Abstract
The microRNAs (miRNAs) are a group of small non-coding RNAs found in both plants and animals. Their functions and target genes have been identified in many plant species. It has been shown that miRNAs have crucial roles in stress responses of plants as much as other defence mechanisms. Throughout abiotic stress, miRNAs regulate their target genes and take part in signalling pathways. In this study, we evaluated the expression profiles of miR156, miR170, miR171, miR395, miR398 and miR845, which have been shown to be related with stress, and their target genes in different tissues of Solanum lycopersicum H-2274 plants under salt stress. We applied 100 mmol/L NaCl to tomato plants for one week and performed quantitative polymerase chain reaction (qPCR) analysis. The results that stood out belonged to miR156 both in root and stem tissues and miR398 in stem tissues. Interestingly the expression profiles of these miRNAs and their target genes were similar. These findings show that there is a complex stress response mechanism still waiting to be deciphered. Our findings could provide valuable information to understand the function of miRNAs in stress response mechanisms of plants.
Introduction
The ability of plants to escape from adverse environmental cues such as drought, salinity, flood, high temperature, cold, UV radiation, heavy metal, herbivores, and pathojen attack is limited due to their sessile nature [Citation1,Citation2]. Abiotic stress factors especially drought and salinity affect plant growth, development and yield negatively [Citation3,Citation4]. Salinity, the second most important stress factor that affects the agricultural lands and restricts production, endangers the sustainability of agriculture with the rapidly growing world population [Citation5]. Salt stress often triggers the activation of detoxification mechanisms for reactive oxygen species (ROS) [Citation6]. Furthermore, salt stress can cause ion toxicity, nutritional disorders, oxidative stress, alteration of metabolic processes, membrane disorganization, reduced cell division and genotoxicity [Citation7,Citation8].
In order to cope with stress factors, plants have developed diverse mechanisms. Expression levels of genes which have roles in a broad spectrum such as signal transduction, energy metabolism, transcription, protein biosynthesis and degradation, membrane traffic, photosynthesis, change as a response to salt stress. In addition, post-transcriptional mechanisms play crucial roles in stress responses of plants. miRNAs, 21-24 nucleotide long non-coding small RNA molecules, are one of the most important elements of post-transcriptional regulation and plant stress response [Citation9]. miRNA-mediated post-transcriptional gene regulation is essential for adaptation to stress conditions as well as normal growth and development of plants [Citation10,Citation11]. To date, many miRNAs have been identified related with biotic and abiotic stress in different plants. Researchers have conducted experiments in relation with nutrient deficiency, drought, cold, high salinity, bacterial infection, mechanical stress, UV-B radiation [Citation3,Citation12–14]. It has been shown that in plants miR156, miR170, miR171, miR395 and miR398 demonstrate stress related expression along with taking part in developmental processes [Citation15]. In Arabidopsis induction of miR171 expression is common during salt, drought and cold stress [Citation16]. Besides, miR395 expression is important during stress since it takes part in the regulation of sulfation-mediated hormonal activity [Citation17]. Furthermore, miR398 is in relation with Cu/Zn superoxide dismutase genes and an important element in oxidative stress response [Citation18]. In contrast, it has been reported that miR845 was not regulated under abiotic stress [Citation19]. It is known that plant miRNAs are generally related with transcription factors or development related genes [Citation20]. Since stress exposure changes the expression levels of miRNA genes, the expression levels of their targets are also affected in a negative or positive manner which results in changes in growth, photosynthetic capacity, hormonal regulation and energy metabolism. In this concept, it is crucial to analyze the expression profiles of miRNA targets along with miRNAs.
Solanum lycopersicum (tomato) is an agronomically important plant due to its antioxidant characteristics as well as being a good source of Vitamin A, Vitamin C, potassium and lycopene [Citation21]. But, salinity in soil and irrigation water in agricultural areas significantly reduces both the yield and quality of tomato production [Citation22]. Moreover, commercial cultivars of tomatoes display sensitivity to salt stress. Until now, the molecular pathways involved in salt stress response in tomato plants have not been completely elucidated. In this aspect, additional studies are needed to clarify the stress molecular response in plants. With this motivation, in this study, we evaluated the expression profiles of six selected miRNAs (miR156, miR170, miR171, miR395, miR398 and miR845) and their selected targets under salt stress condition.
Materials and methods
Plant material, stress treatment and growth conditions
Commercial Solanum lycopersicum H-2274 (tomato) plants were used in this study. All seeds were surface sterilized as described by Candar-Cakir et al. [Citation5]. Surface sterilized seeds were placed on Murashige and Skoog (MS) medium [Citation23] supplemented with 3% (w/v) sucrose and 0.9% (w/v) agar. Seeds and plantlets were incubated in a plant growth chamber under optimized conditions (16 h light/8 h dark illumination, 70% relative humidity, and 25 °C temperature). While the tomato plants belonging to the control group remained in MS medium, the tomato plants to be treated with salt were transferred to MS medium containing salt 100 mmol/L NaCl. For salt stress experiments, 15-day-old tomato plants with 10 plants per experimental group were subjected to 100 mmol/L NaCl for 7 days, while control group plants were grown under the same conditions without salt treatment. At the end of this time period, samples were collected, frozen in liquid nitrogen, and stored at −80 °C until use for molecular analysis.
RNA Isolation and RT-qPCR analysis
Total RNA was isolated from the roots, leaves and stems of the collected samples using TRIzol (HibriGen, Turkey) reagent according to the manufacturer’s instructions. Before cDNA synthesis, RNA integrity and quantity were examined by agarose gel electrophoresis and NanoDrop spectrophotometer, respectively. Since we evaluated the expression profiles of both selected miRNAs and their targets, two different cDNA amplification kits were used in this project. For the miRNA expression analyses, cDNA was synthesized by TaqMan™ MicroRNA Reverse Transcription Kit (Applied Biosystems™) using stem loop primers () and for the expression analyses of selected miRNA targets, cDNA was synthesized by High-Capacity cDNA Reverse Transcription Kit (Applied Biosystems™) using oligo d(T) primer.
Table 1. Primer sequences of the stem loop primers.
Firstly, the expression profiles of 6 selected miRNAs (ath-miR398a-5p, ath-miR845a, ath-miR170-5p, sly-miR395a, sly-miR171a, sly-miR156a) were evaluated in root and stem tissues seperately. RT-qPCR analyses were carried out using 2X SYBR Green Mix (HibriGen, 0120-UB-756) with Roche LightCycler Nano instrument. All reactions were performed in a total volume of 20 µL including 0.5 µmol/L of each primer, 2 µL of cDNA and 10 µl 2X SYBR Green mix. Cycling conditions were as follow: 95 °C for 10 min, followed by 45 amplification cycles of 95 °C for 15 s and 60 °C for 60 s. The obtained Ct values were evaluated relatively using the ΔΔCt method. The primer sequences of the selected miRNAs are given in .
Table 2. Primer sequences of the selected miRNAs used in qPCR analysis.
For the evaluation of gene expression, we selected 11 targets including 6 miRNAs. As mentioned above, RT-qPCR analyses were carried out using 2X SYBR Green Mix with Roche LightCycler Nano instrument. All reactions were performed in a total volume of 25 µL including 0.2 µmol/L of each primer, 1 µL of cDNA and 12.5 µL 2X SYBR Green mix. Cycling conditions were as follows: 50 °C for 60 s, followed by 40 amplification cycles of 94 °C for 30 s, 64 °C for 30 s and 60 °C for 60 s. The obtained Ct values were evaluated relatively using the ΔΔCt method. Actin (the housekeeping gene) (Forward 5’TGTGTTGGACTCTGGTGATGGTGT3’ and Reverse 5’ATCCAAACGAAGAATGGCATGCGG3’) was chosen as the endogenous control for normalization. The primer sequences and accesion numbers of the selected miRNA targets were given in .
Table 3. Primer sequences of the selected miRNA targets used in qPCR analysis.
Target selection
Potential targets of 6 selected miRNAs, known to be related with abiotic stress, were determined by using the bioinformatics tools ‘psRNATarget’ [Citation24–26, <http://plantgrn.noble.org/psRNATarget/#>] and ‘Sol Genomics Network’ [Citation27, <https://solgenomics.net/>].
Statistical analysis
qPCR results and analyses of gene expression were statistically evaluated via one-way analysis of variance (ANOVA) Tukey test and two-way ANOVA using the GraphPad Prism®7 computer program. Differences were considered statistically significant at p < 0.05 level. Experiments were performed with 3 biological and 2 technical repetitions.
Results
Plant germination and stress Implementation
Surface sterilized H-2274 tomato plants were germinated on MS medium after one week of incubation in plant growth chamber. In order to analyze the morphological and molecular effects of salt stress, 0 and 100 mmol/L NaCl were applied to 15-day-old plants during a week. As seen in , the development of tomato plants was affected by NaCl application.
RNA isolation and qPCR analysis
The expression levels of the six selected miRNAs and their selected eleven targets were analyzed via qPCR after salt stress implementation (). We analyzed the expression levels of selected miRNA targets in root and stem tissues.
Figure 2. Graphical representation of the changes in the expression levels of selected miRNAs and their targets in H-2274 tomato plants exposed to salt stress. Expression profiles of miRNAs in root tissue (A) and stem tissue (B); and expression profiles of miRNA targets in root tissue (C) and stem tissue (D).
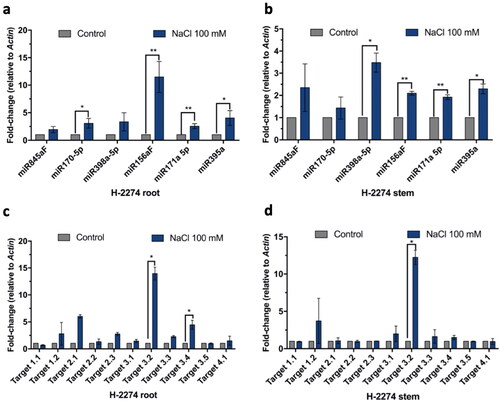
The expression levels of miR156aF, miR171a5p and miR395a were significantly increased in both root and stem tissues (), whereas the expression of miR170-5p was significantly increased only in root tissue () and the expression of miR398a-5p was significantly increased only in stem tissue (). Above all, the expression of miR845aF did not show any significant change.
Also, we analyzed the expression levels of 11 selected miRNA targets in root and stem tissues after salt stress (). The data showed that only the expression of Target 3.2 was significantly increased in both root and stem tissues. In addition, while the expression of Target 3.4 was significanly increased in root tissue (), there was no other statistically significant expression change in the root and stem tissues.
Discussion
Plants cannot escape from stress or changing environmental conditions due to their sedentary lifestyle. They try to adapt or respond to these conditions in order to go on with their lives. To accomplish this, they have developed various mechanisms on morphological, cellular and molecular levels [Citation1,Citation28]. Especially, adverse environmental conditions such as salinity and drought limit the plant growth and productivity. This situation endangers the sustainability of agriculture against the ever-increasing world population [Citation5,Citation29,Citation30]. In order to overcome these negative conditions affecting plant development and yield, plants that can adapt to these conditions and have high yields should be developed. In this context, the first step is to clarify the molecular mechanisms of the plants' responses to changing environmental conditions.
Salinity is one of the most common stress conditions in the world that significantly limits the plant growth and yield. After being exposed to salt, plant cells experience osmotic stress and if the salinity persists for a long time, the ionic balance is disturbed and ionic stress occurs [Citation8,Citation31,Citation32]. Zhang et al. [Citation33] revealed the negative effect of salt stress on photosynthesis and plant growth. In response to salt stress, the expressions of genes involved in a wide range of processes change, such as energy metabolism, signal transduction, transcription, protein biosynthesis and degradation and photosynthesis. Changes such as methylation and histone modification, as well as post-transcriptional regulation also play a crucial role in the response to salt stress. Moreover, it has been demonstrated that miRNAs are one of the important elements involved in post-transcriptional regulation in plants in response to stress factors [Citation9,Citation29,Citation34]. Many miRNA functions are involved in signaling mechanisms to generate stress responses [Citation30]. Although there have been studies on stress conditions and miRNA functions in plants such as Arabidopsis, cotton, rice, and soybean [Citation13,Citation16,Citation19,Citation20,Citation35–38], no comprehensive study showing the relationship between salt stress, miRNAs and targets in tomato plants has been conducted yet. The tomato plant is a preferred crop today with its high lycopene content and high antioxidant enzyme hosting capacity [Citation21,Citation39]. However, the development, yield and nutritional content of tomato plants are affected by environmental factors such as drought and salt stress. Therefore, more efficient and stress-resistant tomato plants should be developed. In order to achieve this, the molecular mechanisms underlying the stress response should be elucidated.
Within the scope of this study, 14-day H-2274 tomato plants were exposed to NaCl at 0 and 100 mmol/L concentrations for 7 days, and their effects on plant growth were observed morphologically. The development of tomato plants with NaCl application was negatively affected and less biomass increase was achieved compared to control plants. Similarly, application of NaCl has a negative effect on root development. Similar studies with maize and Solanum nigrum have shown that NaCl application in various concentrations limits the growth of shoots and roots [Citation40,Citation41]. After morphological analysis, RNA was isolated from tomato plants and miRNAs were reverse transcribed. Meanwhile, miRNAs that had been proven to be related with stress in plants were choosen as listed miR845aF, miR170-5p, miR398a-5p, miR156aF, miR171a-5p, miR395a and their expression profiles were analyzed. As a result of these analyzes, since the data obtained from leaf tissue was not statistically significant, these examples were not included in the expression analysis of the target genes of miRNAs later in the study. Unlike the leaf tissue, a significant increase was observed in the expression of miRNAs both in root and stem tissues over-all. In other words, both in stem and root tissues miR156aF expression was increased most significantly. Similarly, Korir et al. [Citation42] observed an increase in the expression of miR156, especially in root tissues, as a result of miRNA analyzes on tomato plants. In addition, Esposito et al. [Citation43], showed that miR156 has different expression levels in various tissues as a response to cold stress via their study with Solanum commersonii.
In the later stages of the study, the expression profiles of the targets belonging to the 6 selected miRNAs were analyzed and a significant increase was observed in the expression of Target 3.2 both in root and stem tissues. In fact, inversely proportional to the increase in the expression of miR156, the expression of the target gene was expected to decrease; however, the opposite effect was observed. This suggests that the miR156 is not directly related to its target, but there are other intermediate steps in the stress response. As a matter of fact, in a study conducted with maize, Azahar et al. [Citation44] showed that miR156 is related to chromatin rearrangement. Additionally, Cui et al. [Citation45] showed that miR156 is related to branching, blooming, number and quality of fruits as a result of their studies with tomatoes. Moreover, Xie et al. [Citation46], with their analysis in Solanum tuberosum, showed that miR156 is involved in stress response mechanisms as well as functions in metabolic pathways.
Unlike miR156aF, miR398a-5p expression was increased only in the stem tissue. As a result of various studies on this miRNA, it was determined that the miR398 family is associated with oxidative stress mechanisms in higher plants. In addition, miR398 expression has been shown to differ between plant tissues and different stress conditions [Citation47–50]. As a result of their transcriptome study in tomato, Feng et al. [Citation51] determined a 6-fold increase in the expression of miR398a-5p after biotic stress. Paul et al. [Citation52] conducted a study with Passiflora edulis and they have revealed that miR398 expression varies between tissues and is associated with target genes that take part in the defense response of both miR398 and miR171. They have also shown that miR398 is related with target genes which function in salt stress response. Our results support these findings. Additionally, studies with miR171 were conducted and some information was obtained. The miR171 family is highly conserved among plants and their functions in different plant species are determined. As a result of studies with tomato plants, it was revealed that the miR171 family plays a role in anther development, branching and leaf morphogenesis [Citation53]. In addition, Candar-Cakir et al. [Citation5] determined the miRNAs associated with drought stress in tomato and showed that miR171 family plays an important role in the regulation of these stress response genes. Also, an increase in the expression of miR171 under abiotic stress conditions has been observed in Arabidopsis plants and is thought to be involved in the regulation of the stress response [Citation16]. Moreover, miRNAs belonging to miR156/miR157, miR171/miR170, miR398, miR856, miR845a and miR171a family have been found to be involved in response to biotic stress [Citation54–57].
In line with all the studies and the information obtained from this one, as a result of salt and drought stress, different miRNA distribution among the various plant tissues shows that they may have different transcription binding sites. Although some miRNA families involved in post-transcriptional regulation against salt stress have been identified in plants, the role of salt stress-related miRNAs in tomato plants has not yet been established. In order to achieve a sustaniable agriculture along with the global warming, it is crucial to decipher the mechanisms underlying the stress mechanisms. It is believed that the data obtained from this study will be useful in clarifying miRNA related stress response mechanisms.
Conclusions
The expression levels of miR156 in root and stem tissues were increased as well as the miR398 expression in stem tissues. No significant change was observed in other miRNAs (miR170, miR171, miR395 and miR845) whose expression profiles were examined. In addition, an inversely proportional decrease was expected in the expression of miR156 target mentioned as Target 3.2, whereas an increase was observed. These data show that there may be a complex molecular mechanism, including intermediate steps in the formation of the stress response. It is thought that the information obtained from this study will contribute to the elucidation of the molecular mechanisms of miRNA-associated stress response in plants.
Compliance with ethical standards
Ethical Approval: This article does not contain any studies with human participants or animals performed by any of the authors.
Informed Consent: This article does not contain any studies with human participants.
Author contributions
Software, ÖÇ, BA and BK; supervision, ÖÇ and NTK; writing, review and editing, ÖÇ, BA, BK and NTK; Research, BA, BK and NTK; All authors have read and agreed to the published version of the manuscript.
Acknowledgments
We thank Dr. Premkumar Albert Sunderraj (Istanbul University, guest scientist) for technical support. This work was kindly supported by Scientific Research Projects Coordination Unit of Istanbul University with project number 29242.
Disclosure statement
The authors declare no conflict of interest.
Data availability statement
All data that support this study are available from the corresponding author upon reasonable request.
Additional information
Funding
References
- Gravitol C, Hemerly AS, Ferreira PC. Genetic and epigenetic regulation of stress responses in natural plant populations. Biochimica et Biophysica Acta. 2012;1819(2):176–185.
- Suzuki N, Rivero RM, Shulaev V, et al. Abiotic and biotic stress combinations. New Phytol. 2014;203(1):32–43.
- Jatan R, Lata C. Role of MicroRNAs in abiotic and biotic stress resistance in plants. Proc Indian Natn Sci Acad. 2019;85(3):553–567.
- Saini A, Li Y, Jagadeeswaran G, et al. 2012. Role of microRNAs in plant adaptation to environmental stresses. In Sunkar R, editor. MicroRNAs in plant development and stress responses, signaling and communication in plants. Vol. 15. Berlin: Springer.
- Candar-Cakir B, Arican E, Zhang B. Small RNA and degradome deep sequencing reveals drought‐and tissue‐specific micrornas and their important roles in drought‐sensitive and drought‐tolerant tomato genotypes. Plant Biotechnol J. 2016;14(8):1727–1746. https://doi.org/10.1111/pbi.12533.
- Monteiro CC, Carvalho RF, Gratão PL, Carvalho G, et al. Biochemical responses of the ethylene-insensitive never ripe tomato mutant subjected to cadmium and sodium stresses. Environ Exp Bot. 2011;71(2):306–320.
- Tuteja N. Abscisic acid and abiotic stress signaling. Plant Signal Behav. 2007;2(3):135–138.
- Zhu J, Fu X, Koo YD, et al. An enhancer mutant of Arabidopsis salt overly sensitive 3 mediates both ıon homeostasis and the oxidative stress response. Mol Cell Biol. 2007;27(14):5214–5224.
- Vinocur B, Altman A. Recent advances in engineering plant tolerance to abiotic stress: achievements and limitations. Curr Opin Biotechnol. 2005;16(2):123–132.
- Phillips JR, Dalmay T, Bartels D. The role of small RNAs in abiotic stress. FEBS Lett. 2007;581(19):3592–3597.
- Sunkar R, Chinnusamy V, Zhu J, et al. Small RNAs as big players in plant abiotic stress responses and nutrient deprivation. Trends Plant Sci. 2007;12(7):301–309.
- Sunkar R, Zhou X, Zheng Y, et al. Identification of novel and candidate miRNAs in rice by high throughput sequencing. BMC Plant Biol. 2008;8:25.
- Zhao B, Liang R, Ge L, et al. Identification of drought-induced microRNAs in rice. Biochem Biophys Res Commun. 2007;354(2):585–590.
- Zhou X, Wang G, Sutoh K, et al. Identification of cold-inducible microRNAs in plants by transcriptome analysis. Biochim Biophys Acta. 2008;1779(11):780–788.
- Patel P, Yadav K, Ganapathi TR. Small and hungry: microRNAs in micronutrient homeostasis of plants. Microrna. 2017;6(1):22–41.
- Liu HH, Tian X, Li YJ, et al. Microarray-based analysis of stress-regulated microRNAs in Arabidopsis thaliana. RNA. 2008;14(5):836–843.
- Kopriva S, Mugford SG, Baraniecka P, et al. Control of sulfur partitioning between primary and secondary metabolism in Arabidopsis. Front Plant Sci. 2012;3:163.
- Sunkar R, Kapoor A, Zhu JK. Posttranscriptional induction of two Cu/Zn superoxide dismutase genes in Arabidopsis is mediated by downregulation of miR398 and important for oxidative stress tolerance. Plant Cell. 2006;18(8):2051–2065.
- Zhou L, Liu Y, Liu Z, et al. Genome-wide identification and analysis of drought-responsive microRNAs in Oryza sativa. J Exp Bot. 2010;61(15):4157–4168.
- Zhou X, Khare T, Kumar V. Recent trends and advances in identification and functional characterization of plant miRNAs. Acta Physiol Plant. 2020;42(2):25. https://doi.org/10.1007/s11738-020-3013-8.
- Rai AC, Singh M, Shah K. Engineering drought tolerant tomato plants over-expressing BcZAT12 gene encoding a C2H2 zinc finger transcription factor. Phytochemistry. 2013;85:44–50.
- Sekmen A, Demiral T, Tosun N, et al. Tuz Stresi Uygulanan Domates Bitkilerinin Bazı Fizyolojik Özellikleri ve Toplam Protein Miktarı Üzerine Bitki Aktivatörünün Etkisi [Effect of plant activator on some physiological properties and total protein amount of tomato plants exposed to salt stress]. Ege Üniversitesi Ziraat Fakültesi Dergisi. 2005;42(1):85–95. https://dergipark.org.tr/tr/pub/zfdergi/issue/5081/69415.
- Murashige T, Skoog F. A revised medium for rapid growth and bioassays with tobacco tissue cultures. Physiol Plant. 1962;15(3):473–497.
- Dai X, Zhuang Z, Zhao PX. Computational analysis of miRNA targets in plants: current status and challenges. Brief Bioinform. 2011;12(2):115–121.
- Dai X, Zhao PX. psRNATarget: a plant small RNA target analysis server. Nucleic Acids Res. 2011;39(Web Server issue):W155–9.
- Dai X, Zhuang Z, Zhao PX. psRNATarget: a plant small RNA target analysis server (2017 release. Nucleic Acids Res. 2018. 46(W1):W49-W54. doi: 10.1093/nar/gky316.
- Fernandez-Pozo N, Menda N, Edwards JD, et al. The Sol Genomics Network (SGN)-from genotype to phenotype to breeding . Nucleic Acids Res. 2015;43(Database issue):D1036–41.
- Patel P, Yadav K, Ganapathi TR, et al. 2019. Plant miRNAome: Cross talk in abiotic stressful times. In: Rajpal et al., editors. Genetic enhancement of crops for tolerance to abiotic stress: mechanisms and approaches. Vol. I, Sustainable Development and Biodiversity 20. Springer Nature Switzerland AG 2019. https://doi.org/10.1007/978-3-319-91956-0_2. Springer International Publishing
- Bhat K, Mondal TK, Gaikwad AB, et al. Genome-wide identification of drought-responsive miRNAs in grass pea (Lathyrus sativus L). Plant Gene. 2020;21:100210. https://doi.org/10.1016/j.plgene.2019.100210.
- Ding Y, Tao Y, Zhu C. Emerging roles of microRNAs in the mediation of drought stress response in plants. J Exp Bot. 2013;64(11):3077–3086.
- Akbudak MA, Filiz E. Whirly (Why) transcription factors in tomato (Solanum lycopersicum L.): genome-wide identification and transcriptional profiling under drought and salt stresses. Mol Biol Rep. 2019;46(4):4139–4150. https://doi.org/10.1007/s11033-019-04863-y.
- Maathuis FJM, Ahmad I, Patishtan J. Regulation of Na(+) fluxes in plants. Front Plant Sci. 2014;5:467.
- Zhang F, Zhu G, Du L, et al. Genetic regulation of salt stress tolerance revealed by RNA-Seq in cotton diploid wild species, Gossypium davidsonii. Sci Rep. 2016;6:20582.
- Chen X. Small RNAs and their roles in plant development. Annu Rev Cell Dev Biol. 2009;25:21–44.
- Kulcheski FR, de Oliveira LF, Molina LG, et al. Identification of novel soybean microRNAs involved in abiotic and biotic stresses. BMC Genom. 2011;12(307). doi: 10.1186/1471-2164-12-307.
- López-Galiano MJ, García-Robles I, González-Hernández AI, et al. Expression of miR159 ıs altered in tomato plants undergoing drought stress. Plants. 2019;8(7):201.
- Sunkar R, Zhu JK. Novel and stress-regulated microRNAs and other small RNAs from Arabidopsis. Plant Cell. 2004;16(8):2001–2019.
- Xie F, Wang Q, Sun R, et al. Deep sequencing reveals important roles of microRNAs in response to drought and salinity stress in cotton. J Exp Bot. 2015;66(3):789–804.
- Klee HJ, Giovannoni JJ. Genetics and control of tomato fruit ripening and quality attributes. Annu Rev Genet. 2011;45:41–59.
- Ben Abdallah S, Aung B, Amyot L, et al. Salt stress (NaCl) affects plant growth and branch pathways of carotenoid and flavonoid biosyntheses in Solanum nigrum. Acta Physiol Plant. 2016;38(3):72.
- Li H, Yan S, Zhao L, et al. Histone acetylation associated up-regulation of the cell wall related genes is involved in salt stress induced maize root swelling. BMC Plant Biol. 2014;14:105.
- Korir NK, Li X, Xin S, et al. Characterization and expression profiling of selected microRNAs in tomato (Solanum lycopersicon) 'Jiangshu14'. Mol Biol Rep. 2013;40(5):3503–3521.
- Esposito S, Aversano R, Bradeen JM, et al. Deep-sequencing of Solanum commersonii small RNA libraries reveals riboregulators involved in cold stress response. Plant Biol. 2018. 22(S1):133-142.
- Azahar I, Ghosh S, Adhikari A, et al. Comparative analysis of maize root sRNA transcriptome unveils the regulatory roles of miRNAs in submergence stress response mechanis. Environ Exp Bot. 2020;171:103924. https://doi.org/10.1016/j.envexpbot.2019.103924.
- Cui L, Zheng F, Wang J, et al. miR156a-targeted SBP‐Box transcription factor SlSPL13 regulates inflorescence morphogenesis by directly activating SFT in tomato. Plant Biotechnol J. 2020;18(8):1670–1682. https://doi.org/10.1111/pbi.13331.
- Xie F, Frazier TP, Zhang B. Identification, characterization and expression analysis of MicroRNAs and their targets in the potato (Solanum tuberosum). Gene. 2011;473(1):8–22. https://doi.org/10.1016/j.gene.2010.09.007.
- Casati P. Analysis of UV-B regulated miRNAs and their targets in maize leaves. Plant Signal Behav. 2013;8(10):e26758.
- Javed M, Sinha A, Shukla LI. Evaluation of mature miR398 family, expression analysis and the post-transcriptional regulation evidence in gamma-irradiated and nitrogen-stressed Medicago sativa seedlings. Int J Radiat Biol. 2018;95(5):585–596.
- Zhao J, He Q, Chen G, et al. Regulation of non-coding RNAs in heat stress responses of plants. Front Plant Sci. 2016;7:1213
- Zhou ZS, Zeng HQ, Liu ZP, et al. Genome-wide identification of Medicago truncatula microRNAs and their targets reveals their differential regulation by heavy metal. Plant Cell Environ. 2012;35(1):86–99.
- Feng J, Liu S, Wang M, et al. Identification of microRNAs and their targets in tomato infected with Cucumber mosaic virus based on deep sequencing. Planta. 2014;240(6):1335–1352. https://doi.org/10.1007/s00425-014-2158-3.
- Paul S, de la Fuente-Jiménez JL, Manriquez CG, et al. Identification, characterization and expression analysis of passion fruit (Passiflora edulis) microRNAs. 3 Biotech. 2020;10(1):25.
- Kravchik M, Stav R, Belausov E, et al. Functional characterization of microRNA171 family in tomato. Plants. 2019;8(1):10.
- Chauhan S, Yogindran S, Rajam MV. Role of miRNAs in biotic stress reactions in plants. Ind J Plant Physiol. 2017;22(4):514–529. https://doi.org/10.1007/s40502-017-0347-3.
- Fahlgren N, Howell MD, Kasschau KD, et al. High-throughput sequencing of Arabidopsis microRNAs: evidence for frequent birth and death of MIRNA genes. PLoS One. 2007;2(2):e219.
- Tandon G, Singh S, Kaur S, et al. Computational deciphering of biotic stress associated genes in tomato (Solanum lycopersicum). Genom Data. 2017;14:82–90.
- Zuo J, Wang Y, Liu H, et al. MicroRNAs in tomato plants. Sci China Life Sci. 2011;54(7):599–605.