Abstract
Here we report the discovery and identification of antibacterial substances from the secondary metabolites of a new strain Bacillus velezensis BA-26. The whole genome of B. velezensis BA-26 was sequenced. Its genome data were annotated, and four potentially unknown gene clusters and nine known secondary metabolite synthetic gene clusters were analyzed and excavated by antiSMASH software. Based on the predicted secondary metabolites from the genome of strain BA-26, the material identification of isolated and purified extracellular secondary metabolites was conducted in combination with mass spectrometry data. A total of 24 antifungal compounds were identified, namely, iturin and various fengycins. The minimum inhibitory concentration (MIC) of iturin to Botrytis cinerea was 62.50 µg·mL−1. The MICs of C14 fengycin A, C16 fengycin A and C18 fengycin A were 62.50, 31.25 and 0.49 µg·mL−1, respectively, indicating that the increase in the number of carbon atoms in side chain fatty acids of fengycin improves its biological activity. Two kinds of anti-disease fungal compounds from B. velezensis BA-26, namely, iturin and fengycin, were purified and identified; they were found to have antibacterial activities against common pathogenic fungi. Therefore, B. velezensis BA-26 may be potentially used as a biological control agent. This study enriches the genome information on B. velezensis, elucidates the active components of B. velezensis BA-26 antibacterial substances, and provides a useful reference for using strain BA-26 as a biological control agent.
Supplemental data for this article is available online at https://doi.org/10.1080/13102818.2021.1934540 .
Introduction
Bacillus has a wide range of applications in medicine, food, agriculture, and industry because of its ability to produce a variety of extracellular bioactive substances (Citation1). Among these, many Bacillus strains have been developed into commercial-grade biological control agents (Citation2). The extracellular antibacterial active substances produced by Bacillus can be divided into antibacterial substances synthesized by the ribosomal pathway: bacteriocins, antibacterial proteins, chitinase, β-1,3-glucanase, and antibacterial substances synthesized by non-ribosomal pathways such as surfactins, iturin, fengycin and bacillaene (Citation3). Among these, bacteriocin and surfactins, iturin, fengycins and antibacterial proteins have been extensively characterized (Citation4). B. velezensis is a gram-positive aerobic bacillus that produces bioactive substances such as bacteriocins and lipopeptides that promote plant growth and inhibit a variety of microbial pathogens (Citation5,Citation6). Investigations on the molecular structure, functional characteristics and application of B. velezensis antibacterial substances have mainly focused on a variety of bacteriostatic active substances, whereas research on a single substance is limited (Citation5).
Recent studies have attempted to elucidate the antibacterial mechanism of B. velezensis using functional genomics analysis, using FZB42 as model strain (Citation7,Citation8). Genome mining of B. velezensis has identified secondary metabolite gene clusters of lipopeptides (Citation9). Genomic analysis and mass spectrometry have revealed extracellular secondary metabolites of B. velezensis 9 D-6 (Citation10). Various research studies have shown that a large number of lipopeptides appeared in the extracellular secondary metabolites of B. velezensis, which had broad-spectrum antifungal activity (Citation11,Citation12).
Bacillus lipopeptides are usually composed of three family compounds, including iturins, surfactins and fengycins (Citation12). Since iturin was isolated from B. subtilis, many lipopeptides belonging to the iturin family have been identified such as iturins A, B, C, D and E; bacillomycins D, F and L; and mycosubtilin (Citation13). Recent studies show that other strains that produce iturin family lipopeptides are Paenibacillus, B. velezensi and B. pumilus (Citation14,Citation15). The surfactin family has many different homologues and isomers, but all of these substances are made up of one hydroxyl fatty acid chain and an interconnected heptapeptide ring lactone structure (Citation16). Fengycin is also a lipopeptide synthesized by a non-ribosomal pathway, which is composed of a hydrophilic cyclic peptide, which includes 10 amino acids and a hydrophobic β-fatty acid chain (Citation17). Currently, there are four common types of fengycins, namely, A, B, A2 and B2. Their structural differences only involve a single amino acid (Citation18,Citation19). These reports are suggestive of an antibacterial synergistic effect among different types of lipopeptides (Citation20).
In our previous study, we determined that B. velezensis BA-26 has a strong fungal inhibitory effect and has a wide bacteriostatic spectrum (Citation21). The inhibitory effects of Fusarium sambucinum, Alternaria solani, Rhizoctonia solani, Botrytis cinerea, Botryosphaeria dothidea, Alternaria alternate and Aspergillus oryzae were very strong, but had no bacteriostatic effect on Staphylococcus aureus and Escherichia coli (Citation21). The strain BA-26 that we used in this study was isolated and identified in a previous work (Citation21). In this study, through whole-genome sequencing and bioinformatics analysis of B. velezensis BA-26, the secondary metabolites of the strain were predicted, and the unique secondary metabolite synthetic gene cluster of the strain was identified. Moreover, extracellular secondary metabolites were isolated, purified and identified using an ammonium sulfate precipitation method, reverse-phase high performance liquid chromatography (RP-HPLC) and Liquid Chromatography-Mass Spectrometry (LC-MS/MS), and the active components of strain BA-26 antifungal substances were identified. In addition, the bacteriostatic effect of antifungal compounds was assessed and its underlying mechanism improved, thereby providing a theoretical basis for potential agricultural applications.
Materials and methods
Currently, we determined that B. velezensis BA-26 has a strong fungal inhibitory effect, but we have not identified which secondary metabolites work. Therefore, we performed whole-genome sequencing on strain BA-26 to obtain whole-genome data, and perform gene annotation on the genome data. On the basis of genomic data, we used antiSMASHv4.2 software to analyze the secondary metabolites contained in synthetic gene clusters and predicted the antibacterial substances. Then, the antibacterial substances in its extracellular secondary metabolites were separated, purified and identified to verify the predicted antibacterial substances.
Strain and culture conditions
B. velezensis BA-26 is an antagonistic strain isolated from the hillside of Zhangshiyan by our laboratory. The BA-26 strain was identified as B. velezensis by morphological, physiological and biochemical characterization and 16S rDNA sequence analysis (Citation22). The indicator fungi was Botrytis cinerea, which is maintained at the Microbiology Laboratory of the Academy of Sciences of Hebei Province, China. Strain BA-26 was cultured in NB liquid medium (containing 10 g·L−1 tryptone, 5 g·L−1 beef extract, 10 g·L−1 glucose, and 5 g·L−1 NaCl in distilled water) at 32 °C for 48 h and 180 rpm continuous shaking.
DNA extraction, genome sequencing and assembly
Genomic DNA of B. velezensis BA-26 was extracted using a bacterial genome kit (TIANGEN, Beijing, China). A 10-kb double-stranded DNA fragment was selected to construct the SMRTbell DNA library for sequencing. DNA sequencing was performed by Suzhou Jinweizhi Biotechnology Co., Ltd., and the whole genome of B. velezensis BA-26 was sequenced using an Illumina HiSeq 2500 system and the third-generation high-throughput Pacbio SMRT sequencing technique. The quality statistics software cutadapt v1.9.1 was used to optimize the removal of connectors and low-quality sequences of the second-generation sequencing raw data (Citation23). The preliminary assembly results were obtained based on the three-generation sequencing data using the software HGAP4 v4.0 and Falcon v0.3. The quality-filtered second-generation sequencing data were compared to the preliminary assembly results, and the assembly results were further corrected using the software Pilon v1.2.2 to obtain the final assembly results (Citation23).
Genome annotation
Based on the predicted protein sequence, using BLAST (Citation24); the E-value of the sequence alignment was set to 1 × e−5, and the sequence alignment length to > 60% of the protein length. The optimal matching results were selected as the annotation results of the gene. The NR (Citation25), GO (Citation26), KEGG (Citation27), COG (Citation28) and CAZy (Citation29) databases were used in functional annotation of genes. Prediction and analysis of gene clusters encoding secondary metabolites in B. velezensis BA-26 were performed using the online software antiSMASH v4.2 (Citation30).
Separation and purification of antifungal secondary metabolites
The fermentation broth cultured with strain BA-26 for 48 h was centrifuged at 10,000 g for 30 min, and ammonium sulfate solid was added to the cell-free supernatant to reach 60% saturation, left to stand at 4 °C for 12 h, and then centrifuged at 8,000 g for 30 min to collect the precipitate. Then, the precipitate was dissolved in 5 mL of sterile water, and bacteriostatic activity was assessed. Here, the precipitate represented the antibacterial crude extract, which was further separated and purified using macroporous resin XAD-7HP (Citation31) and washed with distilled water thrice. The samples were extracted at 42 °C in a rotary evaporator and stored at 4 °C until use.
The sample was further purified by RP-HPLC (SHIMADZU LC-20AT, Japan). Mobile phase A was HPLC-grade water containing 0.1% (volume ratio) trifluoroacetic acid, and mobile phase B was acetonitrile containing 0.1% (volume ratio) trifluoroacetic acid. The macroporous resin XAD-7HP separation samples were loaded into a C18 column (250 × 4.6 mm, 5 μm; WONDASIL, Japan), a 10%–90% acetonitrile linear gradient was employed for elution (0–60 min), 90% acetonitrile for isometric elution (60–66 min), 90%–10% acetonitrile linear gradient for elution (66–68 min), and 10% acetonitrile for isometric elution (68–78 min) at a flow rate of 1 mL·min−1, a detection wavelength of 214 nm, and column temperature at 30 °C. The corresponding components per minute were collected, and the components with antifungal activity were further screened for structural identification.
LC-MS/MS analysis
The samples with antifungal activity were analyzed by mass spectrometry using an XEVO-G2-Q-TOF-MS system (Waters Company). The conditions of UPLC were as follows: mobile phase A was acetonitrile containing 0.1% (volume ratio) formic acid, and mobile phase B was HPLC-grade water containing 0.1% (volume ratio) formic acid, Waters UPLC HSS T3 (C18; 2.1 mm × 100 mm, 1.7 µm), 10%–65% acetonitrile linear gradient for elution for 3 min, and 65%–100% acetonitrile linear gradient for elution for 3 min. The injection volume is 5.0 μL, the flow rate was 0.3 mL·min−1, the detection wavelength was 214 nm, and column temperature was 40 °C. The mass scanning range was 50–4,000 M/Z, the capillary voltage was 3,000 V, the desolvent temperature was 400 °C, nitrogen was used in all kinds of gas paths, positive ion mode analysis was employed, and Masslyxn data management software was utilized for data acquisition.
MIC analysis
Four kinds of high-purity antifungal compounds (C15 iturin A, C14 fengycin A, C16 fengycin A, and C18 fengycin A) were isolated and purified for MIC analysis of B. cinerea. Four antifungal compounds with concentrations of 0.24, 0.49, 0.98, 1.95, 3.91, 7.81, 15.63, 31.25, 62.50, 125.00, 250.00 and 500.00 µg·mL−1 were prepared in sterile water using the double dilution method (Citation32), and 100 μL each was added to the agar pore. After culturing at a constant-temperature incubator at 26 °C for 48 h, the half diameter of the inhibition area was measured to determine the inhibitory effect on B. cinerea. We used 70% aseptic nitrile aqueous solution as control, and each group was repeated thrice.
Results
Genomic characteristics of B. velezensis BA-26
The whole genome of strain BA-26 was sequenced using the Illumina HiSeq 2500 system and the PacBio SMRT sequencing technique, resulting in 11,213,428 reads for 1,682,014,200 total bases, with approximately 416-fold coverage. There are 4,035,062-bp in the complete genome circular chromosomes of strain BA-26, with a GC content of 46.41% ( and ). The genome sequence was predicted to comprise 3,927 encoding genes, of which 3,663 were protein-coding genes (CDSs), 89 were rRNA operon genes, and 86 were tRNA genes. In addition, the repeat sequences in the genome were predicted from scratch, and three long terminal repeats, 22 long scattered repeats, 5 short scattered repeats, and 83 simple repeats were detected. No plasmid sequence was identified by genome assembly. Approximately 3,433 protein-coding genes in strain BA-26 have COG numbers. They are divided into five functional classes in the COG database and are mainly related to the transport and metabolism of carbohydrates, amino acid transport, and metabolism and transcription (). The complete genome sequence of B. velezensis BA-26 has been submitted to GenBank under the accession number CP046918.
Figure 1. Genome circle map (A) and COG function annotation (B) of B. velezensis BA-26. (A) The circle map shows seven kinds of information from the outside to the inside: circle 1 is genome location information, circle 2 is GC content information, circle 3 is coding gene on positive chain (red label), circle 4 is coding gene on negative chain (green label), circle 5 is ncRNA information on the positive chain (blue mark), circle 6 is ncRNA information on the negative chain (purple label), and circle 7 marks the information of long fragment repeats (≥ 100 bp) in the genome (orange tagging). (B) Functional classification of COG genes of strain BA-26.
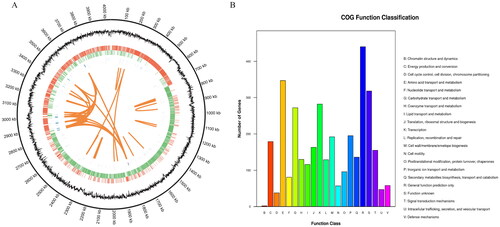
Table 1. Genomic characteristics of B. velezensis BA-26.
Functional annotation of genes using KEGG
The B. velezensis BA-26 genome was annotated by the KEGG database, and a histogram was obtained according to the secondary classification and statistics of KEGG. The results showed that the genome of the strain BA-26 had the highest number of metabolism-related genes (1,762), environmental information processing-related genes (291), genetic information processing-related genes (233) and cell process-related genes (87) (). The statistical results showed that various substance metabolism pathways and energy metabolism pathways provide the necessary nutrients and energy supply for the growth of the strain. There are many genes that are related to lipid metabolism, i.e. terpenoids and polyketones metabolized by strain BA-26, and there are many genes involved in the biosynthesis of other secondary metabolites, indicating that the strain may have the potential to synthesize a variety of lipopeptide substances, although it does not rule out the possibility of discovering other novel secondary metabolites.
Functional annotation of genes using GO
GO is a database of annotation and classification of gene and protein functions for a variety of species. According to the properties of the encoded products (), the functions of sequenced genes could be divided into three categories: biological process, molecular function and cellular component. Metabolic and cellular processes play a major role in the biological processes of strain BA-26. Binding and catalytic activity functions have obvious advantages in the functional category of molecular function. Cell parts and membrane gene synthesis are major aspects of the cellular component functional category. The results () showed that 80% of the annotated genes may be involved in the synthesis and transport of secondary metabolites. Combined with the results of functional gene annotation, we elucidated the genomic basis by which strain BA-26 synthesizes a variety of secondary metabolites.
Functional annotation of genes using CAZy
CAZy is a database of enzymes related to the degradation, synthesis and modification of carbohydrates that describes the catalytic structure of the strain as well as functional information. We used the CAZy database to annotate the genes in the B. velezensis BA-26 genome. The results showed () that the number of glycosyltransferases (GT) which was noted in the genome of strain BA-26, was 256, and there are 164 genes encoding glycoside hydrolases (GHs), and 91 genes encoding carbohydrate-binding modules (CBMs). There are 35 genes encoding carbohydrate esterases (CEs), 11 genes related to auxiliary activities (AAs), and the polysaccharin lyases (PLs) are encoded by at least three genes. The metabolism of substances in the organism is achieved by a variety of special functions. As can be seen from the above statistical data, the GTs, GHs and CBM-related enzymes occupy a larger proportion and are involved in the synthesis of the secondary metabolites of the non-ribosomal pathway.
Prediction and identification of the gene cluster of secondary metabolites
The presence of 13 secondary metabolite gene clusters in the genome of B. velezensis BA-26 was predicted by the online software antiSMASH v4.2 (Supplemental Table S1). According to the differences in gene clusters encoding secondary metabolite synthases, there are five categories: transatpks, NRPS, terpenes, PKS and Other. Six gene clusters were identical to those known in macrolactin, bacillaene, fengycin, difficidin, bacillibactin and bacilysin, all showing 100% similarity. Clusters 11, 10 and 12 were similar to known clusters, namely, surfactin (91% similarity), rhizocticin (22% similarity) and butirosin (7% similarity). In addition, four new clusters, namely, Clusters 4, 5, 8 and 13 were predicted, and no known clusters were matched. In conclusion, there are many kinds of antibacterial substances produced by strain BA-26, especially common lipopeptide substances, which can be studied in the next step with reference to the method of separation and purification of these substances.
Purification of antifungal compounds and LC-MS/MS analysis
The cell-free supernatant of strain BA-26 was precipitated with ammonium sulfate and purified by macroporous resin XAD-7HP, and the crude antifungal extract was obtained. The crude antifungal extract was isolated by RP-HPLC, and 11 kinds of active components with antifungal effect were identified. The purified components had strong antifungal activity against B. cinerea. To determine the structure of each active substance and find new antibacterial substances, these substances were identified by LC-MS/MS (Citation32). The results showed that there were 24 kinds of antifungal compounds in the extracellular active substances of strain BA-26, all of which were lipopeptides. Compared with previous studies (Citation33), they are iturins and various fengycins (Supplemental Table S2).
For iturin by secondary mass spectrometry (), the molecular ion peaks [M + H]+ (m/z 1057.5698), [M + Na]+ (m/z 1079.5515) and [M + K]+ (m/z 1095.5308) were detected, and their molecular weight was determined to be 1,056. These results were compared to the reported iturin data (Citation34) and identified as C15 iturin A.
Figure 5. LC-MS/MS analysis of iturin and fengycin A. C15 iturin A (A) and fengycin A (B–F) series compounds were identified by mass spectrometry and both showed strong antifungal activity against B. cinerea.
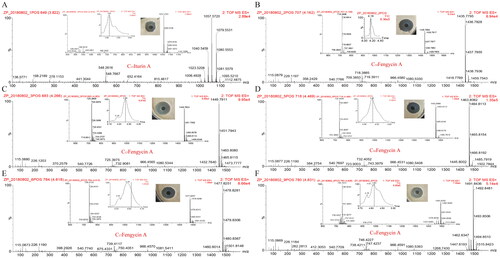
In this study, 23 fengycin substances were detected within the m/z range of 1,435–1,520 (Supplemental Table S2). Fengycins A and B differed in structure, and the sixth amino acids of their cyclic peptides were Ala (89.1 Da) and Val (117.1 Da), respectively (Citation19). Therefore, in the process of mass spectrometry, different fragment ion peaks were observed. The fragment ion peaks m/z (966/1,080) and m/z (994/1,108) are usually utilized as characteristic fragment ions to identify the two lipids fengycins A and B (Citation19). By mass spectrometry analysis, a total of five fragment ion peaks [M + H]+ (1,435.7806, 1,449.7924, 1,463.8096, 1,477.8257 and 1,491.8452) were detected. They all had characteristic fragment ion peaks m/z (966/1,080), which were identified as C14 fengycin A, C15 fengycin A, C16 fengycin A, C17 fengycin A and C18 fengycin A (). Similarly, fragment ion peaks [M + H]+ (1,449.7905, 1,463.8070, 1,477.7845, 1,441.8416, 1,505.8545 and 1,519.8677) were detected by mass spectrometry. They all had characteristic fragment ion peaks m/z (994/1108), which were identified as C13 fengycin B, C14 fengycin B, C15 fengycin B, C16 fengycin B, C17 fengycin B and C18 fengycin B (). In addition, fragment ion peaks m/z (952/1,066) and m/z (980/1,094) were detected during secondary mass spectrometry as characteristic fragment ions in the identification of fengycin subtypes (fengycins A2 and B2) (Citation19). The characteristic fragment ion peak m/z (952/1,066) was detected at fragment ion peak [M + H]+ (1,435.7767), which was identified as C14 fengycin A2 (), and the characteristic fragment ion peak m/z (980/1,094) was detected at fragment ion peak [M + H]+ (1,449.7965, 1,463.8070, 1,477.8143 and f1,491.8419), which were C13 fengycin B2, C14 fengycin B2, C15 fengycin B2 and C16 fengycin B2, respectively (). Compared with the results of previous studies, it was found that there were also unsaturated fatty acids in the fengycin family (Citation19, Citation33). Among these, C15:1 fengycin A, C15:1 fengycin A2, C17:1 fengycin A, C16:1 fengycin A, C14:1 fengycin B2, C15:1 fengycin B and C16:1 fengycin B () were compared to known fengycins, their mass decreased by 2 Da, and unsaturated double bonds were detected (Citation35).
MIC of iturins and fengycins against B. cinerea
MIC analysis was performed on the lipopeptide antifungal compounds iturin and fengycin that were isolated and purified from strain BA-26. The MIC of iturin was 62.50 µg·mL−1 (Supplemental Table S3). The results of MIC and determination of three different fengycin substances showed that the MIC of all fengycin substances to B. cinerea was ≤ 62.50 μg·mL−1 (Supplemental Table S3). Comparison of the MICs of the three kinds of fengycin (C14 fengycin A, C16 fengycin A and C18 fengycin A) against B. cinerea revealed a gradual increase in their antifungal activities.
Discussion
In recent years, the newly proposed B. velezensis has gradually increased in the natural environment (Citation11,Citation12), and in addition to its practical application as biocontrol agents, the active substances produced by B. velezensis strains have anti-bacterial, anti-fungal and anti-viral properties (Citation5). As of November 2019, all or part of the gene sequencing data of 263 B. velezensis have been submitted to the NCBI database. The genome sequencing data of B. velezensis continue to increase, and genome mining has facilitated the identification of antibacterial substance synthesis-related gene clusters that may be utilized in the development of various antibacterial substances (Citation9,Citation10). Therefore, genome sequencing and analysis of the novel strain B. velezensis BA-26 is essential and may allowthe identification of novel gene clusters related to the synthesis of secondary metabolites.
Whole-genome sequencing of B. velezensis BA-26 has identified 136 genes that are related to the biosynthesis, transport and catabolism of secondary metabolites, which is greater than that in B. amyloliquefaciens WS-8 (110 genes) (Citation35) and B. subtilis BSD-2 (98 genes) (Citation36). The antibacterial substance genes detected in B. amyloliquefaciens WY047 are fenA, ituA, bmyD, hag, mrsA and tasA (Citation37). So far, it has been reported that most Bacillus strains produce not more than three or four antibacterial compounds (Citation38). Using the online software antiSMASH v4.2, we identified nine gene clusters that are similar to known active substances in strain BA-26, as well as four potentially unknown gene clusters of secondary metabolites. Two kinds of lipopeptide biosynthesis-related gene clusters (surfactin, fengycin) were detected in the genome of strain BA-26, similar to many other Bacillus that could produce a large number of lipopeptides (Citation11). In this study, two antifungal compounds, namely, iturin and fengycin, were purified and identified in strain BA-26. Together with the fengycin gene clusters identified in the genome of strain BA-26, we hypothesize that iturins might be one of the four unidentified secondary metabolites.
Eleven antifungal components were isolated by RP-HPLC, and LC-MS/MS identification showed that there were 24 kinds of antifungal compounds (Supplemental Table S1), which were largely iturins and fengycins, and both had strong antagonistic effects on B. cinerea. Generally speaking, the antifungal activity of fengycins is stronger than iturins, whereas its hemolytic effect is weak (Citation39). Fengycins were first reported in B. subtilis (Citation40) and have been shown to induce plant immune responses (Citation41). A variety of fengycin variants have been identified based on the four heterogeneous sites existing in the fengycin family (Citation19). In this study, in the fengycin family that was identified in the m/z 1,435–1,520 range, we detected four subtypes of fengycin, namely, A, B, A2 and B2. By comparing the ion peaks of protonated molecules observed by mass spectrometry, we found that the fengycin class had a molecular mass difference of 14 Da and with a chain length varying from C13 to C18. Similar to previous studies, the presence of unsaturated fatty acids in some fengycins with chain lengths ranging from C14 to C17 resulted in a 2-Da reduction in molecular weight (Citation19, Citation42). It has been reported that there are also unsaturated double bonds in many fengycin derivatives (Citation33). Liu et al. found that the main active substances in B. amyloliquefaciens WS-8 were iturin and fengycin (Citation35). In this context, our study elucidated the active components of the antifungal substance of strain BA-26.
A previous study has shown that the antifungal compounds of strain BA-26 had a strong inhibitory effect on 12 pathogenic fungi (Citation21). In this study, the MICs of iturin and fengycin that were isolated and purified from the strain BA-26 were determined, and both substances showed strong antifungal activity compared with other studies (Citation32). In addition, the antifungal activity of fengycin was higher than that of iturin analogues (Supplemental Table S3), which was similar to the findings of a previous report (Citation18). At least for surfactins, it is known that increasing the acyl chain length results in stronger bilayer solubilization and membrane activity (Citation43). For iturins, the antifungal activity is generally enhanced with increasing number of carbon atoms on the fatty acid side chains, presumably due to stronger interactions with biomembranes (Citation44). By comparing the MICs of C14 fengycin A, C16 fengycin A and C18 fengycin A against B. cinerea, we determined that the increase in the number of side chain fatty acid carbon atoms in fengycin increases their biological activity. To our knowledge, this is the first report on the comparison of antifungal activity of different fengycin substances. Some studies have shown that the pairwise combinations of different types of lipopeptide, such as surfactants, iturins and fengycins, also have antifungal synergistic effects, although their combined application for prevention and treatment requires further investigation (Citation20).
In conclusion, based on the measured whole genome data of B. velezensis BA-26, 13 secondary metabolite synthetic gene clusters were identified by bioinformatics analysis, of which 4 belonged to potentially unknown gene clusters. In addition, 24 antifungal compounds were identified by mass spectrometry, and the structures of iturins and antifungal compounds of fengycin derivatives were revealed. In this study, by determining the MIC of iturin and different fengycin substances on B. cinerea, we determined that the antifungal substances derived from strain BA-26 had obvious inhibitory effects on pathogenic fungi, indicating that strain BA-26 could be potentially used as an effective biological control agent. In the future, the synergistic antifungal effects of iturins and fengycins can also be studied to provide a reference for the combined control of various antifungal substances.
Author contributions
L.H.W and Z.L.P designed this experiment. W.B.Q, L.C, L.H.W, Y.X.M, W.Y.N, Z.F.Y, and C.H.C executed the experiment. W.B.Q, L.H.W and L.C performed the analysis and wrote the paper. All the authors have read and approved the final manuscript.
Supplemental Material
Download PDF (1.9 MB)Acknowledgments
We thank LetPub (www.letpub.com) for its linguistic assistance during the preparation of this manuscript.
Disclosure statement
The authors have no financial conflicts of interest to declare.
Data availability
All data that support the findings from this study are available from the corresponding author (…) upon reasonable request.
Additional information
Funding
References
- Meena K, Kanwar S. Lipopeptides as the antifungal and antibacterial agents: applications in food safety and therapeutics. BioMed Res Int. 2015;2015:473050.
- Droby S, Wisniewski M, Teixidó N, et al. The science, development, and commercialization of postharvest biocontrol products. Postharvest Biol Technol. 2016;122:22–29.
- Steller S, Vollenbroich D, Leenders F, et al. Structural and functional organization of the fengycin synthetase multienzyme system from Bacillus subtilis b213 and A1/3. Chem Biol. 1999;6(1):31–41.
- Jacques P. Surfactin and Other Lipopeptides from Bacillus spp. Berlin Heidelberg: Springer; 2010.
- Rabbee M, Ali M, Choi J, et al. Bacillus velezensis: A Valuable Member of Bioactive Molecules within Plant Microbiomes. Molecules (Basel, Switzerland). 2019;24(6):1046.
- Schofield BJ, Skarshewski A, Lachner N, et al. Near complete genome sequence of the animal feed probiotic, Bacillus amyloliquefaciens H57. Genomic Sci. 2016;11(1):60.
- Chen X, Koumoutsi A, Scholz R, et al. Comparative analysis of the complete genome sequence of the plant growth-promoting bacterium Bacillus amyloliquefaciens FZB42. Nat Biotechnol. 2007;25(9):1007–1014.
- Fan B, Wang C, Song X, et al. Bacillus velezensis corrigendum: FZB42 in 2018: The Gram-positive model strain for plant growth promotion and biocontrol. Front Microbiol. 2019;10:1279.
- Adeniji A, Aremu O, Babalola O. Selecting lipopeptide-producing, Fusarium-suppressing Bacillus spp.: Metabolomic and genomic probing of Bacillus velezensis NWUMFkBS10.5. Microbiol Open. 2019;8:e00742.
- Grady E, MacDonald J, Ho M, et al. Characterization and complete genome analysis of the surfactin-producing, plant-protecting bacterium Bacillus velezensis 9D-6. BMC Microbiol. 2019;19(1):5.
- Cao Y, Pi H, Chandrangsu P, et al. Antagonism of two plant-growth promoting Bacillus velezensis isolates against Ralstonia solanacearum and Fusarium oxysporum. Sci Rep. 2018;8(1):4360.
- Liu Y, Teng K, Wang T, et al. Antimicrobial Bacillus velezensis HC6: production of three kinds of lipopeptides and biocontrol potential in maize. J Appl Microbiol. 2020;128(1):242–254.
- Carrillo C, Teruel J, Aranda F, et al. Molecular mechanism of membrane permeabilization by the peptide antibiotic surfactin. Biochim Biophys Acta. 2003;1611(1–2):91–97.
- Chung Y, Kim C, Hwang I, et al. Paenibacillus koreensis sp. nov., a new species that produces an iturin-like antifungal compound. Int. J. Syst. Evol. Microbiol. 2000;50(4):1495–1500.
- Yu GY, Sinclair JB, Hartman GL, et al. Production of iturin A by Bacillus amyloliquefaciens suppressing Rhizoctonia solani. Soil Biol Biochem. 2002;34:0–963.
- Angelini T, Roper M, Kolter R, et al. Bacillus subtilis spreads by surfing on waves of surfactantProc Natl Acad Sci USA 2009;106:18109–18113.
- Nishikiori T, Naganawa H, Muraoka Y, et al. Plipastatins: new inhibitors of phospholipase A2, produced by Bacillus cereus BMG302-fF67, III. Structural elucidation of plipastatins. J Antibiot. 1986;39:755–761.
- Ongena M, Jourdan E, Adam A, et al. Surfactin and fengycin lipopeptides of Bacillus subtilis as elicitors of induced systemic resistance in plants. Environ Microbiol. 2007;9(4):1084–1090.
- Pathak KV, Keharia H, Gupta K, et al. Lipopeptides from the banyan endophyte, Bacillus subtilis K1: mass spectrometric characterization of a library of fengycins. J Am Soc Mass Spectrom. 2012;23(10):1716–1728.
- Blée E. Impact of phyto-oxylipins in plant defense. Trends Plant Sci. 2002;7(7):315–322.
- Liu C, Liu HW, Wang BQ, et al. Isolation of antifungal substances from Bacillus amyloliquefaciens BA-26 and its antifungal activity against Botrytis cinerea. Biotechnol Bull. 2019a;35:83–89.
- Yang F, Zhang FY, Sun JC, et al. Identification and preliminary application of antagonistic strains of potato fungal diseases. China Veg. 2019;11:56–62.
- Li H, Durbin R. Fast and accurate long-read alignment with Burrows-Wheeler transform. Bioinformatics (Oxford, England). 2010;26(5):589–595.
- van HA, Jong Ad, Montalbán-López M, et al. BAGEL3: Automated identification of genes encoding bacteriocins and (non-)bactericidal posttranslationally modified peptides. Nucleic Acids Res. 2013;41:W448–453.
- Ashburner M, Ball C, Blake J, et al. Gene ontology: tool for the unification of biology. The Gene Ontology Consortium. Nat Genet. 2000;25(1):25–29.
- Consortium GO. Gene Ontology Consortium: going forward. Nucleic Acids Res. 2015;43:D1049–1056.
- Kanehisa M, Goto S, Sato Y, et al. Data, information, knowledge and principle: back to metabolism in KEGG. Nucl Acids Res. 2014;42(D1):D199–205.
- Tatusov R, Fedorova N, Jackson J, et al. The COG database: an updated version includes eukaryotes. BMC Bioinf. 2003;4(1):41.
- Wang S, Chen GJ, Zhang HQ, et al. Carbohydrate-active enzyme (CAZy) database and its new prospect. Chin J Bioprocess Eng. 2014;12:102–108.
- Blin K, Wolf T, Chevrette M, et al. antiSMASH 4.0-improvements in chemistry prediction and gene cluster boundary identification. Nucleic Acids Res. 2017;45(W1):W36–W41.
- Xin B, Zheng J, Xu Z, et al. Three novel lantibiotics, Ticins A1, A3, and A4, have extremely stable properties and are promising food biopreservatives. Appl Environ Microbiol. 2015;81(20):6964–6972.
- Xu BH, Lu YQ, Ye ZW, et al. Genomics-guided discovery and structure identification of cyclic lipopeptides from the Bacillus siamensis JFL15. PloS One. 2018;13(8):e0202893.
- Chen MC, Wang JP, Zhu YJ, et al. Antibacterial activity against Ralstonia solanacearum of the lipopeptides secreted from the Bacillus amyloliquefaciens strain FJAT-2349. J Appl Microbiol. 2019;126(5):1519–1529.
- Pathak K, Keharia H. Characterization of fungal antagonistic bacilli isolated from aerial roots of banyan (Ficus benghalensis) using intact-cell MALDI-TOF mass spectrometry (ICMS). J Appl Microbiol. 2013;114(5):1300–1310.
- Liu HW, Wang YN, Yang QX, et al. Bacillus amyloliquefaciens Genomics and LC-MS reveal diverse active secondary metabolites in WS-8. J Microbiol Biotechnol. 2019b;30(3):417–426.
- Liu HW, Yin S, An LK, et al. Complete genome sequence of Bacillus subtilis BSD-2, a microbial germicide isolated from cultivated cotton. J Biotechnol. 2016;230:26–27.
- Han Y, Li XX, Guo YY, et al. Co-production of multiple antimicrobial compounds by Bacillus amyloliquefaciens WY047, a strain with broad-spectrum activity. Trans Tianjin Univ. 2018;24(2):160–171.
- Mora I, Cabrefiga J, Montesinos E. Antimicrobial peptide genes in Bacillus strains from plant environments. Int Microbiol. 2011;14:213–223.
- Aranda FJ, Teruel JA, Ortiz A. Further aspects on the hemolytic activity of the antibiotic lipopeptide iturin A. Biochim Biophys Acta. 2005;1713:51–56.
- Vanittanakom N, Loeffler W, Koch U, et al. Fengycin–a novel antifungal lipopeptide antibiotic produced by Bacillus subtilis F-29-3. J Antibiot. 1986;39(7):888–901.
- Liu J, Zhou T, He D, et al. Functions of lipopeptides bacillomycin D and fengycin in antagonism of Bacillus amyloliquefaciens C06 towards Monilinia fructicola. J Mol Microbiol Biotechnol. 2011;20(1):43–52.
- Kim P, Bai H, Bai D, et al. Purification and characterization of a lipopeptide produced by Bacillus thuringiensis CMB26. J Appl Microbiol. 2004;97(5):942–949.
- Francius G, Dufour S, Deleu M, et al. Nanoscale membrane activity of surfactins: influence of geometry, charge and hydrophobicity. Biochim Biophys Acta Biomembr 2008;1778:2058–2068.
- Cho KM, Math RK, Hong SY, et al. Iturin produced by Bacillus pumilus HY1 from Korean soybean sauce (kanjang) inhibits growth of aflatoxin producing fungi. Food Control. 2009;20(4):402–406.