Abstract
Sialic acids (Sia) represent a set of derivatives of nine-carbon sugar neuraminic acid that occupy a terminal position in oligosaccharide chains located on the surface of cells. They are very important for several physiological and pathological processes, including bacterial and viral infections. Until recently, Sia had been described mostly in animals, viruses, bacteria and protozoan species. In the last years, information on sialic acids existence in filamentous fungi has been published, but these data are very scarce. The same is valid for the enzymes that are involved in the synthesis, transport and degradation of Sia in fungi. So far, most data known regarding fungal sialidases are a result of published complete fungal genomes. However, based on the accumulated data, it appears that the kingdom of fungi probably harbours hitherto unknown mechanisms and enzymes of sialic acid metabolism. This review attempts to present the state of knowledge on sialic acids in fungi with respect to their structure, diversity and biological role, as well as Sia metabolism and enzymes involved in their conversion. The summary is presented at the background of the respective metabolic mechanisms possessed by eukaryotes and prokaryotes. The possible role of sialome and sialometabolism in fungi are also discussed.
Keywords:
Introduction
‘Sialic acids are not only the most interesting molecules in the world, but also the most important’. Although this conclusion of the American scientist Eric Sixmister goes beyond the frame of real significance of sialic acids and their metabolism, it is verified by the intensive development of Sialobiology, the science of sialoconjugates [Citation1]. The growing awareness of the significance of sialic acids (Sia) in human health and disease has led to an increase in research into their biochemistry. Sia set the pattern for practically all interactions between cells, compounds and cells, hosts and pathogens in higher animals and humans. Nowadays, their structure, properties and functions are well studied and reviewed by a number of authors [Citation2–11]. Compared to Sia presence in higher animals and prokaryotes, their occurence in fungi has been established later and so far in a few species. However, as early as 1999, the data available until then were summarized in a thorough review by Alviano et al. [Citation12]. They all refer to fungi that can cause dermatomycoses and the possible role of sialic compounds in the pathogenesis has received serious attention. The Kingdom Fungi represents a separate group of eukaryotic organisms, which offers enormous biodiversity with over 100,000 known so far of about 1.5 million existing species [Citation13]. Fungi are involved in the degradation of dead organic matter, including such of animal origin. This suggests the presence of Sia and sialometabolism not only in pathogenic but also in saprophytic species, which is also characteristic of prokaryotes. Indeed, although rare, new data on fungal sialic acids as well as fungal sialidases, key enzymes in sialoconjugates catabolism, continued to emerge in the following years. This review is an attempt to summarize the information published or known to us until now regarding sialic acids and their metabolism in fungi. The vast amount of data on sialic compounds in higher animals and prokaryotes compared to single reports of fungi is an intriguing discrepancy, which could mean that either Sia in fungi are found only in exceptional cases, or the discovery of sialometabolism in them is a matter of time. Any new information in this area would contribute to the development of adequate antifungal therapy for pathogenic species; and for saprophytic ones, to the elucidation of their mechanisms of adaptation to environmental conditions.
Sialic acids
Sialic acids (Sia) comprise a family of about 50 natural derivatives of the nine-carbon sugar neuraminic acid [Citation6]. The unsubstituted form of neuraminic acid contains five hydroxyl, one amine and one carboxyl group (). It is not found in nature, but its N- or О-substituted representatives (sialic acids) are widely distributed in animals and some microorganisms, occupying the terminal position within glycan chains (glycans) of glycoproteins and glycolipids [Citation11]. Usually, the amino group is acetylated to form N-acetylneuraminic acid (Neu5Ac) – the most widely distributed sialic acid (). Replacement of one hydrogen atom in the methyl part of the acetyl group with a hydroxyl group results in the formation of N-glycolyl neuraminic acid (Neu5Gc), which is common in many animal species. In humans, Neu5Gc is found only in cases of certain types of cancer [Citation6]. An unusual modification should be mentioned in which the acetamide group is replaced by an additional hydroxyl group at position 5 of the sugar, leading to 2-keto-3-deoxy-nonulosonic acid (KDN) () [Citation4]. The carboxyl groups of sialic acids are deprotonated at the physiological pH values, thus providing their net negative charge [Citation14].
Figure 1. Chemical structure of: (А) Neuraminic acid. (B) N-acetylneuraminic acid (NANA, Neu5Ac, sialic acid). (C) 2-Keto,3-Deoxynonic acid, KDN.
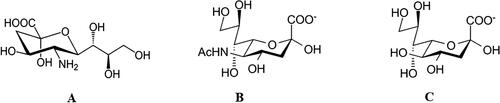
In glycans, sialic acids are linked via C2 by an α-glycosidic bond usually to position 3 or 6 to galactose but sometimes to N-acetyl-D-galactosamine, N-acetyl-D-glucosamine or a second molecule of sialic acid (to position 8). The latter case is in the so-called colominic acid, a homopolymer of Neu5Ac, produced by some strains of Escherihia coli [Citation4]. The diversity of glycans is further enhanced by the biosynthesis of about 50 different types of sialic acids, which are formed by substituents on the hydroxyl groups and the amine group. Thus, according to the world-famous glycobiologist, Professor Roland Schauer, ‘the third language of life’ is formed – the language of glycoconjugates (besides the ones of nucleic acids and proteins) [Citation6]. Along with the oligosaccharide chains of glycoconjugates, Sia are no longer considered inert structures, but rather such that store biological information [Citation15]. Each species has a specific profile of sialoconjugates, depending on the tissue and stage of ontogenetic development.
Functions of sialic acids
The terminal position of Sia makes them capable to influence the physical and chemical properties of glycoconjugates. Due to their negative charge, these ketosugars are involved in binding and transport of positively charged molecules, attraction and repulsion between cells and molecules, and stabilizing the correct conformation of enzymes in the cell membrane [Citation16]. Abundantly represented in mucins, they enhance their viscosity and therefore, their protective function [Citation7]. Sia take part both in recognition processes and masking phenomena between molecules and cells. They serve as ligands for hormones, lectins, antibodies, microorganisms and toxins. On the other hand, they prevent recognition of receptors and protect them from degradation by proteases [Citation14, Citation16, Citation17]. Sialic acids take part in numerous key biological processes as embryogenesis, growth and development of neurons and stem cells, regulation of immune system, host-pathogen interactions [Citation11] and influence the tumor cell metastatic potential [Citation6]. In higher animals they are part of the immune system, giving the organism cells certain identity and cover their antigen receptors, protecting them from autoimmune reactivity [Citation17]. One intriguing and so far unexplored function of Sia is the ability to prevent oxidative damage. They can act as a reactive oxygen species scavenger to reduce the oxidative stress in the inflamed tissues [Citation18].
Distribution of sialic acids in living organisms
Sialic acids are now considered to be exclusively distributed in the animal kingdom and in some prokaryotes, and do not occur in plants. In the animal kingdom, Sia are common in the Deuterostomata superphylum, from Echinodermata to Mammalia. They are abundantly represented as terminal monosaccharides associated with cell surface glycoconjugates in higher animals [Citation19]. Sialic acids are rarely found in protostomes. Such cases are known so far in Drosophila melanogaster, where they occur for a short period during the embryogenesis [Citation20], in the larvae of the cicada Philaenus spumarius [Citation21] and the prothoracic glands of the greater wax moth Galleria mellonella [Citation22]. Some viral glycoproteins, such as those of coronaviruses and Dengue virus, contain sialic acid [Citation23, Citation24].
Sia have also been found in the cell surface glycoproteins of some parasitic protozoa like Trypanosoma cruzi, T. brucei, T. congolense [Citation25]. Recently, the presence KDN in the algae species Prymnesium parvum was announced. The authors believe not only that this algal species can synthesize de novo KDN, but also that sialic acid biosynthesis may be common among microalgae [Citation26]. Substances similar to sialic acids (legionaminic acid) have been found in archaea [Citation27]. However, it is currently assumed that archaea and plants do not possess sialic acids [Citation6, Citation9].
Some bacterial pathogens, such as Campylobacter jejuni, enterohaemorrhagic E. coli, Haemophilus influenzae, H. ducreyi, Neisseria gonorrhoeae, N. meningitidis, P. multocida and Streptococcus agalactiae, may add sialic acid residues to their cell surface as a means of ‘masking’, which prevents it from being recognized by the host immune system. Sialic acids are the only nine-carbon sugars found in prokaryotes [Citation14].
Sialic acids in representatives of the Kingdom Fungi
Sialic acids in the Kingdom Fungi were reported for the first time for the yeast form of the dimorphic fungus Sporothrix schenckii, the causative agent of sporotrichosis, a dermatomycosis commonly known as ‘rose handler's disease’ [Citation28, Citation29]. It is important to note that the yeast form of the fungus is its pathogenic form. The presence of Sia was demonstrated by direct and indirect methods (lectin agglutination and colorimetric assay respectively). The neuraminic acid derivative present in S. schenckii glycolipids was assessed as N-glycolylneuraminic acid by thin-layer chromatography of hydrolysates of polar lipid fractions. Traces of N-acetylneuraminic acid were also detected. A minimum density of 2.0–2.8 × 106 residues of sialic acid per cell was measured in S. schenckii. The contribution of sialic acids to pathogenicity was supported by the results from phagocytosis experiments. Removal of sialic acid by neuraminidase caused a 7.7-fold increase of yeast phagocytosis by mouse peritoneal macrophages. The authors concluded that in the absence of an effective immune response the presence of sialic acid residues may significantly increase the survival of S. schenckii yeast forms in vivo [Citation28]. The infective yeast forms have a double acidic cell surface layer rich in sialic acids, whereas saprophytic hyphae have a single acidic layer [Citation12].
These findings were followed by reports for sialic acids present in several fungal species, most of them living as saprophytes, but often associated with opportunistic infections in man. A combination of several methods has been used in each study, including mild hydrolysis and extraction procedures, HPTLC, gas-chromatography, colorimetric assays, mass spectroscopy, lectin-golden labeling, various lectins and influenza C virus binding.
Another dimorphic fungus, Fonsecaea pedrosoi, was reported to contain sialic acids. F. pedrosoi is commonly found in tropical and sub-tropical regions where it grows in soil. It is one of the causative agents of chromoblastomycosis, a chronic infection of skin and subcutaneous tissues in man. Souza et al. [Citation30] describe the occurrence of sialoconjugates in hyphae and conidia of F. pedrosoi grown in a synthetic medium. The types of sialic acid residues were identified as N-acetylneuraminic (Neu5Ac) and N-glycolylneuraminic (Neu5Gc). Neu5Ac was the only sialic acid type found in the mycelium, while both derivatives were detected in conidia, with predominance of Neu5Gc [Citation30]. In a later study it was demonstrated that the parasitic forms of F. pedrosoi (sclerotic cells) did not produce Sia even when they were grown in media containing external sialic sources [Citation31]. The same authors analyzed the nature of the sialylated macromolecules in mycelium and conidia and characterized them as sialoproteins with molecular masses of 44, 56 and 77 kDa. The 77-kDa glycoprotein was associated particularly with the conidial extracts, confirming the suggestion of Souza et al. [Citation30] that sialic acid expression is associated with fungal morphogenesis [Citation31]. Moreover, agglutination of conidia in the presence of influenza C virus suggested the occurrence of 9-O-acetylated sialic acid forms. The authors suggested the occurrence of 9-O-acetylated Sia, derivatives that are more resistant to sialidase cleavage. Clearly noticeable changes in the morphology of F. pedrosoi hyphae have been observed after treatment with sialidase [Citation31]. The same phenomenon is observed in some mammalian cells that lose some of their Sia. Sialic residues are likely to increase the viscosity inherent in some glycoproteins, and this property alters the structural role of these macromolecules [Citation32].
One intriguing discovery of sialic acids in a parasitic fungus that is not associated with animal host is in Ascocalyx abietina. It causes the scleroderric canker in conifers, resulting in severe damage for pine, fir and spruce forests in Europe and North America. The hyphae of this fungus produce an extracellular matrix that covers all cells, and is suggested to perform various biological functions. Sialic acids occur in the lipid bodies of the dense fibrillar network of this matrix, probably linked as terminal residues in glycolipids or glycoproteins. In view of the peripheral position of the sialogonjugates in the fibrillar network, the authors suggest Sia might be a strong determinant of the pathogenicity of this fungus. Their negative charge may contribute to the transport of cationic compounds through the cell wall, and to the slimy character of the matrix, preventing fungal cells aggregation and enhancing protection to unfavourable environmental conditions [Citation33, Citation34].
There is evidence for occurrence of sialic acid in trace amounts in Pneumocystis carinii (now referred to as Pneumocystis jirovecii) cyst wall [Citation35, Citation36]. This species is a major cause of morbidity and mortality in patients with AIDS and immunocompromised patients. Initially it was considered a protozoan before being reclassified as an ascomycetous fungus in 1988 [Citation37].
A sialoglycoprotein exoantigen was identified in Cryptococcus neoformans, the etiological agent of cryptococcosis in the lungs of immunocompromised people, especially those with advanced HIV/AIDS [Citation38]. Cryptococcosis can also affect the central nervous system, and other organs. This species has an encapsulated yeast form and a filamentous reproductive phase that produces basidiospores. Its affiliation to the phylum Basidiomycota seems to be a rare case, since most fungal sialic acids have been found among members of the phylum Ascomycota. Rodrigues et al. [Citation39] have demonstrated that the yeast form of C. neoformans, grown in chemically defined medium, contains terminal Neu5Ac and N-acetyl-9-O-acetylneuraminic acid (Neu5, 9Ac2) linked by α,2-6 and α,2-3 bonds to a galactose unit of certain glycoproteins located on the surface of the yeast cell wall, and are suggested to play an essential role in the initial stages of infection.
The major virulence factor of C. neoformans, its capsular polysaccharide, is not fully accomplished in the early stages of infection and invading cells are usually eliminated by the alveolar macrophages. However, some C. neoformans cells can survive intracellularly in macrophages [Citation40]. The expression of Sia could, at this stage, play an important role in protecting the fungus against phagocytosis, until the yeast cells are fully encapsulated [Citation39]. In a further study, aiming to identify the sialoconjugates and the process of their synthesis [Citation41], the authors revealed two glycoproteins, with molar masses of 38 and 67 kDa, and a sialyltransferase activity in a partially purified protein fraction from cryptococcal yeast forms. These results indicated that sialic acids in C. neoformans are linked to glycoproteins, which are sialylated by the action of a fungal sialyltransferase, being the first demonstration of this biosynthetic step in pathogenic fungi.
The first report on the occurrence of Sia in an agent of a systemic mycosis was the identification of Neu5Ac in Paracoccidioides brasiliensis [Citation42]. Agglutination by peanut lectin after sialidase treatment showed that Neu5Ac are linked to subterminal β-galactopyranosyl units. The expression of Neu5Ac in P. brasiliensis grown in a chemically defined medium indicates that these residues are synthesized de novo. The authors suggest that surface sialoglycoconjugates of the infective yeast form of P. brasiliensis may inhibit fungal phagocytosis during early infection [Citation42]. As in F. pedrosoi, a difference in the expression of surface sialoglycoconjugates has been observed, in correlation with the growth phase of P. brasiliensis. The mature budding cells contained more sialic acid residues than young yeasts. Moreover, the aged yeast cells without budding displayed considerable decrease of sialylation, resulting in marked enrichment of surface β-galactosyl residues. This unmasking phenomenon could favor the phagocytosis of yeast cells in vivo [Citation12].
Candida albicans, which is part of human natural microflora but often can cause serious infections, is also shown to express sialic acid conjugates on its cell surface. The surface-located iC3b glycoprotein receptor of C. albicans was found to contain Sia [Citation43], as well as certain surface mannoproteins of both blastoconidia and pseudohyphae [Citation44], one of them associated with adhesion to host cells [Citation12]. Sialic acid residues were suggested to contribute to the negative charge of the cells [Citation45].
Wasylnka et al. [Citation46] have demonstrated that Aspergillus fumigatus possesses Sia on the surface of its conidia. A. fumigatus is a ubiquitous saprophytic fungus. Humans and animals constantly inhale its numerous airborne conidia, which are normally either eliminated in the immunocompetent host by innate immune mechanisms, or cause mild to moderate bronchopulmonar infections or allergies. With increases in the number of immunocompromised patients worldwide, this fungus has gradually become the major cause of severe and often fatal invasive aspergillosis in humans [Citation47]. The infection depends on the ability of A. fumigatus conidia to adhere to host lung cells [Citation48]. According to Wasylnka and Moore [Citation49], A. fumigatus conidiospores bind to fibronectin and intact basal lamina significantly better than other non-pathogenic Aspergillus species. In a following study [Citation46] it was demonstrated that A. fumigatus conidia bind to fibronectin in part via sialoglycoconjugates on the spore surface by an interaction between the negatively charged Sia and positively charged regions of the fibronectin protein. The authors have supposed that this binding in vivo may be important in the development of invasive aspergillosis. They have also estimated a density of 6.7 × 105 sialic acid residues per conidium, and have identified them as Neu5Ac [Citation46]. Another study using lectin binding revealed that the sialic acids are α2,6-linked to a galactose residue [Citation50]. The information available so far on sialic acids found in fungi is briefly summarized in .
Table 1. Presence of sialic acids and relevant enzymes in fungi, as reported so far in available literature.
Sialic acid metabolism in eukaryotes
Having a fundamental importance in Kingdom Animalia, sialic acid metabolism has been thoroughly studied and described [Citation6, Citation10, Citation15, Citation51, Citation52]. Due to the large number of modifications of Sia themselves, as well as the compounds to which they bind, sialometabolism is a complex network of reactions involving a large number of enzymes. However, the main pathways of synthesis, sialylation and degradation can be represented as a general scheme of several key transformations performed by certain enzymes (.).
Figure 2. Schematic presentation of sialometabolism in eukaryotes. Green arrows show the steps of Neu5Ac de novo synthesis; orange arrows, sialylation, and blue arrows, catabolic steps. PSA, polysialic acid.

The synthesis of sialic acid starts from glucose in the cytosol. The crucial step in the long chain of reactions is the epimerisation of UDP-N-acetylglucosamine to N-acetylmannosamine-6-phosphate (ManNAc6P). Neu5Ac is synthesized by condensation of phosphoenol-pyruvate with ManNAc6P to yield N-acetyl-9-phosphoneuraminic acid (Neu5Ac9P), followed by dephosphorylation. The free Neu5Ac is activated to CMP-Neu5Ac in the nucleus [Citation6]. A specific nucleotide sugar transporter mediates CMP-Neu5Ac transport into the endoplasmic reticulum and Golgi complex for subsequent addition to glycoconjugates. Other modifications such as various O-acetylation and O-methylation, as well as polymerization of Neu5Ac take place in the Golgi apparatus [Citation51]. Glycoconjugates are either transported to cell surface, or secreted, or they are delivered to lysosomes. Lysosomal sialidases cleave Sia and they are transported into the cytosol. There, they can undergo another cycle of sialyl glycoconjugate synthesis, or further catabolisation by a cytosolic aldolase, yielding ManNAc and pyruvate, the latter entering the oxidative TCA pathway of energy production [Citation6].
It is important to note that there are unicellular eukaryotes that neither synthesize nor catabolize sialic acids, but express them at their cell surface [Citation53]. This phenomenon has been observed, for example, in the parasitic protozoa that cause American Chagas disease and the African sleeping sickness, Trypanosoma cruzi, and T. brucei. It is achieved using a special type of sialidases located on the surface of their cell membrane. These enzymes, called trans-sialidases, cleave the sialic residue from the substrate, but transfer it to another glycan of surface glycoproteins instead of releasing it into the medium ().
Sialic acid metabolism in bacteria
Unlike animals, where Sia are involved in cell–cell interactions, development, immune homeostasis and other complex processes, in bacteria sialic acids serve primarily as a source of carbon, nitrogen, energy and cell wall synthesis. In pathogenic bacteria Sia are involved in the process of infection and colonization of the host, or play dual role, both in nutrition and pathogenicity. Although Sia metabolic pathways aim different biological purposes in eukaryotes and bacteria, both include similar enzymes that use common mechanisms to catalyze the biosynthesis, degradation, and transfer of sialic residues. In some prokaryotes, this set of enzymes can be observed in its complete form, but is most often presentеd in various abbreviated variants, depending on its particular biological sense ().
Figure 4. Schematic presentation of sialic acid metabolism in bacteria. Green arrows show the steps of Neu5Ac de novo synthesis; orange arrows, sialylation; and blue arrows, catabolic steps. Cylinders in blue and green in outer membrane present ABC- and TRAP-transporters; Red oval in periplasm, mutarotase; White cylinder, permease NanT. Abbreviations: PSA, polysialic acid; CPS, capsule polysaccharide; LPS, lipopolysaccharide.
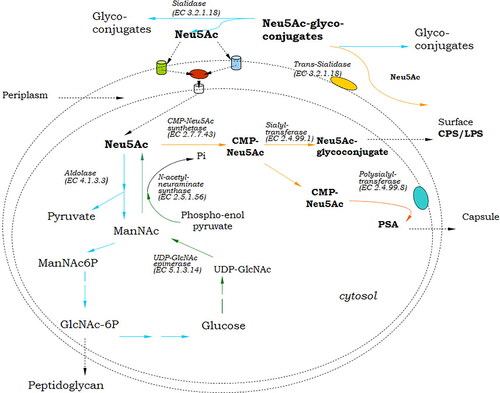
Only a limited number of pathogenic bacteria (some N. meningitidis serotypes, C. jejuni, S. agalactiae) and commensals (E. coli serotype K1, Fusobacterium nucleatum) are able to synthesize de novo sialic acid [Citation54, Citation55]. These species assemble sialic acid into cell surface glycolipids, protecting themselves from the detection of host immune system [Citation9]. As in eukaryotes, the anabolic pathway of Neu5Ac in bacteria starts with the conversion of UDP-GlcNAc to ManNAc by UDP-GlcNAc-epimerase (). Unlike eukaryotes, ManNAc produced by bacteria is directly used in the presence of phosphoenolpyruvate by Neu5Ac synthase for the formation of Neu5Ac without the involvement of a kinase or a phosphatase [Citation51].
Most bacterial pathogens obtain sialic acid by scavenging pathways, in which free sialic acid is obtained directly from the host (N. gonorrhoeae, H. influenzae, and Pasteurella multocida) [Citation51]. Similar to eukaryotes, sialylation in bacteria is mainly catalyzed by sialyltransferases and polysialyltransferases. Trans-sialylation has also been found in certain pathogenic bacteria (Corynebacterium diphtheriae) which lack the ability to synthesize sialic acid themselves [Citation55]. These organisms cleave Sia from the sialoglycoconjugates of animal host cells by their surface-localized trans-sialidases and decorate their cell surfaces to evade the host’s immune system [Citation53].
The fundamental differences in the structure and biology of eukaryotic and prokaryotic organisms lead to different spatial organization of sialic acid metabolism. In contrast to eukaryotes, in bacteria part of the catabolism of sialic compounds as well as part of sialylation takes place outside the cell, by secretory sialidases or by sialyltransferases and trans-sialidases incorporated into the cell membrane. Polysialyltransferases (localized in eukaryotes in the Golgi complex membranes) in bacteria are cytoplasmic membrane associated enzymes [Citation51].
Many pathogenic and commensal bacteria use sialic acid as a nutrient. The first step in sialic acid catabolism is carried out by sialidases, which cleave these terminal residues from sialoconjugate substrates. Free sialic acids are broken down by Neu5Ac lyase to form ManNAc and pyruvate, which are respectively directed to glycolysis and the tricarboxylic acid cycle [Citation9]. In bacteria that assimilate Sia as carbon source, sialic acid residues are transported across the cell membrane to the interior of the cell by means of specific transmembrane proteins. Three types of such proteins have been described in gram-negative bacteria. Two of them (porins) are located in the outer membrane – ABC-transporters and TRAP-transporters. Once in the periplasm, sialic acid undergoes a temporary steric conversion from its usual α-anomer to a β-anomer, catalyzed by specific mutarotase. The β-anomer is thermodynamically more advantageous for the NanT permease located in the inner membrane through which it passes into the cytoplasm. In the cytosol, sialic acids regain their α-configuration and undergo further degradation.
Both in eukaryotes and bacteria, the three most important groups of enzymes that have the ability to cleave and transport sialic acid are sialidases, sialyltransferases and trans-sialidases. Sialidases (neuraminidases, acetylneuraminyl hydrolases EC 3.2.1.18) cleave the terminal sialic acid residues from various sialyl substrates like glycoproteins, glycolipids, gangliosides, and polysaccharides [Citation55]. Sialyltransferases (E.C.2.4.99.X) are key enzymes in the biosynthesis of sialoglycoconjugates. They catalyze the transfer of sialic residues from their activated form, citidine 5′-monophosphate sialic acid (CMP-Neu5Ac) to appropriate acceptors usually containing terminal galactose, N-acetylgalactosamine (GalNAc) or another sialic acid [Citation8]. Trans-sialidases (E.C. 3.2.1.18) are multifunctional enzymes which gather two activities and two distinct active sites on one molecule. In the absence of suitable acceptor, these enzymes act as sialidases transferring the sialic acid residues to water molecules and are therefore classified in the same enzyme group [Citation56].
Enzymes of sialic acid metabolism in fungi
The existence of sialic compounds in species of the fungal kingdom presupposes the presence of at least some of the enzymes necessary for their synthesis, transfer, degradation or acquisition by the environment. The detection of Sia in P. brasiliensis, C. neoformans and F. pedrosoi grown in a medium free of sialic acid-containing substances, indicates that these ketosugars are synthesized de novo and are transferred to terminal β-galactosyl residues probably by regular sialyltransferases [Citation12].
Glycosylation of proteins in fungi takes place by approximately the same mechanism as in mammalian cells. Proteins having the corresponding signal peptide enter the endoplasmic reticulum, where they are glycosylated and transferred to the Golgi apparatus for further transformation. From there, they are directed to the relevant organelles, the plasma membrane and the cell wall, or secreted into the periplasm [Citation12]. Since the conclusion of Alviano et al. [Citation12] that the addition of sialic residues to fungal glycoproteins is a completely unknown process, no new data have been obtained to disprove it. A. fumigatus is the most thoroughly studied representative of filamentous fungi in terms of sialic acid and sialidase expression. According to Tiralongo et al. [Citation48] A. fumigatus is incapable of utilising or incorporating exogenous Neu5Ac or ManNAc. The origin of its surface Sia remains a mystery, as although sialic acid de novo biosynthesis has been reported in A. fumigatus [Citation46], its genome appears to lack the genes for the known Neu5Ac biosynthetic enzymes [Citation57, Citation58]. However, an alternative pathway exists for sialic acid acquisition in bacteria, the uptake of free sialic acid via plasma membrane permeases [Citation14], and such mechanism is possible to occur in fungi.
The first report of the presence of sialidase in fungal species dates back to 1974. Uchida et al. [Citation59] have screened 1000 microorganisms, 380 of them being fungi, and neuramidases were detected in S. schenckii and Penicillium urticae (deposited as a neuraminidase producer strain P. griseofulvum Dierckx, ATCC® 32794™). Muller [Citation60] reports the occurrence of neuraminidase in S. schenckii and Ceratocystis stenoceras.
These findings remained the only reports of fungal sialidases until 2005, when a novel sialidase was described in A. fumigatus [Citation61]. In a number of following studies, A. fumigatus sialidase has been extensively investigated. It has been cloned and characterized by Warwas et al. [Citation62]. A year later its crystal structure was revealed, being the first crystal structure of a fungal sialidase. Moreover, it was found that this is actually a KDNase – enzyme that cleaves KDN (Figure1C) [Citation57]. The crystal structure reveals that the enzyme is a dimer of two identical protein chains, of 42 kDa each. A. fumigatus sialidase has 30% identity to the sialidase of the bacterial species Micromonospora viridifaciens (PDB 1eus). Its sequence has a 20-amino-acid signal peptide and contains the conservative key residues at the sialidase active site [Citation57, Citation62]. A row of consecutive studies described its kinetics, specificity, localization and inhibition thoroughly [Citation58, Citation63–65, etc.]. The authors have proved that A. fumigatus can utilize KDN and not Neu5Ac as a sole carbon source [Citation57]. No CMP-sialic acid transport was detected. A. fumigatus KDNase was found to be evenly distributed throughout the cell, with a higher concentration on the cell periphery [Citation63].
In 2011, a patent was published describing the identification, isolation and purification of extracellular sialidase from P. chrysogenum. The main physicochemical characteristics of the enzyme are described, as well as its cloning into another filamentous fungus strain, A. niger CBS513.88, in order to increase its production [Citation66]. The polypeptide of the invention can be used in the preparation of food or feed, or as a medicament or part of a medicament. P. chrysogenum strain Wisconsin 54-1255 sialidase gene ZJW has homology with sialidase sequences of Actinomyces and Arthrobacter. The enzyme is extracellular, with a predicted molecular weight of 42.7 kDa. Dekker et al. [Citation67] have registered a patent application of manufacturing sour milk preparations with the use of a sialidase from P. chrysogenum.
Sakarya and Gunay [Citation68] demonstrated that the probiotic yeast Saccharomyces boulardii expresses sialidase, selective for α (2-3)-linked sialic acid. It removes surface α (2-3)-linked sialic acid of the sialyl-Lewis x antigen, which is abundantly expressed on the inflamed gastrointestinal epithelial cell surface. This antigen is the ligand for the sialic acid-binding Helicobacter pylori adhesion. The action of S. boulardii sialidase inhibits H. pylori adherence to duodenal epithelial cells. Recently published data from projects for whole genome sequencing, show that species of the genera Penicillium, Aspergillus, Sporotrichum, Alternaria, Fusarium, Colletotrichum, etc, (almost exclusively belonging to the phylum Ascomycota) possess genes with putative sialidase activity.
As pointed out by Alviano et al. [Citation30], the presence of sialyl-galactosyl sequences in fungal cells grown in a chemically defined medium indicates a sialyltransferase-dependent sialylation. Angata and Varki [Citation5] note the possibility that Sia in fungi are obtained from external sources and also do not rule out that fungal cells could have alternative pathways to synthesize and express sialic acids. According to Petit et al. [Citation69], no sialyltransferase-related sequence could be identified in fungi. The first and so far only demonstration of glycosylation process in partially purified preparation was reported by Rodrigues et al. [Citation41], in C. neoformans. One possible explanation for the presence of sialic derivatives in fungi without the detection of enzymes for their biosynthesis is the presence of trans-sialidases. These enzymes would deliver sialic acid residues directly from the host or environment, binding them to appropriate glycans on the cell surface. As far as we know from the available literature, such enzymes in fungi have not yet been found. Despite the experiments of a number of scientists who have studied the sialometabolism of A. fumigatus in detail, no trans-sialidase genes have been found in its genome. The presence of O-substituted sialic acids in fungi also suggests the presence of corresponding O-acyltransferases.
Currently, in view of the above described data, a Bulgarian scientific team including our group is focused on investigation of the presence of sialidase activity in fungal isolates from various environmental sources. The results demonstrated sialidase presence in a wide range of non-clinical fungal strains [Citation70].
Biological role of sialome and sialometabolism in fungi
It is not easy to summarize the diversity and role of sialic compounds due to their direct or indirect participation in numerous and diverse processes. A novel term, ‘sialome’ has been introduced by Varki and Angata ‘to denote the total complement of sialic acid types and linkages and their modes of presentation on a particular organelle, cell, tissue, organ, or organism—as found at a particular time and under specific conditions’ [Citation71].
Sialic acids are distinctive by their multifunctionality. In microorganisms which are pathogens or commensals in animals, the expression of sialic compounds on the cell surface is associated with avoiding the action of the host's immune system. As mentioned above, the authors, describing the presence of sialic compounds in pathogenic fungal species, provide evidence for their contribution to the protection against phagocytosis, resistance to sialidase action, coverage of antigenic determinants, adhesion to host tissues. On the other hand, sialoside compounds in fungi appear to be involved in roles, which have not been observed in bacteria so far, such as the structural function suggested in F. pedrosoi, assumed to be more characteristic of eukaryotes. An interesting exception in this respect is A. abietina, a species that has nothing to do with substrates associated with animal tissues: it does not decompose organic matter of animal origin, nor is it found in animal hosts. In this case, the role of Sia in the pathogenic process is related to their physicochemical properties, which make them an effective shell, facilitating the growth of hyphae, the transfer of positively charged ions and protection from drought.
For some microbial pathogens, sialidases are believed to act as virulence factors. It has been demonstrated that sialidases play a substantial role in a number of infectious diseases like cholera, gas gangrene, meningitis, septicemia, cystic fibrosis, etc. [Citation72–75]. Most sialidase-producing pathogenic bacteria utilize sialoconjugates as carbon, nitrogen and energy sources. The analysis of nearly 2000 bacterial genomic sequences shows that genes required for sialic acid catabolism are restricted to species that are commensal or pathogenic in animals. Most of these species colonize surfaces rich in Sia such as the respiratory and digestive tract, suggesting that sialic catabolism plays an important role in their survival in vivo [Citation76]. Bacteria enter the mucosa from the external environment and begin to grow in it as in a substrate. In commensal bacteria, the role of sialidase is limited to the catabolisation of sialic acids, while pathogenic bacteria use the cleavage of sialic residues by sialidase also to destroy the mucous layer, thus penetrating the very surface of the epithelium. It thus becomes available to other enzymes, such as proteases, hyaluronidases, collagenases, and other enzymes, which allow the bacterium to destroy tissues and penetrate deep into them [Citation4]. By removing terminal sialic residues from various sialoconjugates (membrane-type glycoproteins and glycopeptides, gangliosides, proteoglycans) located on the cell surface, the enzyme reveals receptors to which the infection agent binds using adhesins. Receptors for toxins are also revealed (a typical example is cholera toxin, ). Penetrating the cells, toxins cause a chain of biochemical processes damaging them and the macroorganism. Thus, any role of the enzyme in bacterial pathogenesis can be a consequence of its trophic function [Citation72].
Figure 5. Binding and penetration of cholera toxin (CT) into epithelial cell. Vibrio cholerae sialidase cleaves sialic acid (violet triangle) from membrane associated ganglioside (GT1b) to yield GM1 – the receptor for CT. The GM1-CT complex is internalized into the cell, where it activates adenylate cyclase (AC). High levels of cyclic AMP (cAMP) lead to intensive secretion of Na+, K+, Cl-, HCO3- and H2O into the intestine lumen, resulting in diarrhea and dehydration – the major symptoms of cholera.
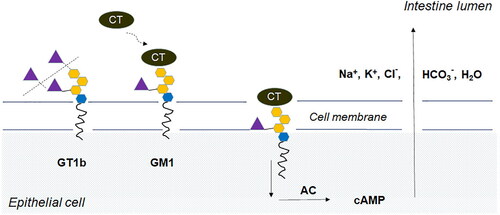
It is likely that the same relation is valid also for filamentous fungi, since the sialidase producers known so far among them can cause diseases. For example, fungal species like S. schenckii, and F. pedrosoi, which are widely distributed in soil and decompose plant material, can infect humans as well as animals and are the causative agents of dermatomycoses. A. fumigatus can cause severe disease in immunocompromised patients. It can utilize KDN as a sole carbon source [Citation57].
Remarkably well adapted to all conditions on Earth, fungi are invariably present in virtually all terrestrial ecosystems, and are key players in the processes that sustain life on the planet. Given the widespread distribution of sialic derivatives in animal tissues, it is logical to assume that filamentous fungi involved in the degradation of dead organic matter of animal origin (containing sialic compounds) may possess sialidases, aldolases and other enzymes needed for the catabolism of these substances. Of the sialidases described so far, this is the case with P. chrysogenum, which has rarely been reported as a cause of human disease. It is even more likely for some mold species which are parasitic on animals to possess such enzymes. Our observations on a large number of fungal strains isolated from various natural sources, as well as the available gene sequences of putative fungal sialidases, indicate that the actual number of saprophytic producers is probably significantly higher than previously thought.
Conclusions
Given all the available data on the presence of sialic acids in fungi, the numerous gene sequences of fungal sialidases, as well as the isolated so far observations of sialyltransferase and aldolase activity, it can be assumed that fungi have also other enzymes of sialic metabolism that are yet to be identified. Since their search by analogy with already known proteins does not yield results, it is possible that they are novel, hitherto unknown enzymes that perform the biosynthesis and transfer of sialic acids by alternative pathways.
Disclosure statement
No potential conflict of interest was reported by the authors.
Additional information
Funding
References
- Vimr E. Microbial sialidases: does bigger always mean better? Trends Microbiol. 1994;2(8):271–277.
- Varki A. Diversity in the sialic acids. Glycobiology. 1992;2(1):25–40.
- Schauer R, Kamerling J. Chemistry, biochemistry and biology of sialic acids. New Compr Biochem. 1997;29:243–402.
- Traving C, Schauer R. Structure, function and metabolism of sialic acids. Cell Mol Life Sci. 1998;54(12):1330–1349.
- Angata T, Varki A. Chemical diversity in the sialic acids and related alpha-keto acids: an evolutionary perspective. Chem Rev. 2002;102(2):439–470.
- Schauer R. Sialic acids: fascinating sugars in higher animals and man. Zoology (Jena)). 2004;107(1):49–64.
- Varki A. Sialic acids in human health and disease. Trends Mol Med. 2008;14(8):351–360.
- Chen X, Varki A. Advances in the biology and chemistry of sialic acids. ACS Chem Biol. 2010;5(2):163–176.
- Vimr E. Unified theory of bacterial sialometabolism: how and why bacteria metabolize host sialic acids. ISRN Microbiol. 2013; 2013:816713
- Ghosh S. Sialic acid and biology of life: an introduction. In: Sialic acids and sialoglycoconjugates in the biology of life, health and disease. In Versteeg-Buschman L, editor. London (UK): Academic Press; 2020. p. 1–61.
- Tiralongo J, Martinez-Duncker I. Sialobiology: structure, biosynthesis and function. In Tiralongo J, Martinez-Duncker I, editors. Sialic acid glycoconjugates in health and disease. Sharjah (UAE): Bentham Science Publishers; 2013; pp. 618.
- Alviano CS, Travassos LR, Schauer R. Sialic acids in fungi: a minireview. Glycocon J. 1999;16(9):545–554.
- Hawksworth D. The fungal dimension of biodiversity: magnitude, significance, and conservation. Mycol Res. 1991; 95(6):641–655.
- Vimr E, Kathryn A, Deszo E, et al. Diversity of microbial sialic acid metabolism. Microbiol Mol Biol Rev. 2004; 68(1):132–153.
- Bonten EJ. Lysosomal neuraminidase – a unique member of the sialidase superfamily [PhD thesis]. Memphis (TN): Department of Genetics, St. Jude Children's Research Hospital; 2000.
- Schauer R. Sialic acids and their role as biological masks. Trends Biochem Sci. 1985;10(9):357–360.
- Schauer R. Sialic acids as regulators of molecular and cellular interactions. Curr Opin Struct Biol. 2009;19(5):507–514.
- Iijima R, Takahashi H, Namme R, et al. Novel biological function of sialic acid (N-acetylneuraminic acid) as a hydrogen peroxide scavenger. FEBS Lett. 2004;561(1-3):163–166.
- Chang Y, Uchiyama S, Varki A, et al. Leukocyte inflammatory responses provoked by Pneumococcal Sialidase. mBio. 2012;3(1):e00220-11.
- Roth J, Kempf A, Reuter G, et al. Occurrence of sialic acids in Drosophila melanogaster. Science. 1992;256(5057):673–675.
- Malykh YN, Krisch B, Gerardy-Schahn R, et al. The presence of N-acetylneuraminic acid in Malpigian tubules of larvae of the cicada Philaenus spumarius. Glycoconj J. 1999;16(11):731–739.
- Karaçalı S, Kırmızıgül S, Deveci R, et al. Presence of sialic acid in prothoracic glands of Galleria mellonella (Lepidoptera). Tissue Cell. 1997;29(3):315–321.
- Rottier PJM. The coronavirus membrane glycoprotein. In Siddell SG, editor. The coronaviridae. New York (NY): Plenum Press; 1995. p. 115–139.
- Banerjee N, Mukhopadhyay S. Viral glycoproteins: biological role and application in diagnosis. Virusdisease. 2016;27(1):1–11.
- Schauer R, Kamerling JP. The chemistry and biology of trypanosomal trans-sialidases: virulence factors in Chagas disease and sleeping sickness. Chembiochem. 2011;12(15):2246–2264.
- Wagstaff BA, Rejzek M, Field RA. Identification of a Kdn biosynthesis pathway in the haptophyte Prymnesium parvum suggests widespread sialic acid biosynthesis among microalgae. J Biol Chem. 2018;293(42):16277–16290.
- Zaretsky M, Roine E, Eichler J. Sialic acid-like sugars in Archaea: legionaminic acid biosynthesis in the halophile Halorubrum sp. PV6. Front Microbiol. 2018;9:2133.
- Alviano CS, Pereira MEA, Souza W, et al. Sialic acids are surface components of Sporothrix schenckii yeast forms. FEMS Microbiol Lett. 1982;15(3):223–227.
- Vásquez-del-Mercado E, Arenas R, Padilla-Desgarenes C. Sporotrichosis. Clin Dermatol. 2012;30(4):437–443.
- Souza ET, Silva-Filho FC, De Souza W, et al. Identification of sialic acids on the cell surface of hyphae and conidia of the human pathogen Fonsecaea pedrosoi. J Med Vet Mycol. 1986;24(2):145–153.
- Alviano DS, Rodrigues ML, Almeida CA, et al. Differential expression of sialylglycoconjugates and sialidase activity in distinct morphological stages of Fonsecaea pedrosoi. Arch Microbiol. 2004;181(4):278–286.
- Schauer R. Chemistry, metabolism, and biological functions of sialic acids. Adv Carbohydr Chem Biochem. 1982;40:131–234.
- Benhamou N, Ouellette GB. Ultrastructural localization of glycoconjugates in the fungus Ascocalyx abietina, the scleroderris canker agent of conifers, using lectin-gold complexes. J Histochem Cytochem. 1986;34(7):855–867.
- Benhamou N, Ouellete GB. Ultrastructural characterization of an extracellular fibrillar sneath on cells of Ascocalyx abietina, the scleroderris canker agent of conifers. Can J Bot. 1987;65(1):154–167.
- De Stefano J, Cushion M, Puvanesarajah V, et al. Analysis of Pneumocystis carinii cyst wall. II. Sugar composition. J Protozool. 1990;37(5):436–441.
- De Stefano JA, Trinkle LS, Walzer PD, et al. Flow cytometric analyses of lectin binding to Pneumocystis carinii surface carbohydrates. J Parasitol. 1992;78(2):271–280.
- Truong J, Ashurst JV. Pneumocystis (Carinii) Jiroveci Pneumonia. Treasure Island (FL): StatPearls; 2019. https://www.ncbi.nlm.nih.gov/books/NBK482370/
- Hamilton AJ, Jeavons L, Hobby P, et al. A34- to 38-Kilodalton Cryptococcus neoformans glycoprotein produced as an exoantigen bearing a glycosylated species-specific epitope. Infect Immun. 1992;60(1):143–149.
- Rodrigues ML, Rozental S, Couceiro J, et al. Identification of N-acetylneuraminic acid and its 9-O-acetylated derivative on the cell surface of Cryptococcus neoformans: influence on fungal phagocytosis. Infect Immun. 1997;65(12):4937–4942.
- Fan W, Kraus PR, Boily M, et al. Cryptococcus neoformans gene expression during murine macrophage infection. Eukaryot Cell. 2005;4(8):1420–1433.
- Rodrigues ML, Dobroff AS, Couceiro JN, et al. Sialylglycoconjugates and sialyltransferase activity in the fungus Cryptococcus neoformans. Glycoconj J. 2002;19(3):165–173.
- Soares RMA, Alviano CS, Angluster J, et al. Identification of sialic acids on the cell surface of hyphae and yeast forms of the human pathogen Paracoccidioides brasiliensis. FEMS Microbiol Lett. 1993;108(1):31–34.
- Alaei S, Larcher C, Ebenbichler C, et al. Isolation and biochemical characterization of the iC3b receptor of Candida albicans. Infect Immun. 1993;61(4):1395–1399.
- Wadsworth E, Prasad SC, Calderone R. Analysis of mannoproteins from blastoconidia and hyphae of Candida albicans with a common epitope recognized by anti-complement receptor type 2 antibodies. Infect Immun. 1993;61(11):4675–4681.
- Jones L, Hobden C, O’Shea P. Use of a real-time fluorescent probe to study the electrostatic properties of the cell surface of Candida albicans. Mycol Res. 1995;99(8):969–976.
- Wasylnka JA, Simmer MI, Moore MM. Differences in sialic acid density in pathogenic and non-pathogenic Aspergillus species. Microbiology (Reading)). 2001;147(Pt 4):869–877.
- Latgé JP. Aspergillus fumigatus and aspergillosis. Clin Microbiol Rev. 1999;12(2):310–350.
- Tiralongo J, Wohlschlager T, Tiralongo E, et al. Inhibition of Aspergillus fumigatus conidia binding to extracellular matrix proteins by sialic acids: a pH effect? Microbiology (Reading)). 2009;155(Pt 9):3100–3109.
- Wasylnka JA, Moore MM. Adhesion of Aspergillus species to extracellular matrix proteins: evidence for involvement of negatively charged carbohydrates on the conidial surface. Infect Immun. 2000;68(6):3377–3384.
- Warwas ML, Watson JN, Bennet AJ, et al. Structure and role of sialic acids on the surface of Aspergillus fumigatus conidiospores. Glycobiology. 2007;17(4):401–410.
- Li Y, Chen X. Sialic acid metabolism and sialyltransferases: natural functions and applications. Appl Microbiol Biotechnol. 2012;94(4):887–905.
- Lipničanová S, Chmelová D, Ondrejovič M, et al. Diversity of sialidases found in the human body – a review. Int J Biol Macromol. 2020;148:857–868.
- Vimr E, Lichtensteiger C. To sialylate, or not to sialylate: that is the question. Trends Microbiol. 2002;10(6):254–257.
- Lewis AL, Robinson LS, Agarwal K, et al. Discovery and characterization of de novo sialic acid biosynthesis in the phylum Fusobacterium. Glycobiology. 2016;26(10):1107–1119.
- Kim S, Oh DB, Kang HA, et al. Features and applications of bacterial sialidases. Appl Microbiol Biotechnol. 2011;91(1):1–15.
- Engibarov S, Eneva R, Abrashev I. Multifunctional enzymes related to sialic acid cleavage and transfer in microorganisms. Microbiology for a Better Health and Industry. 70th Anniversary of the Stephan Angeloff Institute of Microbiology. Bulgarian Acad Sci. 2017;6:115–120.
- Telford JC, Yeung JHF, Xu G, et al. The Aspergillus fumigatus sialidase is a 3-deoxy-d-glycero-d-galacto-2-nonulosonic acid hydrolase (KDNase): structural and mechanistic Insights. J Biol Chem. 2011;286(12):10783–10792.
- Yeung JHF, Telford JC, Shidmoossavee FS, et al. Kinetic and structural evaluation of selected active site mutants of the Aspergillus fumigatus KDNase (sialidase). Biochemistry. 2013;52(51):9177–9186.
- Uchida Y, Tsukada Y, Sugimori T. Production of microbial neuraminidases induced by colominic acid. Biochim Biophys Acta. 1974;350(2):425–431.
- Muller HE. Occurrence of neuraminidase in Sporothrix schenckii and Ceratocystis stenoceras and its role in ecology and pathomechanism of these fungi. Zentralbl Bacteriol Orig A. 1975;232(2-3):365–372.
- Nierman W, Pain A, Anderson M, et al. Genomic sequence of the pathogenic and allergenic filamentous fungus Aspergillus fumigatus. Nature. 2005;438(7071):1151–1156.
- Warwas ML, Yeung G, Indurugalla D, et al. Cloning and characterization of a sialidase from the filamentous fungus, Aspergillus fumigatus. Glycoconj J. 2010;27(5):533–548.
- Yeung JHF. Sialic acid metabolism in the opportunistic fungal pathogen, Aspergillus fumigatus [PhD thesis]. Canada: Simon Fraser University; 2015.
- Nesbitt JR. The role of AfKDNase in the growth and development of Aspergillus fumigatus [PhD thesis]. Canada: Simon Fraser University; 2017.
- Nesbitt JR, Steves EY, Schonhofer CR, et al. The Aspergillus fumigatus sialidase (KDnase) contributes to cell wall integrity and virulence in amphotericin T-treated mice. Front Microbiol. 2018;8:2706.
- Van Dijk A, Cyplenkova N, Dekker P, et al. Delft United States Patent Patent N0.2 US 8,012,733 B2, Sep. 6, 2011.
- Dekker P. Use of Sialidase in Dairy Technology United States Patent Application Publication.
- Sakarya S, Gunay N. Sacharomyces boulardii expresses neuraminidase activity selective for α2,3-linked sialic acid that decreases Helicobacter pylori adhesion to host cells. APMIS. 2014;122(10):941–950.
- Petit D, Teppa E, Cenci U, et al. Reconstruction of the sialylation pathway in the ancestor of eukaryotes. Sci Rep. 2018;8(1):2946.
- Abrashev R, Krumova E, Petrova P, et al. Distribution of a novel enzyme of sialidase family among native filamentous fungi. Fungal Biol. 2021; in press.
- Varki A, Angata T. Siglecs-the major subfamily of I-type lectins. Glycobiology. 2006;16(1):1R–27R.
- Corfield T. Bacterial sialidases-roles in pathogenicity and nutrition. Glycobiology. 1992;2(6):509–521.
- Hoyer LL, Roggentin P, Schauer R, et al. Purification and properties of cloned Salmonella typhimurium LT2 sialidase with virus-typical kinetic preference for sialyl alpha 2. J Biochem. 1991;110(3):462–467.
- Quistgaard EM, Thirup SS. Sequence and structural analysis of the Asp-box motif and Asp-box beta-propellers; a widespread propeller-type characteristic of the Vps10 domain family and several glycoside hydrolase families. BMC Struct Biol. 2009;9(46):46.
- Banerjee A, Van Sorge NM, Sheen TR, et al. Activation of brain endothelium by pneumococcal neuraminidase NanA promotes bacterial internalization. Cell Microbiol. 2010;12(11):1576–1588.
- Almagro-Moreno S, Boyd EF. Insights into the evolution of sialic acid catabolism among bacteria. BMC Evol Biol. 2009;9(118):118.