. In (B), grey lines in the background indicate the collinear blocks within the citrus and Arabidopsis genomes, while the blue lines highlight the syntenic CsCDPK gene pairs.
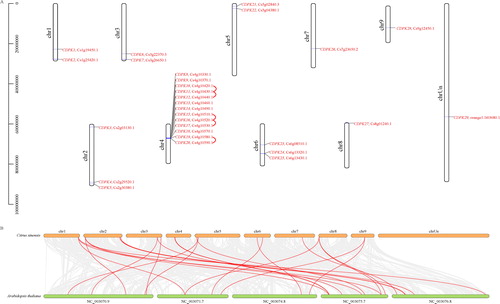
Our results identified 29 CDPKs in citrus and suggested that the variation in CDPK numbers among different plants may be attributed to gene duplications (). For example, in Arabidopsis, AtCPK21, 22, 23, 27 and 31 are tandem duplicates on chromosome 4 [Citation4], which is similar to the citrus genome, where three clusters (CsCDPK10, 11, 12, CsCDPK15, 16, 17 and CsCDPK19, 20) were found on chromosome 4. Seventeen CsCDPKs showed syntenic relationships with AtCDPKs, all of which were localized on chromosomes 2, 5, 6, 7, 8 and 9 (but not chromosome 4) (). Together, these results suggest that fewer CsCDPKs compared to other plants may be due to less gene duplication on the chromosomes.
Phylogenetic, exon–intron structure, conservative domains and motifs analysis of the CsCDPKs
The phylogenetic relationships of citrus and Arabidopsis CDPKs were analyzed by a phylogenetic tree of the protein sequence alignment. As shown in , the citrus and Arabidopsis CDPKs clustered into four major groups. Groups I–IV possessed 30, 16, 13 and 4 members, respectively, and differences were observed between Arabidopsis and citrus. The number of citrus and Arabidopsis CDPKs in groups I and II were nearly equal, and most of the CsCDPKs in group I were together. Group IV had one CsCDPK and three Arabidopsis CDPKs ().
Figure 2. Phylogenetic tree of the CsCDPKs based on an alignment of the citrus and Arabidopsis proteins. Note: The phylogenetic tree was constructed via the neighbour-joining method in MEGA 7.0. Reliability of the predicted tree was tested by bootstrapping with 1000 replicates. Branch lines with different colours represent different CsCDPK groups.
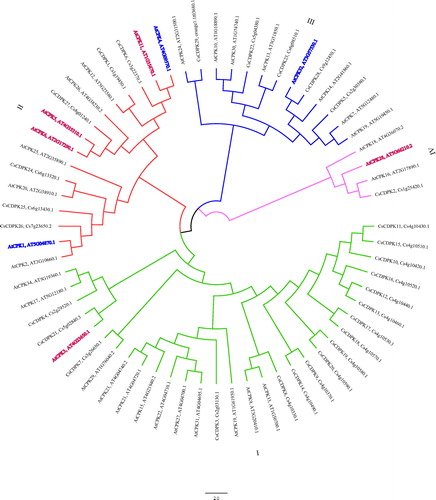
The CsCDPKs in different groups were characterized according to their EF-hand numbers, Pkinase domains and exon–intron structures. Motifs 2, 7, 8 and 10 composed the EF-hand domain, motif 4 composed the Pkinase domain (), and all 29 CsCDPKs had two characteristic domains. The number of introns varied from 4 (CsCDPK17) to 13 (CsCDPK7). Most of the CsCDPKs in group I possessed motifs 2, 7, 8, 10 and 4, but CsCDPK7, 14, 16, 17 and 18 lacked one or more motifs of EF-hand and most lacked the untranslated region (UTR) in the 3′ ends. Nearly all of the CsCDPKs in groups II and III contained 10 motifs, and the differences were in the exon–intron structure. Group IV contained only one member, CsCDPK2, which lacked motif 10 ().
Figure 3. Phylogenetic tree of the deduced CsCDPK proteins associated with the motif and exon–intron compositions of the CsCDPK genes. Note: The phylogenetic trees were constructed via the neighbour-joining method in MEGA 7.0 (left side of the figure). Reliability of the predicted tree was tested by bootstrapping with 1000 replicates. The motif composition related to each CsCDPK protein is displayed in the middle of the figure. The motifs (1–10) are displayed in different coloured boxes, and motif information is provided in . The exon–intron structure of the CsCDPK genes and EF-hand and Pkinase domains are displayed on the right side of the figure. Green boxes indicate untranslated 5′- and 3′-regions, yellow boxes indicate the coding sequence (CDS), and black lines indicate introns. The domains are highlighted by purple or pink boxes, respectively. The length of the protein can be estimated with the scale at the bottom.
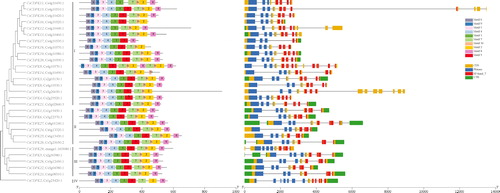
The phylogenetic tree generated from the protein sequence alignment of citrus and Arabidopsis segregated the 29 CsCDPKs into four large groups. Group members shared similar protein sequence lengths, motif compositions and exon–intron structures, suggesting a close relationship. Thus, CsCDPKs and their homolog AtCPKs in the same branch may play similar roles in plant–microbe interactions and abiotic stress responses. AtCPK3 clustered with CsCDPK21 and is required for the phytosphingosine-induced programmed cell death response to Fusarium moniliforme [Citation44]. CsCDPK6 and 27 clustered with AtCPK5, 6 and 11 in the same branch. AtCPK1, 5, 6 and 11 encode redundant positive regulators of the defence responses downstream of multiple pathogen-associated molecular patterns (PAMPs) and damaged-associated molecular patterns (DAMPs), including flg22, elf18, PEP3 and OGs. This is consistent with cpk5, 6 and 11 hypersensitivities to Pseudomonas syringae DC3000 and Botrytis cinerea, since the proteins they encode regulate salicylic acid and ethylene accumulation [Citation19,Citation45,Citation46]. AtCPK28 clustered with CsCDPK2, and AtCPK28 negatively regulates PAMP-induced oxidative bursts by reducing the stability of another RBOHD-stimulating enzyme, Botrytis-induced kinase1 [Citation47]. Drought triggers large-scale transcriptional reprogramming that is partially mediated by the CPKs via regulation of ABA signalling. AtCPK32 clustered with CsCDPK28, and AtCPK32 has been shown to interact with and phosphorylate ABF4, which is important for the ABA-induced transactivation activity of ABF4 [Citation48]. Similarly, AtCPK4 clustered with CsCDPK6, and AtCPK4 has been verified to phosphorylate ABF2 and stimulate its activity in a protoplast transient assay [Citation49]. ABF1 and 4 are substrates of AtCPK4 and 11 whose over-expression triggered drought tolerance and enhanced the expression of the stress-responsive genes RAB18 and KIN1 and 2 (cpk4 and 11 exhibited the opposite phenotype) [Citation11]. The phylogenetic tree predicted that the CsCDPKs are involved in microbe–plant interactions and drought stress, but this hypothesis requires verification in future studies.
Analysis of CsCDPK expression and the cis-elements in CsCDPK promoters
Gene expression analysis revealed that 29 CsCDPKs were variably expressed in the roots. CsCDPK1, 2, 5, 6, 7, 8, 9, 13, 17, 18, 19, 21, 24, 25, 26, 27 and 29 were upregulated by AMF colonization, and CsCDPK3, 7 and 28 were downregulated. Drought-repressed expression of CsCDPK10, 11, 12, 15 and 16 induced CsCDPK20 and 22. Furthermore, AMF colonization and drought stress exerted synergistic effects; CsCDPK2, 3, 6, 13, 17 and 21 expression was enhanced in the D_AMF treatment group, whereas CsCDPK20 and 22 expression was repressed in W_AMF but induced in D_AMF (; Supplemental Table S3). The expression patterns of the nine selected CsCDPKs were analyzed by qRT-PCR to confirm the transcriptome data. The qRT-PCR results showed that CsCDPK7, 20, 22 and 23 expression was induced by AMF and/or drought stress, whereas CsCDPK3, 11, 25 and 27 expression was repressed by AMF and/or drought stress (as expected). CsCDPK4 expression showed synergistic effects for AMF and drought stress. Thus, the qRT-PCR expression patterns were consistent with the transcriptome data ().
Figure 4. Expression profiles of the CsCDPK genes responding to AMF colonization and/or drought stress. Note: The colour scale represents the log2 expression values; the red and blue colours indicate high or low transcript abundances compared to the relevant control (non-AMF plants under the well-watered condition), respectively.
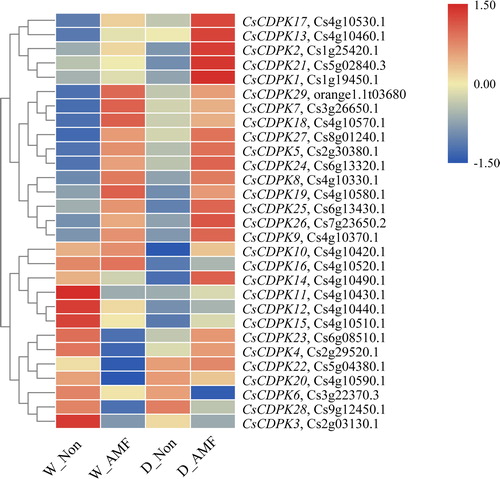
Figure 5. CsCDPK expression with AMF colonization and/or drought stress treatment by qRT-PCR. Note: The relative gene expression was calculated using the 2−△△Ct method, with β-actin as the reference gene. Significant differences between treatments were determined by Duncan’s Multiple Range Tests at p = 0.05. Different letters indicate significant differences.
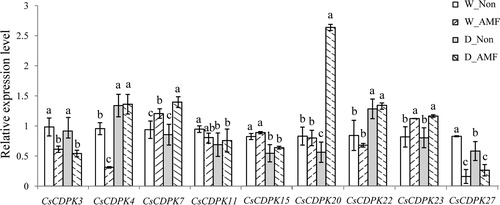
Several cis-elements, including ‘hormone-responsive’, were identified in the upstream regulatory regions (promoters) of the CsCDPKs. The cis-elements for ‘defence and stress responsive’ and ‘drought inducibility’ are responsible for the plant response to mycorrhizal colonization and drought stress. ‘Defence- and stress-responsive’ cis-elements were identified in the promoters of eight CsCDPKs (CsCDPK10, 11, 19, 20, 23, 24, 26 and 29) and ‘drought inducibility’ cis-elements were identified in the promoters of 16 CsCDPKs (CsCDPK1, 2, 4, 5, 6, 8, 11, 12, 13, 15, 16, 19, 20, 21, 28 and 29). Two or more ‘drought inducibility’ cis-elements were identified in CsCDPK8, 10, 11, 13, 15 and 16, indicating greater drought stress regulation. The promoters of CsCDPK10, 11, 19, 20 and 29 contained ‘drought inducibility’ and ‘defence and stress responsive’ cis-elements, suggesting that these genes may be regulated by drought conditions and microbial interactions ().
Figure 6. Predicted cis-elements in the promoters of the CsCDPKs. Note: The ‘defence- and stress-responsive’ and ‘drought inducibility’ cis-elements are indicated by red and blue triangles, respectively.
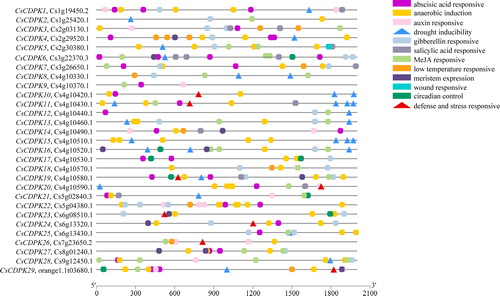
The variable structures and expression patterns of the CsCDPKs indicated different functions. Twenty-nine CsCDPKs were expressed in the roots, implying a role in root development and other associated biological processes. ‘Defence and stress responsive’ cis-elements were identified in the promoters of CsCDPK10, 11, 19, 20, 23, 24, 26 and 29, but CsCDPK1, 2, 5, 6, 7, 8, 9, 13, 17, 18, 19, 21, 24, 25, 26, 27 and 29 were upregulated by AMF colonization (). The patterns of CsCDPK expression were not consistent with the cis-elements in their promoters for ‘defence and stress responsive’, which may be due to the integration of other gene regulatory elements, such as trans-acting factors [Citation50]. AtCDPK3, 5, 6, 11 and 28 are regulators of defence responses, and some of the CsCDPKs (CsCDPK2, 6, 21 and 27 clustered with AtCDPK3, 5, 6, 11 and 28) were induced by AMF colonization. This result suggests that the process regulating CDPKs in response to pathogens and AMF exists with some overlap. ‘Drought inducibility’ cis-elements were present in the promoters of CsCDPK1, 2, 4, 5, 6, 8, 11, 12, 13, 15, 16, 19, 20, 21, 28 and 29, and only CsCDPK20 and 22 were induced by drought. CsCDPK22 clustered with AtCPK30, and a previous study showed that AtCPK7, 10, 12 and 30 interact with and phosphorylate or activate ABF1, ABF2, ABF3 and/or ABF4. Multiple CPKs and ABFs probably constitute an intricate signalling network to regulate gene expression during drought [Citation48,Citation49,Citation51]. The functions of CsCDPK20 and 22 in the signal transduction of CsCDPKs under drought conditions require further study.
Conclusions
We identified 29 CsCDPKs on at least nine chromosomes in the citrus genome. Three clusters were on chromosome 4, with three or two CsCDPKs, and 17 CsCDPKs showed syntenic relationships with the AtCDPKs. All of the CsCDPKs clustered into four major groups, with distinct protein domains, motifs and sequences. Gene expression analysis revealed that 29 CsCDPKs were expressed in the roots, with variable expression patterns. CsCDPK1, 2, 5, 6, 7, 8, 9, 13, 17, 18, 19, 21, 24, 25, 26, 27 and 29 were upregulated by AMF colonization, and CsCDPK3, 7 and 28 were downregulated. The drought-repressed expression of CsCDPK10, 11, 12, 15 and 16 induced CsCDPK20 and 22. Furthermore, AMF colonization and drought stress exerted synergistic effects; CsCDPK2, 3, 6, 13, 17 and 21 expression was enhanced in the drought stress with AMF treatment (D_AMF), while CsCDPK20 and 22 expression was repressed in well-watered plants with AMF treatment (W_AMF) but induced in D_AMF. The regulation of CsCDPK expression was not consistent with the cis-elements in the promoters, and the regulation of the CsCDPKs should be investigated further. Collectively, the results of this study provide a basis for future functional studies of the citrus CDPK proteins and their responses to AMF and drought stress.
Author contribution statement
Bo Shu: conceived the experiments and wrote the manuscript. Chun Luo, Bo Shu, Fei Zhang: carried out the experiments. Chun Luo, Dengwei Jue, Dejian Zhang, Chunyan Liu: analyzed the data. Qiangsheng Wu, Chun Luo: critically reviewed the manuscript.
Supplemental Material
Download PDF (648.9 KB)Disclosure statement
No potential conflict of interest was reported by the authors.
Additional information
Funding
References
- Reddy ASN, Ali GS, Celesnik H, et al. Coping with stresses: roles of calcium- and calcium/calmodulin-regulated gene expression. Plant Cell. 2011;23(6):2010–2032.
- Klimecka M, Muszyńska G. Structure and functions of plant calcium dependent protein kinases. Acta Biochim Pol. 2007;54(2):219–233.
- Harper JF, Harmon AC. Plants, symbiosis and parasites: a calcium signalling connection. Nat Rev Mol Cell Biol. 2005;6(7):555–566.
- Cheng S-H, Willmann MR, Chen H-C, et al. Calcium signaling through protein kinases. The arabidopsis calcium-dependent protein kinase gene family. Plant Physiol. 2002;129(2):469–485.
- Harmon AC, Yoo B, Mccaffery C. Pseudosubstrate inhibition of CDPK, a protein kinase with a calmodulin-like domain. Biochemistry. 1994;33(23):7278–7287.
- Delormel TY, Boudsocq M. Properties and functions of calcium-dependent protein kinases and their relatives in Arabidopsis thaliana. New Phytol. 2019;224(2):585–604.
- Saijo Y, Hata S, Kyozuka J, et al. Over-expression of a single Ca2+-dependent protein kinase confers both cold and salt/drought tolerance on rice plants. Plant J. 2000;23(3):319–327.
- Vivek PJ, Tuteja N, Soniya EV. CDPK1 from ginger promotes salinity and drought stress tolerance without yield penalty by improving growth and photosynthesis in Nicotiana tabacum. PLoS ONE. 2013;8(10):e76392.
- Campo S, Baldrich P, Messeguer J, et al. Overexpression of a calcium-dependent protein kinase confers salt and drought tolerance in rice by preventing membrane lipid peroxidation. Plant Physiol. 2014;165(2):688–704.
- Brandt B, Munemasa S, Wang C, et al. Calcium specificity signaling mechanisms in abscisic acid signal transduction in Arabidopsis guard cells. eLife. 2015;4:e03599.
- Zhu SY, Yu XC, Wang XJ, et al. Two calcium-dependent protein kinases, CPK4 and CPK11, regulate abscisic acid signal transduction in Arabidopsis. Plant Cell. 2007;19(10):3019–3036.
- Wei S, Hu W, Deng X, et al. A rice calcium-dependent protein kinase OsCPK9 positively regulates drought stress tolerance and spikelet fertility. BMC Plant Biol. 2014;14(1):133.
- Kobayashi M, Ohura I, Kawakita K, et al. Calcium-dependent protein kinases regulate the production of reactive oxygen species by potato NADPH oxidase. Plant Cell. 2007;19(3):1065–1080.
- Asano T, Hayashi N, Kobayashi M, et al. A rice calcium-dependent protein kinase OsCPK12 oppositely modulates salt-stress tolerance and blast disease resistance. Plant J. 2012;69(1):26–36.
- Fu L, Yu X, An C. Overexpression of constitutively active OsCPK10 increases Arabidopsis resistance against Pseudomonas syringae pv. tomato and rice resistance against Magnaporthe grisea. Plant Physiol Biochem. 2013;73:202–210.
- Xing T, Wang XJ, Malik K, et al. Ectopic expression of an Arabidopsis calmodulin-like domain protein kinase-enhanced NADPH oxidase activity and oxidative burst in tomato protoplasts. Mol Plant Microbe Interact. 2001;14(10):1261–1264.
- Coca M, Segundo B. AtCPK1 calcium-dependent protein kinase mediates pathogen resistance in Arabidopsis. Plant J. 2010;63(3):526–540.
- Cheng SH, Sheen J, Gerrish C, et al. Molecular identification of phenylalanine ammonia-lyase as a substrate of a specific constitutively active Arabidopsis CDPK expressed in maize protoplasts. FEBS Lett. 2001;503(2–3):185–188.
- Dubiella U, Seybold H, Durian G, et al. Calcium-dependent protein kinase/NADPH oxidase activation circuit is required for rapid defense signal propagation. Proc Natl Acad Sci USA. 2013;110(21):8744–8749.
- Romeis T, Ludwig AA, Martin R, et al. Calcium-dependent protein kinases play an essential role in a plant defence response. EMBO J. 2001;20(20):5556–5567.
- Ray S, Agarwal P, Arora R, et al. Expression analysis of calcium-dependent protein kinase gene family during reproductive development and abiotic stress conditions in rice (Oryza sativa L. ssp. indica). Mol Genet Genomics. 2007;278(5):493–505.
- Li AL, Zhu YF, Tan XM, et al. Evolutionary and functional study of the CDPK gene family in wheat (Triticum aestivum L.). Plant Mol Biol. 2008;66(4):429–443.
- Zuo R, Hu R, Chai G, et al. Genome-wide identification, classification, and expression analysis of CDPK and its closely related gene families in poplar (Populus trichocarpa). Mol Biol Rep. 2013;40(3):2645–2662.
- Liu W, Li W, He Q, et al. Genome-wide survey and expression analysis of calcium-dependent protein kinase in Gossypium raimondii. PLoS ONE. 2014;9(6):e98189.
- Cai H, Cheng J, Yan Y, et al. Genome-wide identification and expression analysis of calcium-dependent protein kinase and its closely related kinase genes in Capsicum annuum. Front Plant Sci. 2015;6:737.
- Zhang K, Han Y, Zhao F, et al. Genome-wide identification and expression analysis of the CDPK gene family in grape, Vitis spp. BMC Plant Biol. 2015;15(1):164.
- Zhang M, Liu Y, He Q, et al. Genome-wide investigation of calcium-dependent protein kinase gene family in pineapple: evolution and expression profiles during development and stress. BMC Genom. 2020;21(1):72.
- Li M, Hu W, Ren L, et al. Identification, expression, and interaction network analyses of the CDPK gene family reveal their involvement in the development, ripening, and abiotic stress response in banana. Biochem Genet. 2020;58(1):40–62.
- Mccormack E, Braam J. Calmodulins and related potential calcium sensors of Arabidopsis. New Phytol. 2003;159(3):585–598.
- Gasteiger E, Gattiker A, Hoogland C, et al. Expasy: the proteomics server for in-depth protein knowledge and analysis. Nucleic Acids Res. 2003;31(13):3784–3788.
- Edgar RC. MUSCLE: multiple sequence alignment with high accuracy and high throughput. Nucleic Acids Res. 2004;32(5):1792–1797.
- Kumar S, Stecher G, Tamura K. MEGA7: molecular evolutionary genetics analysis version 7.0 for bigger datasets. Mol Biol Evol. 2016;33(7):1870–1874.
- Chen C, Chen H, Zhang Y, et al. TBtools: an integrative toolkit developed for interactive analyses of big biological data. Mol Plant. 2020; 13(8):1194–1202.
- Wang Y, Tang H, DeBarry JD, et al. MCScanX: a toolkit for detection and evolutionary analysis of gene synteny and collinearity. Nucleic Acids Res. 2012;40(7):e49.
- Zhang F, Zou YN, Wu QS, et al. Arbuscular mycorrhizas modulate root polyamine metabolism to 374 enhance drought tolerance of trifoliate orange. Environ Exp Bot. 2020;171:103926.
- Luo C, Sun QF, Zhang F, et al. Genome-wide identification and expression analysis of the Citrus malectin domain-containing receptor-like kinases in response to arbuscular mycorrhizal fungi colonization and drought. Hortic Environ Biotechnol. 2020;61(5):891–901.
- Shu B, Li WC, Liu LQ, et al. Transcriptomes of arbuscular mycorrhizal fungi and litchi host interaction after tree girdling. Front Microbiol. 2016;7:408.
- Shu B, Xia RX, Wang P. Differential regulation of Pht1 phosphate transporters from trifoliate orange (Poncirus trifoliata L. Raf) seedlings. Sci Hortic. 2012;146:115–123.
- Livak KJ, Schmittgen TD. Analysis of relative gene expression data using real-time quantitative PCR and the 2−ΔΔCT method. Methods. 2001;25(4):402–408.
- Rombauts S, Déhais P, Montagu MV, et al. PlantCARE, a plant cis-acting regulatory element database. Nucleic Acids Res. 1999;27(1):295–296.
- Hrabak EM, Chan CW, Gribskov M, et al. The Arabidopsis CDPK-SnRK superfamily of protein kinases. Plant Physiol. 2003;132(2):666–680.
- Asano T, Tanaka N, Yang G, et al. Genome-wide identification of the rice calcium-dependent protein kinase and its closely related kinase gene families: comprehensive analysis of the CDPKs gene family in rice. Plant Cell Physiol. 2005;46(2):356–366.
- Chen F, Fasoli M, Tornielli GB, et al. The evolutionary history and diverse physiological roles of the grapevine calcium-dependent protein kinase gene family. PLoS ONE. 2013;8(12):e80818.
- Lachaud C, Prigent E, Thuleau P, et al. 14-3-3-Regulated Ca(2+)-dependent protein kinase CPK3 is required for sphingolipid-induced cell death in Arabidopsis . Cell Death Differ. 2013;20(2):209–217.
- Gravino M, Savatin DV, MacOne A, et al. Ethylene production in Botrytis cinerea- and oligogalacturonide-induced immunity requires calcium-dependent protein kinases. Plant J. 2015;84(6):1073–1086.
- Li S, Han X, Yang L, et al. Mitogen-activated protein kinases and calcium-dependent protein kinases are involved in wounding-induced ethylene biosynthesis in Arabidopsis thaliana. Plant Cell Environ. 2018;41(1):134–147.
- Monaghan J, Matschi S, Shorinola O, et al. The calcium-dependent protein kinase CPK28 buffers plant immunity and regulates BIK1 turnover. Cell Host Microbe. 2014;16(5):605–615.
- Choi HI, Park HJ, Park JH, et al. Arabidopsis calcium-dependent protein kinase AtCPK32 interacts with ABF4, a transcriptional regulator of abscisic acid-responsive gene expression, and modulates its activity. Plant Physiol. 2005;139(4):1750–1761.
- Lu Y, Chen X, Wu Y, et al. Directly transforming PCR-amplified DNA fragments into plant cells is a versatile system that facilitates the transient expression assay. PLoS ONE. 2013;8(2):e57171.
- Chow CN, Chiang-Hsieh YF, Chien CH, et al. Delineation of condition specific Cis- and Trans-acting elements in plant promoters under various endo- and exogenous stimuli . BMC Genomics. 2018;19(Suppl 2):85.
- Lynch T, Erickson BJ, Finkelstein RR. Direct interactions of ABA-insensitive(ABI)-clade protein phosphatase(PP)2Cs with calcium-dependent protein kinases and ABA response element-binding bZIPs may contribute to turning off ABA response. Plant Mol Biol. 2012;80(6):647–658.