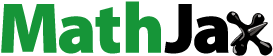
Abstract
Zinc deficiency in human body has gradually become a major challenge to public health in modern society. In our previous work, a novel polysaccharide from Inonotus obliquus IP3a was successfully isolated and purified. The preparation process of IP3a-Zn was optimized by response surface methodology based on single factor experiments. Characterization and antioxidant activities of IP3a-Zn were investigated. The optimal preparation process of IP3a-Zn was obtained as follows: chelation time was 6.3 h, zinc (II) initial concentration was 4.6 mg/mL and mass ratio of IP3a to zinc sulfate heptahydrate was 4.8:1(mg/mg). The weight loss of IP3a-Zn was slightly smaller than that of IP3a within the heating temperature range. After chelation with zinc, IP3a-Zn had crystalline properties like IP3a. IP3a-Zn had stronger antioxidant activity than IP3a. The results indicated that chelation with zinc enhanced the antioxidant activity of IP3a. IP3a-Zn may serve as a good source of zinc as a supplement and could be used as an antioxidant for the food industries.
Introduction
Zinc (Zn) is one of the essential trace elements in the body, and it plays an important role in the normal cell function and metabolism of the human body [Citation1, Citation2]. In recent years, zinc deficiency has become the main cause of public health morbidity, which may result in stunted growth in children [Citation3, Citation4] and a decline in learning and memory [Citation5]. Furthermore, due to zinc deficiency, it can cause oxidative damage to the human body [Citation6]. Currently, zinc supplements can be divided into two forms, including organic zinc (such as polysaccharides or amino acid chelates) and inorganic zinc (such as zinc sulfate or zinc oxide). Among them, inorganic zinc is the most common. However, the human body has a low absorption rate of inorganic zinc, and excessive intake will cause toxicity to the human body. Organic zinc has obvious advantages in human utilization and physiological activity [Citation7]. It is necessary to develop safe, effective, long-term and non-toxic zinc supplements to replace traditional inorganic zinc to increase the zinc content in the human body.
Polysaccharides can be chelated with trace metals or certain non-metals by chemical adsorption [Citation8]. After chelating modification, polysaccharides can improve biological activity and have new functions [Citation9]. At the same time, trace elements are easier to be absorbed by organisms after chelation [Citation10]. There have been reports on the preparation of metal-polysaccharide complexes or chelates, such as selenium-polysaccharide [Citation11, Citation12]. Therefore, complex modification has become an important method for polysaccharide modification.
As a traditional medicinal fungus, Inonotus obliquus has been widely used in China and other countries. It has a long history and has various biological activities such as anti-mitosis and anti-inflammatory activities [Citation13]. It is reported that polysaccharides from I. obliquus have antitumor activity [Citation14, Citation15], antidiabetic activities [Citation16], anti-fatigue [Citation17] and antiapoptotic effect [Citation18]. According to previous research reports, chemical modification of polysaccharides from I. obliquus included sulfation, acetylation and carboxymethylation [Citation19] and a new I. obliquus polysaccharide-iron(III) complex has been successfully synthesized [Citation20]. However, there is no report on the characterization, biological activity and the preparation process of I. obliquus polysaccharide-Zinc (II) complex.
In our previous work, a novel water-soluble polysaccharide IP3a was successfully isolated and purified from I. obliquus [Citation21]. However, the research on chelation between IP3a and Zinc (II) and their biological activities has not been reported yet. Therefore, this study focused on the preparation process of IP3a-Zinc (II) complex (IP3a-Zn) optimized by response surface methodology (RSM) based on single factor experiment, which is an effective statistical method to find optimum processing parameters [Citation22]. In addition, the characterization and antioxidant activity of IP3a-Zn were also investigated. The purpose of this study was to explore whether chelation modification with zinc can improve the antioxidant activity of IP3a and provide basis for development of a new biological zinc supplement with antioxidant activity.
Materials and methods
Materials and chemicals
A novel polysaccharide from I. obliquus coded IP3a was isolated and purified and consisted of rhamnose, arabinose, glucose and galactose in a molar ratio of 2.5:4.6:1.0:2.6 with an average molecular weight of 48,820 Da in our previous work [Citation21]. 1,1-diphenyl-2-picrylhydrazyl (DPPH) was purchased from Sigma Aldrich (Sigma, St Louis, MO, USA). All other chemicals and solvents such as sodium carbonate, absolute ethanol, zinc sulfate heptahydrate, salicylic acid, absolute ethanol, sodium hydroxide, hydrogen peroxide, hydrochloric acid etc. were of analytical reagent grade.
Preparation of IP3a-Zn
Briefly, 25 mL IP3a solution (5 mg/mL) and 25 mL zinc sulfate heptahydrate solution (4 mg/mL) were mixed thoroughly. Under natural pH and 30 °C conditions, the reaction was carried out with shaking at 2.5155 g for 4 h. The reaction solution was then alcohol-precipitated at 4 °C for 24 h and centrifuged (1788.8 g) for 20 min to collect the precipitate. The precipitate was freeze-dried to obtain IP3a-Zn.
Calculation of chelation rate of IP3a-Zn
Zinc sulfate heptahydrate standard solution with different concentrations (0, 0.4, 0.8, 1.2, 1.6, 2.0, 2.4 and 2.8 mg/L) was prepared. The absorbance was measured with an atomic absorption spectrophotometer (Z-2000, Hitachi, Japan). A standard curve was drawn and the equation was obtained as follows: Y = 0.5244X + 0.02451, R2 = 0.9967, where Y is absorbance. X is zinc ion concentration, mg/L.
IP3a and IP3a-Zn was respectively dissolved in 1 L distilled water. According to the data of the zinc standard curve, IP3a-Zn solution was diluted 10 times and then measured with a flame atomic absorption spectrophotometer. According to the absorbance value measured, the zinc ion concentration (mg/L) before and after chelation was obtained, which is recorded as C0 and C, respectively. The chelation rate was calculated according to the following formula:
Single factor experiment of IP3a-Zn preparation
Effect of chelation time on the chelation rate of IP3a-Zn
Initially, 25 mL IP3a solution (5 mg/mL) and 25 mL zinc sulfate heptahydrate solution (4 mg/mL) were mixed thoroughly. Under natural pH and 30 °C conditions, the reaction was carried out with shaking at 150 r/min for different chelation time (2, 4, 6, 8 and 10 h). The reaction solution was then alcohol-precipitated at 4 °C for 24 h and centrifuged (1788.8 g) for 20 min to collect the precipitate. The precipitate was freeze-dried to obtain IP3a-Zn. The effect of chelation time on the chelation rate of IP3a-Zn was investigated to determine the best chelation time.
Effect of zinc (II) initial concentration on the chelation rate of IP3a-Zn
Twenty-five milliliters of IP3a solution (5 mg/mL) and 25 mL zinc sulfate heptahydrate solution with different zinc (II) initial concentration (2, 3, 4, 5, 6 mg/mL) were mixed thoroughly, respectively. Under natural pH and 30 °C conditions, the reaction was carried out with shaking at 2.5155 g for 4 h. The reaction solution was then alcohol-precipitated at 4 °C for 24 h and centrifuged (1788.8 g) for 20 min to collect the precipitate. The precipitate was freeze-dried to obtain IP3a-Zn. Effect of zinc(II) initial concentration on the chelation rate of IP3a-Zn was investigated to determine the best zinc(II) initial concentration.
Effect of mass ratio of IP3a to zinc sulfate heptahydrate on the chelation rate of IP3a-Zn
One milligram of zinc sulfate heptahydrate and IP3a (mass ratio of IP3a to zinc sulfate heptahydrate of 2:1, 3:1, 4:1, 5:1 and 6:1, respectively) were mixed thoroughly with 50 mL distilled water, respectively. Under natural pH and 30 °C conditions, the reaction was carried out with shaking at 2.5155 g for 4 h. The reaction solution was then alcohol-precipitated at 4 °C for 24 h and centrifuged (1788.8 g) for 20 min to collect the precipitate. The precipitate was freeze-dried to obtain IP3a-Zn. Effect of mass ratio of IP3a to zinc sulfate heptahydrate on the chelation rate of IP3a-Zn was investigated to determine the best mass ratio of IP3a to zinc sulfate heptahydrate.
Experiment design of RSM
The ranges of the factors were determined based on preliminary single tests. The chelation rate was used as the response value, a central composite design with three independent variables (X1, Chelation time, X2, Zinc (II) initial concentration, and X3, Mass ratio of IP3a to zinc sulfate heptahydrate) and three levels was chosen to optimize the preparation technology conditions of IP3a-Zn. Factors and levels designed for RSM is shown in .
Table 1. Independent variables and their levels in the response surface design.
Determination of zinc content in IP3a-Zn
IP3a was used a control. The determination of zinc content in IP3a-Zn was performed by flame atomic absorption spectrometric method. Briefly, 0.1 g IP3a-Zn and 0.1 g IP3a were mixed with 10 mL HNO3 and 2 mL HClO4 and hydrolyzed at 160 °C for 12 h, respectively. After the hydrolysis was complete, the sample was diluted with 5 mL water and then analyzed by atomic absorption spectrophotometer (AA6100, Shimadzu Co., Japan).
FT-IR spectroscopy
IP3a (1 mg) and IP3a-Zn (1 mg) powders were dried and mixed with spectroscopic grade potassium bromide respectively and then pressed into pellets. The infrared spectra of IP3a-Zn and IP3a was analyzed with an FT-IR spectrophotometer (Nicolet 6700, Thermo Scientific, USA) at the scanning range of 400-4000 cm−1.
Morphological analysis
The surface structure of IP3a-Zn and IP3a was investigated by a scanning electron microscope (SEM) (Ultra Plus, Zeiss, Germany). The sample was fixed on a clean glass slide with a double-sided carbon conductive adhesive tap. Morphological characterization of the powder was observed by an In-len secondary electron detector (GEMINI).
X-ray diffraction spectrum
The X-ray diffraction spectrum of IP3a-Zn and IP3a was determined by an X-ray diffractometer (SCXmini, Rigaku Inc., Tokyo, Japan). The operating parameters were set as follows: Cu Kα radiation, 40 kV, 200 mA, and a scan rate of 0.5°/min under a nitrogen atmosphere.
Thermogravimetric analysis
Thermogravimetric analysis (TGA) was carried out on a thermo gravimetric analyzer (TGA/SDTA851, Mettler Toledo Instruments Co., Ltd., Switzerland). One milligram of mg sample was inoculated into the sample pan. Thereafter, the sample was heated from 20 to 800 °C at a heating rate of 10 °C/min under a nitrogen atmosphere.
Evaluation of antioxidant activities
Superoxide anion radical scavenging activity
One milliliter of IP3a or IP3a-Zn solution with different concentrations (0.2, 0.4, 0.6, 0.8, 1.0 mg/mL) and 4.5 mL Tris-HCl buffer solution (50 mmol/L, pH 8.2) were mixed with 3.2 mL distilled water for 20 min at 25 °C. Then 0.3 mL pyrogallol was added to react for 3 min at 25 °C. One drop of 10 mol/L HCl was added immediately to stop the reaction. The absorbance was measured at 325 nm. Distilled water was used as the blank control instead of polysaccharide solution. Vitamin C (Vc) was used as the positive control. All assays were performed in triplicate. Superoxide anion radical scavenging rate was calculated as following:
where A is the absorbance value of samples with different concentrations d. A0 is the absorbance value of distilled water.
Hydroxyl radical scavenging activity
Briefly, 1.0 mL IP3a or IP3a-Zn solution with different concentrations (0.2, 0.4, 0.6, 0.8, 1.0 mg/mL) and 1.0 mL salicylic acid ethanol solution (9.0 mmol/L) were mixed with 1.0 mL FeSO4 solution (9.0 mmol/L). Then 1.0 mL H2O2 (9 mmol/L) was added to start the reaction for 30 min at 37 °C. The absorbance was measured at 510 nm. Distilled water was used as the blank control instead of polysaccharide solution. Vc was used as the positive control. All assays were performed in triplicate. The hydroxyl radical scavenging rate was calculated as follows:
where A0 is the absorbance value of distilled water instead of samples with different concentrations; A1 is the absorbance value of distilled water instead of salicylic acid; A2 is the absorbance value of samples with different concentrations.
DPPH radical scavenging activity
Two milliliters of IP3a or IP3a-Zn solution with different concentrations (0.2, 0.4, 0.6, 0.8, 1.0 mg/mL) were mixed with 1 mL DPPH solution (0.1 mmol/L, in 95% ethanol).The mixture was shaken vigorously and allowed to stand for 30 min at 30 °C in dark. The absorbance was measured at 517 nm. Distilled water was used as the blank control instead of polysaccharide solution. Vc was used as the positive control. All assays were performed in triplicate. DPPH radical scavenging rate was calculated as follows:
where A is the absorbance value of samples with different concentrations. A0 is the absorbance value of the DPPH solution without samples.
Statistical analysis
The experimental designs and analysis were done with a Design Expert® software using RSM. The interactions between independent factors and the significant differences were determined with analysis of variance (ANOVA). Differences were considered statistically significant at the p < 0.05 level.
Results
Effect of process parameters on chelation rate
The effect of the chelation time on the chelation rate is shown in . It could be seen that the chelating rate increased continuously with chelating time when the chelating time was less than 6 h. When the chelating time was 6 h, the chelation rate was the highest. When the chelation time increased again, the chelation rate decreased instead. The reason may be that when the chelation time continues to increase, the stability of the reaction system is destroyed. The production rate of side reaction increased to a certain extent, which resulted in complexes dissociation and chelation reduction [Citation23]. Therefore, the best chelation time of IP3a-Zn was 6 h.
Figure 1. Effect of chelation time (A), zinc (II) initial concentration (B) and mass ratio of IP3a to zinc sulfate heptahydrate (C) on chelation rate of IP3a-Zn.
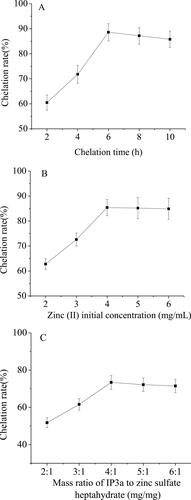
The effect of zinc (II) initial concentration on the chelation rate of IP3a-Zn was shown in . It could be seen that the chelation rate gradually increased with the increase in zinc (II) initial concentration when the zinc (II) initial concentration was lower than 4 mg/mL. When the zinc (II) initial concentration was 4 mg/mL, the chelation rate was the highest. However, when the zinc (II) initial concentration exceeded 4 mg/mL, the chelation rate did not change considerably. The reason may be that the chelation reaction of zinc ions and IP3a had reached a saturated equilibrium state. Therefore, the optimal zinc (II) initial concentration was 4 mg/mL.
The effect of the mass ratio of IP3a to zinc sulfate heptahydrate on the chelation rate of IP3a-Zn was shown in . When the mass ratio of IP3a to zinc sulfate heptahydrate was less than 4:1, the chelation rate gradually increased. When the mass ratio of IP3a to zinc sulfate heptahydrate was 4:1, the chelation rate was the highest. However, when the mass ratio of IP3a to zinc sulfate heptahydrate exceeded 4:1, the chelation rate began to decline slightly. The reason may be that the chelation reaction of zinc ions and IP3a had reached a saturated equilibrium state; too much polysaccharide may disrupt the balance of chelation, which may lead to a decrease in chelation rate.
Statistical analysis and the model building
The result of the response surface analysis of the variation of chelation rate of IP3a-Zn with chelation time (X1), Zinc (II) initial concentration (X2) and mass ratio of IP3a to zinc sulfate heptahydrate (X3) is presented in . The experimental data were analyzed by multiple regression. Chelation rate (Y) was predicted by the following second-order polynomial equation:
Table 2. Result of response surface analysis of the variation of chelation rate of IP3a-Zn with chelation time (X1), Zinc (II) initial concentration (X2) and mass ratio of IP3a to zinc sulfate heptahydrate (X3).
The ANOVA for the response surface quadratic model is summarized in . The ideal regression equation and the high model significance was confirmed by its high F-value (36.64) and low P-value (p < 0.01), which can describe the chelation process of IP3a-Zn.
Table 3. ANOVA for response surface quadratic model.
Linear (X1, X2 and X3) and quadratic coefficients (X12, X22 and X32) were significant for chelation rate (p < 0.05 or p < 0.01). Interaction terms of X1 with X2 and X1 with X3 were significant (p < 0.01). Chelation time (X1) and zinc (II) initial concentration (X2) were the most important factors with a greater effect on chelation rate of IP3a-Zn.
Optimization of preparation process of IP3a-Zn and validation of the model
The 3D response surface is shown in , which reflected the relationship between two independent variables. represents the effect of chelation time, zinc (II) initial concentration and their interactions on the chelation rate of IP3a-Zn. The chelation time and zinc (II) initial concentration had a significant effect on the chelation rate (p < 0.01). At the same time, their interaction was significant (p < 0.01). Compared with the mass ratio of IP3a to zinc sulfate heptahydrate, the chelation time had a more significant effect on the chelation rate of IP3a-Zn (). Their interaction was significant (p < 0.01). The interaction between the zinc (II) initial concentration and the mass ratio of IP3a to zinc sulfate heptahydrate was not significant (p > 0.05) ().
Figure 2. Response surface plots showing the effect of chelation time and zinc (II) initial concentration (A), chelation time and mass ratio of IP3a to zinc sulfate heptahydrate (B) and zinc (II) initial concentration and mass ratio of IP3a to zinc sulfate heptahydrate (C) on chelation rate of IP3a-Zn.
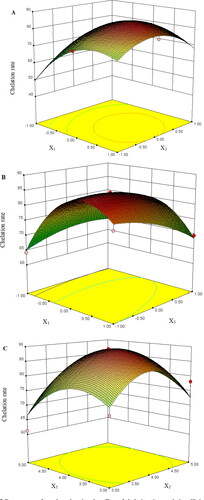
The optimization of the preparation process of IP3a-Zn was based on the analysis of the effect of the chelation conditions on the chelation rate. The optimal preparation process of IP3a-Zn for maximum chelation rate was obtained as follows: the chelation time was 6.3 h, the zinc (II) initial concentration was 4.6 mg/mL and the mass ratio of IP3a to zinc sulfate heptahydrate was 4.8:1 (mg/mg). The maximum chelation rate of IP3a-Zn was 90.2%.
To verify the adequacy of the model equation, three verification experiments were performed under conditions modified for convenience as follows: the chelation time was 6.3 h, the zinc (II) initial concentration was 4.5 mg/mL and the mass ratio of IP3a to zinc sulfate heptahydrate was 5:1 (mg/mg). The average chelation rate of IP3a-Zn was 89.7%, which was in good agreement with the predicted value (90.08%). The result showed that the regression model was accurate and can predict the experimental result in practice.
Zinc content in IP3a-Zn
The zinc content in IP3a-Zn and IP3a is shown in . No zinc was found in IP3a. However, after the combination of IP3a with zinc, the zinc content (7.83 ± 0.52%, w/w) was significantly elevated in IP3a-Zn. The result also suggested that zinc was successfully in conjunction with IP3a.
Table 4. Results of determination of zinc content in IP3a-Zn and IP3a.
FT-IR spectroscopy
The infrared spectrum of IP3a-Zn and IP3a is shown in . It could be seen that a broad peak around 3400 cm−1 was the stretching vibration absorption peak of the O-H group. The weak band around 2910 cm−1 was assigned to the vibration of the C-H group. Compared with the FT-IR spectrum of IP3a, a weak peak of IP3a-Zn appeared at 1640 cm−1 maybe due to the tensile vibration of Zn-O, resulting in the change of hydrogen bonding between OH groups. Crosslinking may be due to electrostatic attraction between the positive charge of zinc ions and the negative charge of polysaccharides [Citation24].
Morphological analysis
The morphological structure of IP3a-Zn and IP3a was analyzed and compared by scanning electron microscope. As shown in , the SEM image of IP3a-Zn and IP3a exhibited obvious difference. The surface of IP3a was relatively smooth and flat with many pore structures (). After chelating with Zn, IP3a-Zn was hairy and the surface was rough (). The reason might be that the electric charge was neutralized after combining with zinc, and the spatial conformation of IP3a tended to assemble.
X-ray diffraction spectrum
The X-ray diffraction spectrum profile of IP3a and IP3a-Zn is shown in . It was observed that there was a ‘bun’ shaped peak in the curve of IP3a and IP3a-Zn, which indicated that IP3a and IP3a-Zn were semi-crystalline substances. After chelation with zinc, the crystalline properties of IP3a were not affected. Zinc treatment did not rearrange the molecular structure of polysaccharide during the chelation process.
Thermogravimetric analysis
The thermogravimetric curve of IP3a and IP3a-Zn is presented in . The weight loss of IP3a and IP3a-Zn involved in three steps. First of all, the weight of IP3a-Zn had a tendency to decline slowly within the range of 200 °C like that of IP3a because of the loss of physically bound water. Secondly, the weight loss trend dropped sharply from 200 to 600 °C because of the loss of chemically bound water caused by oxidation. Finally, when the temperature exceeded 600 °C, relatively little change in weight loss was observed. Compared with IP3a-Zn, the weight loss of IP3a was similar with that of IP3a-Zn. However, the weight loss of IP3a-Zn was slightly smaller than that of IP3a within the heating temperature range. The reason might be that the newly introduced Zn-O group replaced the H-O group in IP3a and changed the structural characteristics, which resulted in the reduction of physical and chemical water.
Antioxidant activities of IP3a and IP3a-Zn
In the present study, the antioxidant activity of IP3a and IP3a-Zn in vitro by scavenging DPPH free radicals, hydroxyl free radicals and superoxide anion free radicals was investigated. The antioxidant activities of IP3a and IP3a-Zn are presented in . As shown in , IP3a and IP3a-Zn had a scavenging effect on superoxide anion free radicals in a concentration-dependent manner. The scavenging capacity of IP3a-Zn on superoxide anion free radicals was higher than that of IP3a. However, the reducing power values of both samples were lower than that of the positive control (Vc). At a concentration of 1.0 mg/mL, the scavenging rate of IP3a, IP3a-Zn and Vc was 48.2%, 53.6% and 68.6%, respectively. As shown in , with the increase in the concentration of IP3a and IP3a-Zn, the scavenging ability of hydroxyl radical increased. The scavenging capacity of IP3a-Zn was stronger than that of IP3a. At a concentration of 1.0 mg/mL, the scavenging rate of IP3a, IP3a-Zn and Vc reached the maximum of 54.2%, 62.6% and 79.2%, respectively. As shown in , IP3a and IP3a-Zn had the obvious scavenging effect on DPPH radical with a concentration-dependent manner. Consistent with the results of scavenging superoxide anion free radicals and hydroxyl free radicals, the scavenging capacity of IP3a-Zn was stronger than that of IP3a. At a concentration of 1.0 mg/mL, the scavenging rate of IP3a, IP3a-Zn and Vc was 55.2%, 60.6% and 85.1%, respectively.
Discussion
In recent years, people have paid more and more attention to zinc-rich polysaccharides with higher antioxidant activity than conventional polysaccharides. As far as we know, there is no report on preparation technology, characterization and biological activity of Inonotus obliquus polysaccharide-Zinc (II) complex. After IP3a was chelated with zinc, we found that IP3a-Zn had an amorphous state. The reason may be that polysaccharides are a heterogeneous class of carbohydrate polymers, composed of monosaccharide units and linked together by glycosidic bonds [Citation23]. As an important indicator of antioxidant activity, ROS is commonly used to assess the health benefits of bioactive polysaccharides. In fact, ROS as free radicals includes hydroxyl radical, superoxide anion radical and non-radical substances [Citation25]. Superoxide anion free radicals can accelerate the aging process from the skin to the internal organs and thereby induce cardiovascular diseases, skin lesions, cancer [Citation26]. We found that the scavenging capacity of IP3a-Zn on superoxide anion free radicals was higher than that of IP3a. The reason may be that the intermediates formed between IP3a-Zn and superoxide anion radicals may be more stable and the ability of scavenging superoxide anion radical increased [Citation26]. The results not only confirmed that the antioxidant activity of IP3a-Zn, but also elucidated that after the chelation of IP3a and zinc, the antioxidant activity of IP3a-Zn did not decrease, but increased further. Hydroxyl radical as one of the most harmful free radicals can cause oxidative damage and cell necrosis or mutation through electron transfer, addition and dehydrogenation [Citation27]. The scavenging capacity of IP3a-Zn was stronger than IP3a. The reason may be related to the coordination of IP3a and Zn, which reduces the number of exposed active groups, thereby weakening the binding with hydroxyl radicals. DPPH is a stable nitrogen-centered organic free radical, widely used in the screening of natural antioxidants [Citation28]. The scavenging capacity of IP3a-Zn on DPPH radical was higher than that of IP3a. The reason may be related to changes in the spatial conformation of the IP3a molecule including the number of zinc binding sites and the binding strength in the complex.
Conclusions
In this work, a novel zinc supplement IP3a-Zn with antioxidant activity was synthesized. The preparation conditions were optimized. The preliminary characteristics of IP3a-Zn were studied. At the same time, the in vitro antioxidant activity of IP3a-Zn was also investigated. After chelation with zinc, IP3a-Zn had crystalline properties like IP3a. IP3a-Zn had stronger antioxidant activity than IP3a, which indicated that the antioxidant activity of IP3a improved in a degree after chelation with zinc. The synthesized IP3a-Zn is an organozinc polysaccharide, which may serve as a good source of zinc as a supplement and could be used as an antioxidant for the food industries. Meanwhile, the present study might provide a new way to develop a new zinc supplement for humans. In the future, the chemical structure and antioxidant mechanism of IP3a-Zn will be investigated.
Data availability statement
All data that support the findings reported in this study are available from the corresponding author upon reasonable request.
Disclosure statement
The authors declare no conflict of interest.
Additional information
Funding
References
- Beyersmann D, Haase H. Functions of zinc in signaling, proliferation and differentiation of mammalian cells. Biometals. 2001;14(3–4):331–341.
- Wang PP, Huang Q, Chen C, et al. The chemical structure and biological activities of a novel polysaccharide obtained from fructus mori and its zinc derivative. J Funct Foods. 2019;54:64–73.
- Caulfield LE, De Onis M, Blössner M, et al. Undernutrition as an underlying cause of child deaths associated with diarrhea, pneumonia, malaria, and measles. Am J Clin Nutr. 2004;80(1):193–198.
- Caulfield LE, Richard SA, Black RE. Undernutrition as an underlying cause of malaria morbidity and mortality in children less than five years old. Am J Trop Med Hyg. 2004;71(2 Suppl):55–63.
- Boroujeni ST, Naghdi N, Shahbazi M, et al. The effect of severe zinc deficiency and zinc supplement on spatial learning and memory. Biol Trace Elem Res. 2009;130(1):48–61.
- Harmaza YM, Slobozhanina EI. Zinc essentiality and toxicity. Biophysical aspects. Biophysics. 2014;59(2):264–275.
- Buff CE, Bollinger DW, Ellersieck MR, et al. Comparison of growth performance and zinc absorption, retention, and excretion in weanling pigs fed diets supplemented with zinc-polysaccharide or zinc oxide. J Anim Sci. 2005;83(10):2380–2386.
- Wang LL, Song S, Zhang B, et al. A sulfated polysaccharide from abalone influences iron uptake by the contrary impacts of its chelating and reducing activities. Int J Biol Macromol. 2019;138:49–56.
- Zhao SW, Li B, Chen GT, et al. Preparation, characterization, and anti-inflammatory effect of the chelate of flammulina velutipes polysaccharide with Zn. Food Agr Immunol. 2017;28(1):162–177.
- Wang H, Betti M. Sulfated glycosaminoglycan-derived oligosaccharides produced from chicken connective tissue promote iron uptake in a human intestinal Caco-2 cell line. Food Chem. 2017;220:460–469.
- Lian KX, Zhu XQ, Chen J, et al. Selenylation modification: enhancement of the antioxidant activity of a glycyrrhiza uralensis polysaccharide. Glycoconj J. 2018;35(2):243–253.
- Li XP, Hou RR, Yue CJ, et al. The selenylation modification of epimedium polysaccharide and isatis root polysaccharide and the immune-enhancing activity comparison of their modifiers. Biol Trace Elem Res. 2016;171(1):224–234.
- Zheng WF, Miao K, Liu Y, et al. Chemical diversity of biologically active metabolites in the sclerotia of Inonotus obliquus and submerged culture strategies for up-regulating their production. Appl Microbiol Biotechnol. 2010;87(4):1237–1254.
- Song Y, Hui J, Kou W, et al. Identification of Inonotus obliquus and analysis of antioxidation and antitumor activities of polysaccharides. Curr Microbiol. 2008;57(5):454–462.
- Jiang SP, Shi FL, Lin H, et al. Inonotus obliquus polysaccharides induces apoptosis of lung cancer cells and alters energy metabolism via the LKB1/AMPK axis. Int J Biol Macromol. 2020;151:1277–1286.
- Wang J, Hu WJ, Li LZ, et al. Antidiabetic activities of polysaccharides separated from Inonotus obliquus via the modulation of oxidative stress in mice with streptozotocin-induced diabetes. PLoS One. 2017;12(6):e0180476–19.
- Zhang CJ, Guo JY, Cheng H, et al. Spatial structure and anti-fatigue of polysaccharide from Inonotus obliquus. Int J Biol Macromol. 2020;151:855–860.
- Han YQ, Nan SJ, Fan F, et al. Inonotus obliquus polysaccharides protect against alzheimer’s disease by regulating Nrf2 signaling and exerting antioxidative and antiapoptotic effects. Int J Biol Macromol. 2019;131:769–778.
- Ma LS, Chen HX, Zhang Y, et al. Chemical modification and antioxidant activities of polysaccharide from mushroom Inonotus obliquus. Carbohydr Polym. 2012;89(2):371–378.
- Wang J, Chen HX, Wang YW, et al. Synthesis and characterization of a new Inonotus obliquus polysaccharide-iron(III) complex. Int J Biol Macromol. 2015;75:210–217.
- Chen YY, Huang YR, Cui ZM, et al. Purification, characterization and biological activity of a novel polysaccharide from Inonotus obliquus. Int J Biol Macromol. 2015;79:587–594.
- Yang T, Zhang SP, Wang RF, et al. Polysaccharides from hizoma panacis majoris and its antioxidant activity. Int J Biol Macromol. 2016;86:56–763.
- Wang L, Li XY. Preparation, physicochemical property and in vitro antioxidant activity of zinc-Hohenbuehelia serotina polysaccharides complex. Int J Biol Macromol. 2019;121:862–869.
- Wang ZJ, Xie JH, Shen MY, et al. Carboxymethylation of polysaccharide from cyclocarya paliurus and their characterization and antioxidant properties evaluation. Carbohydr Polym. 2016;136:988–994.
- Tohma H, Gülçin İ, Bursal E, et al. Antioxidant activity and phenolic compounds of ginger (zingiber officinale rosc.) determined by HPLC-MS/MS. Food Measure. 2017;11(2):556–566.
- Lagercrantz C. Formation of the paramagnetic complex [Cr(OH)5O2]5- in the reaction between chromium(VI) oxide, and hydrogen peroxide or superoxide anion radicals studied by EPR spectroscopy. Free Radic Biol Med. 1999;26(9–10):1134–1137.
- Xu L, Tsona NT, Tang SS, et al. Role of (H2O) n (n = 1–2) in the gas-phase reaction of ethanol with hydroxyl radical: mechanism, kinetics, and products. ACS Omega. 2019; 4(3):5805–5817.
- Hung TM, Na MK, Thuong PT, et al. Antioxidant activity of caffeoyl quinic acid derivatives from the roots of dipsacus asper wall. J Ethnopharmacol. 2006;108(2):188–192.