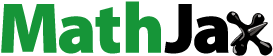
ABSTRACT
In previous study, we identified a novel protein elicitor AMEP412 from Bacillus subtilis BU412, which could trigger plant defense response and induce systemic acquired resistance against diseases. In this paper, the production of the new elicitor was optimised. First, optimal carbon source and nitrogen source were determined as glucose and yeast extract by single factor test. Then, effects of seven different factors on AMEP412 production was studied by Plackett-Burman design. Significant factors (yeast extract, culture temperature and culture time) were selected for further optimisation using central composite design by response surface methodology. The optimum conditions for AMEP412 production were yeast extract 21.72 g/L, culture temperature 30.84 °C and culture time 31.84 h. Under these conditions, the yield of AMEP412 was enhanced from 0.32 to 2.39 mg/mL (7.47-fold), which was quite close to the predicted one. This result significantly raised the yield of AMEP412, which laid solid foundation for its application as a biocontrol agent.
Introduction
Protein elicitors, produced and released by microbes, are regarded as ideal biocontrol candidates for the ability of triggering plant immunity [Citation1–3]. AMEP412 was a novel protein elicitor identified from Bacillus subtilis BU412, which showed good ability in triggering plant defense response and promoting plant resistance [Citation4]. In the identification process, we found that the production of AMEP412 was seriously affected by the fermentation conditions. When B. subtilis BU412 was cultured in Luria-Bertrani medium, the yield of AMEP412 was too low to detect. However, when YME medium [Citation5] was used for culture, the yield of AMEP412 increased greatly, which facilitated its purification and identification. This result suggested that the production parameters of AMEP412 deserved further investigation.
To achieve the maximum potential of a microorganism for the synthesis of a desired product, it is very important to optimise the cultural parameters. Statistical experimental design method is a powerful tool to improve the efficiency of optimisation. The single-factor-at-a-time strategy involves varying the level of one parameter at a time over a certain range while holding the rest of the test variables constant, which can only be used in one-factor optimisation [Citation6]. For experiments containing more than five factors, Plackett-Burman design (PBD) was employed to screen the most significant factors [Citation7]. However, it could not consider the interactions between the factors [Citation8]. Response surface methodology (RSM) is a powerful technique for optimising the processes that are influenced by many factors and their interactions [Citation9]. To our knowledge, RSM had been widely used for optimising the production from metabolisms of Bacillus strains. The yield of enzymes like Nattokinase [Citation10] and α-amylase [Citation11] were promoted by RSM based optimisation using Bacillus strains. Furthermore, the production of small peptides like Iturin A [Citation12] and Fengycin [Citation13] were also developed. Similar with above metabolisms, AMEP412 was also produced by Bacillus strain, so its production could possibly be enhanced by RSM.
In this study, we discussed the screening of appropriate medium components and culture conditions for the production of AMEP412 from B. subtilis BU412.Optimal carbon and nitrogen sources were determined by single factor experiments. PBD was executed to select the significant fermentation factors. RSM was carried out through the central composite design (CCD) to investigate the levels of the significant factors influencing production.
Materials and methods
Production and measurement of AMEP412
Before optimisation, the production of AMEP412 was executed as follows. For seed culture preparation, a loop full of the stock culture of B. subtilis BU412 was inoculated in 100 mL shake-flask containing 50 mL of YME medium (yeast extract 4 g/L, malt extract 10 g/L, dextrose 4 g/L, pH 7.0) and the flask was incubated in rotary shaker for 24 h at 37 °C and 160 rpm. Then 3 mL of the seed was inoculated into 1 L shake-flask containing 300 mL of YME medium and incubated under the same conditions.
AMEP412 was isolated by serials of purification steps from the supernatant of B. subtilis BU412 culture following previous method [Citation4]. Bacillus subtilis BU412 culture was centrifuged to obtain the cell-free culture supernatant. The supernatant was filtered through a 0.22 μm membrane to remove residues and then applied to AKTA Purifier system (Amersham Biosciences). The purification procedures included anion exchange chromatography and size exclusion chromatography, using Source 15Q 4.6/100 PE column and Superdex 75 10/300 GL column. The fraction of the target protein was collected and its concentration was measured through NanoDrop One UV Spectrophotometer (Thermo Scientific).
Selection of carbon and nitrogen sources
Preliminary screening of different carbon and nitrogen sources was carried out using a one factor at-a-time method. The carbon sources (malt extract and dextrose) in YME medium were replaced with different carbon sources (sucrose, glucose, mannitol, maltose and lactose) (0.4%, wt/vol) for optimisation, respectively, with yeast extract (0.4%, wt/vol) as nitrogen source. Similarly, the impact of different nitrogen sources ((NH4)2SO4, soybean tryptone, yeast extract, urea, peptone and NH4Cl) (0.4%, wt/vol) were also tested by replacing malt extract and yeast extract, with glucose (0.4%, wt/vol) as carbon source. These medium combinations were cultured under the same condition mentioned above, and the protein yield results were analysed for best carbon and nitrogen sources.
Selection of significant factors by PBD
The most important factors that significantly influence AMEP412 production were selected by the PBD experiment. A total of seven parameters were included for selection, with each factor represented at two widely spaced intervals designated as −1 (low level) and +1 (high level). The factors were as follows: (1) carbon source, (2) nitrogen source, (3) initial pH, (4) culture time, (5) culture temperature, (6) rotation speed and (7) inoculum size. The experimental design for the screening of the factors was denoted in .
Table 1. PBD for the screening of significant factors affecting AMEP412 production.
Optimisation of AMEP412 production by RSM
RSM based on a CCD was implemented to scrutinise the most optimum level, impact and interactions of three factors such as yeast extract (A), culture temperature (B) and culture time (C) on AMEP412 production. The factors were assessed at five coded levels (−1.68, −1, 0, +1 and +1.68), and their actual and coded values are shown in . The design matrix comprising of 20 experiments in duplicates is shown in . A second-order polynomial quadratic was fitted to response as follows:where Y represents the response (AMEP412 yield mg/mL), A, B and C are the independent factors, β1, β2 and β3 are the linear regression coefficients, β11, β22 and β33 are the quadratic coefficients, and β12, β13 and β23 are the cross-product coefficients of the model [Citation14].
Table 2. Levels of the factors tested in the experimental CCD.
Table 3. CCD matrixes for the experimental design and observed response for AMEP412.
Design-Expert software was used to accomplish the CCD and analyse the experimental data. The model was built through a statistical analysis and a regression analysis. The optimisation of AMEP412 production was aimed to establish the maximal levels within the independent factors. The coefficients of the regression equation were also calculated and analysed using the software Design-Expert. Validation of the obtained model was verified experimentally (triplicates) under optimised condition.
Results
The purification and determination of AMEP412
AMEP412 was purified following the method mentioned above. The protein samples before and after purification were collected and determined by SDS-PAGE (). The result showed that most non-target proteins were successfully removed. The concentration of the purified protein sample could represent the yield of AMEP412, which was employed in following optimisation experiments.
Selection of carbon and nitrogen sources
Among the various carbon sources studied, B. subtilis BU412 produced maximum protein in mannitol, followed by maltose and glucose (). Among nitrogen sources, yeast extract favored maximum protein production, followed by peptone and soybean tryptone. It was noticed that inorganic nitrogen source leaded to no production (). Thus, mannitol and yeast extract were selected as the source of carbon and nitrogen, respectively, for further optimisation.
Table 4. Effect of various carbon and nitrogen sources on AMEP412 production.
Selection of significant factors by PBD
In the PBD experiment, twelve runs were carried out to analyse the effects of seven factors on the AMEP412 yield. The experimentally obtained responses as AMEP412 production (mg/mL) were shown in . The production showed a wide variation from 0.203 to 1.064 in the twelve trials, which suggested that the process optimisation was important. The adequacy of the model was calculated, and the variable evidencing statistically significant effects were screened via the analysis of variance and Student’s t test (). Three factors (yeast extract, culture temperature and culture time) were found to have significant influence on AMEP412 production with a confidence level of 95%, while other factors with confidence levels below 95% were considered insignificant and were not included in the following experiments.
Table 5. Analysis of variance (ANOVA) for the PBD results.
Optimisation of AMEP412 production by RSM design
Based on the results of the PBD, three factors (yeast extract, culture temperature and culture time) were considered for CCD analysis by RSM to find out the optimum conditions for maximizing the production of AMEP412 by B. subtilis BU412. The coded and non-coded levels of the three investigated independent factors were listed in . A set of 20 experiments were conducted based on CCD and their observed results were given in . The investigational response of 2.205 mg/mL was found to be the highest, which was obtained for the factors retained at “0” coded level (yeast extract 15 g/L, culture temperature 28 °C and culture time 30 h). Regression analysis indicated that yeast extract (A), culture temperature (B) and culture time extract (C) were all significant for AMEP412 production. The interactions between the factors such as A-B (yeast extract-culture temperature) and A-C (yeast extract-culture time) were significant, while between B and C (culture temperature-culture time) were insignificant (). The model F-value was 46.53, which implied that the model was significant. The determination of coefficient (R2) of the model was 0.9767, with adjusted R2 and predicted R2 as 95.57% and 87.50%, respectively, which indicated a high degree of correlation between the observed and theoretical values. An insignificant lack of fit indicates the validation of quadratic model for the existing study. A second-order response surface model was obtained:where Y is the response (AMEP412 production mg/mL) and A, B and C symbolise the coded values of the independent factors.
Table 6. Analysis of variance (ANOVA) for the CCD experimental results.
The three-dimensional response surface curves were plotted by software Design Expert (Version 8.05b) to illustrate the interaction of the variables and find the optimum conditions for maximum AMEP412 yield. The effect of two independent variables on AMEP412 production was observed by keeping the third variable constant at the center of the graphs. displayed the three-dimensional curves of the estimated responses from the interaction between yeast extract and culture temperature, yeast extract and culture time, and culture temperature and culture time, respectively.
Figure 2. Response surface plot for AMEP412 yield as a function of yeast extract and culture temperature.
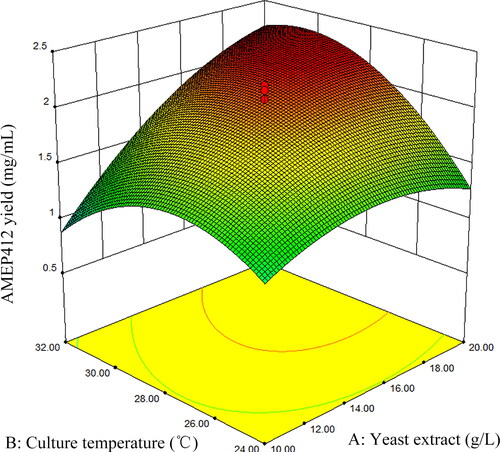
As expressed in , the higher concentration of yeast extract and higher culture temperature showed major impact on enhancing AMEP412 production. The decrease of either factor leaded to the lower production of AMEP412. In , a higher concentration of yeast extract showed a profound increase in AMEP412 yield at moderate temperature. However, increased or declined temperature minimised the production irrespective of the concentration of yeast extract. showed that both moderate culture temperature and moderate culture time were needed to keep higher AMEP412 yield.
Estimated results of the response surface model equation indicated that the highest AMEP412 yield was 2.38 mg/mL with the production parameters as yeast extract 21.72 g/L, culture temperature 30.84 °C and culture time 31.84 h. This value was significantly higher than the non-optimised one (0.32 mg/mL) obtained from the YME medium with former culture conditions, indicating that the RSM design strategy markedly improved AMEP412 production. Confirmation experiments based on optimal factors indicated an AMEP412 yield of 2.39 ± 0.08 mg/mL, which was consistent with the model estimates.
Discussion
In former studies, most protein elicitors were produced by plant pathogen strains [Citation15–19]. However, AMEP412 was secreted by a classical biocontrol strain-B. subtilis. The main difference between these two types of strains lied in the yield of protein elicitor. The expression level of pathogen was generally too low to meet the experiment and application requirements, so most pathogen derived protein elicitors were usually produced by recombinant expression. On the contrary, Bacillus species had good ability to produce various peptides, which were regarded as an important source of antibiotics, enzymes, and insecticides [Citation20,Citation21]. In our previous study [Citation22], the yield of AMEP412 in B. subtilis BU412 could reach 0.3 mg/mL, which was equivalent to the yield of some high efficient recombination expression systems. In addition, AMEP412 was reported as a kind of antimicrobial peptide [Citation22], which was not suitable for recombination expression for its antimicrobial activity against the expression bacteria. Considering the above reasons, the production of AMEP412 was still implemented by its source strain B. subtilis BU412.
Bacillus species are attractive industrial organisms because of their high growth rates leading to short fermentation cycle times, capacity to secrete proteins into the extracellular medium and their general environmental safety [Citation23]. However, the yield of target metabolisms depends on the formulation of fermentation medium and the biosynthesis conditions [Citation24,Citation25]. In this study, the production of AMEP412 was affected by the type of carbon and nitrogen source, which was in accordance with former reports [Citation26,Citation27]. According to the RSM result, the production of AMEP412 was mainly affected by yeast extract, culture temperature and culture time. As the synthesis of AMEP412 by ribosome required sufficient nitrogen source to supply free amino acids, the concentration of yeast extract should be kept at a relatively high level. However, when the concentration of yeast extract exceeded a certain point, it inhibited the yield adversely. This was possibly attributed to the C/N imbalance caused by excess yeast extract [Citation28,Citation29]. A moderate temperature was selected for the optimum production of AMEP412. The low temperature could not satisfy the cell growth and protein synthesis. As AMEP412 showed good thermo stability [Citation4,Citation22,Citation30], the denaturation or degradation caused by high temperature might not be the real reason for limiting the yield. It was inferred the secretion system that AMEP412 occupied tended to close at high temperature. The culture time was also an important factor affecting the production. It was noted that the yield of AMEP412 increased after 24 h, maximised at about 30 h, and then decreased. The tendency had large deviation with the cell growth curve. As AMEP412 was found to have inhibition effect on many microbial [Citation22], including some Bacillus strains (data not shown), we presumed that AMEP412 might play an important role in ecological niche competition. When the nutrition was sufficient, the secretion of AMEP412 was not activated; when the nutrition was in shortage, AMEP412 was secreted to inhibit the nutrition consumption by other microbial cells; when the nutrition was exhausted, the production of AMEP412 was reduced due to limitation of nutrition. In summary, the key point for high production of AMEP412 was keeping the fermentation conditions always at the sweet point, which could be achieved by automatic fermentation in future application.
Conclusions
In this study, PBD and CCD were used to optimise fermentation parameters for enhanced AMEP412 production by B. subtilis BU412. AMEP412 production was significantly dependent on yeast extract, culture temperature and culture time. A7.47-fold improvement in AMEP412 production from the optimised condition was obtained when compared to the yield in the unoptimised one, suggesting the precision and the reliability of that model for protein optimisation studies. This study also supplied optimum fermentation conditions for commercial and cost-effective production of AMEP412.
Author contributions
Quan Liu conceived and designed the experiments. Quan Liu and Yongrui Shen performed the experiments. Quan Liu wrote the manuscript under the guidance of Kuide Yin. All authors reviewed and approved the manuscript.
Funding
This work was financially supported by Heilongjiang Bayi Agricultural University Support Program for San Heng San Zong (ZRCQC201904) and Heilongjiang Bayi Agricultural University Experimental Demonstration Base Project in Jiu San Farm.
Disclosure statement
No potential conflict of interest was reported by the authors.
Data availability statement
All data that support the findings of this study are available from the corresponding authors upon reasonable request.
References
- Jones JD, Dang JL. The plant immune system. Nature. 2006;444(7117):323–329.
- Mishra AK, Sharma K, Misra RS. Elicitor recognition, signal transduction and induced resistance in plants. J Plant Interact. 2012;7(2):95–120.
- Schwessinger B, Ronald PC. Plant innate immunity: perception of conserved microbial signatures. Annu Rev Plant Biol. 2012;63(1):451–482.
- Shen Y, Li J, Xiang J, et al. Isolation and identification of a novel protein elicitor from a Bacillus subtilis strain BU412. AMB Express. 2019;9(1):117.
- Schaad NW, Jones Jb CW. Laboratory guide for the identification of plant pathogenic bacteria. St. Paul (MN): The American Phyto-pathological Society. 2001.
- Akhazarova S, Kavarov V. Experiments optimization in chemistry and chemical engineering. Moscow: Mir Publishers; 1982.
- Plackett RL, Burman JP. The design of optimum multifactorial experiments. Biometrika. 1946;33(4):305–325.
- Montgomery DC. Design and analysis of experiments. 6th ed. New York (NY): Wiley; 2000.
- Khuri AI, Cornell JA. Response surfaces: design and analysis. New York (NY): Marcel Dekker; 1987.
- Deepak V, Kalishwaralal K, Ramkumarpandian S, et al. Optimization of media composition for Nattokinase production by Bacillus subtilis using response surface methodology. Bioresour Technol. 2008;99(17):8170–8174.
- Du R, Zhao F, Qiao X, et al. Optimization and partial characterization of ca-independent α-amylase from Bacillus amyloliquefaciens BH1. Prep Biochem Biotechnol. 2018;48(8):768–774.
- Mizumoto S, Shoda M . Medium optimization of antifungal lipopeptide, iturin A, production by Bacillus subtilis in solid-state fermentation by response surface methodology. Appl Microbiol Biotechnol. 2007;76(1):101–108.
- Wei YH, Wang LC, Chen WC, et al. Production and characterization of Fengycin by indigenous Bacillus subtilis F29-3 originating from a potato farm. Int J Mol Sci. 2010;11(11):4526–4538.
- Bagewadi ZK, Mulla SI, Shouche Y, et al . Xylanase production from Penicillium citrinumisolate HZN13 using response surface methodology and characterization of immobilized xylanase on glutaraldehyde-activated calcium-alginate beads. 3 Biotech. 2016;6(2):164.
- Wei ZM, Laby RJ, Zumof CH, et al. Harpin, elicitor of the hypersensitive response produced by the plant pathogen Erwinia amylovora. Science. 1992;257(5066):85–88.
- Che FS, Nakajima Y, Tanaka N, et al. Flagellin from an incompatible strain of Pseudomonas avenae induces a resistance response in cultured rice cells. J Biol Chem. 2000; 275(41):32347–32356.
- Hanania U, Avni A. High affinity binding site for ethylene-inducing xylanase elicitor on Nicotiana tabacum membranes. Plant J. 1997;12(1):113–120.
- Mao J, Liu Q, Yang X, et al. Purification and expression of a protein elicitor from Alternaria tenuissima and elicitor-mediated defence responses in tobacco. Ann Appl Biol. 2010;156(3):411–420.
- Ma Z, Zhu L, Song T, et al . A paralogous decoy protects Phytophthora sojae apoplastic effector PsXEG1 from a host inhibitor. Science. 2017;355(6326):710–714.
- Dave BR, Parmar P, Sudhir A, et al. Optimization of process parameters for cellulase production by Bacillus licheniformis MTCC 429 using RSM and molecular characterization of cellulase gene. J Bioprocess Biotech. 2015;5(3):1000212.
- Stein T. Bacillus subtilis antibiotics: structures, syntheses and specific functions. Mol Microbiol. 2005;56(4):845–857.
- Liu Q, Shen Y, Yin K. The antimicrobial activity of protein elicitor AMEP412 against Streptomyces scabiei. World J MicrobBiot. 2020;36(1):18.
- Acharya BK, Mohana S, Jog R, et al. Utilization of anaerobically treated distillery spent wash for production of cellulases under solid-state fermentation. J Environ Manage. 2010;91(10):2019–2027.
- Moita C, Feio SS, Nunes L, et al. Optimisation of physical factors on the production of active metabolites by Bacillus subtilis 355 against wood surface contaminant fungi. Int Biodeter Biodeg. 2005;55(4):261–269.
- Grahovac J, Rončević Z, Tadijan IŽ, et al. Optimization of media for antimicrobial compounds production by Bacillus subtilis. Acta Aliment. 2015;44(3):427–435.
- El-Banna NM. Effect of carbon source on the antimicrobial activity of the air flora. World J Microbiol Biotechnol. 2005;21(8–9):1451–1454.
- El-Banna NM, Quddoumi SS. Effect of nitrogen source on the antimicrobial activity of the bacilli air flora. Ann Microbiol. 2007;57(4):669–671.
- Nelly A. Determination of C/N Ratio and development of bioinsecticide production by Bacillus thuringiensis using tofu waste cultivation media. Int J Food Microbiol. 2012;160(2):119–123.
- Samantaray DP, Dash A. Effect of carbon/nitrogen (C/N) ratio on polyhydroxyalkanoates (PHAs) production by Bacillus species under submerged fermentation process. JEnviron Biol. 2020;41(1):118–124.
- Liu Q, Zhang B, Shen Y, et al. Effect of the protein elicitor AMEP412 from Bacillus subtilis artificially fed to adults of the whitefly, Bemisiatabaci (Genn.) (Hemiptera: Aleyrodidae). Egyp J Biol Pest Co. 2020;30:3.