Abstract
Cadmium (Cd) attracts many researchers because of its potential toxicity to humans. It is available in the soil in high concentrations and can cause physiological, morphological and molecular damage to plants. This study investigated the effect of different concentrations of Cd on the DNA as well as the morphological and physiological traits of pea (Pisum sativum). Inter simple sequence repeat (ISSR) markers were utilized to detect the effect of Cd on the genomic DNA of pea. Two ISSR primers (UBC-26 and UBC-35) resulted in highly clear and reproducible bands. The unique polymorphic bands produced with primer UBC-26 were 250 bp, 450 bp and 600 bp in the control but disappeared under the low, medium and high concentrations of Cd. Moreover, the unique polymorphic bands produced with primer UBC-35 were of sizes of 1500 bp and 2000 bp in the control but disappeared in the low, medium and high concentrations, indicating a genotoxic effect of Cd on pea DNA. The toxicity of Cd also negatively affected the morphological traits of roots, stems, leaves, seeds, flowers and fruit, and their physiological traits. The concentration of some mineral elements, chlorophyll, carotene, sugars, lipids, protein and the activity of some enzymes (glutathione peroxidase, catalase, superoxide dismutase, glutathione reductase, ascorbate peroxidase) decreased compared with the control responding to the Cd toxicity. The study reveals the environmental hazards of Cd on the molecular, morphological and physiological traits of P. sativum.
Introduction
Legumes are a major source of nutritional protein for humans and animals. Due to the importance of legumes to humans and animals, scientists are seeking to improve their growth and production [Citation1]. Pea (Pisum sativum L.) is an important nutrient in the human diet because it contains important nutrients such as starch, protein and mineral elements. The fibers presented in the seeds and the cell wall contribute to the health and vitality of the digestive system. Amylose, which is found in peas, plays an important role in lowering the glycemic index. It also contains a group of phytochemicals that may have antioxidant and anticancer properties [Citation2,Citation3]. Heavy metals are considered one of the most important environmental pollutants as their toxicity causes environmental problems that are reflected in the environment and in living organisms [Citation4]. Heavy metal stress has a significant effect on plant growth characters, physiology, anatomy and genetics [Citation5]. This is also the case in unicellular green algae [Citation6]. Heavy metals penetrate plant systems mostly through the soil [Citation7] or via the atmosphere [Citation8,Citation9]. Some heavy metals are toxic to plants [Citation10]. To reduce the potential harm of these environmental pollutants, plants have cellular mechanisms to regulate the concentration of unnecessary metal ions inside the cell [Citation4]. Cadmium is one of the chemical elements present in the soil in high concentrations and can cause physiological and morphological damage to plants. It is also highly toxic to humans [Citation11]. Because of its high mobility and toxicity at low concentrations in plants, it is also toxic to the environment [Citation12]. It also has the ability to travel through the food chain [Citation13,Citation14]. Perhaps Cd is the element that has attracted the most attention in soil science and plant nutrition because of its potential toxicity to humans, as well as its relative movement in the soil and plant systems [Citation4,Citation15]. The accumulation of Cd is also one of the most harmful effects on plants and animals, and a major cause of environmental pollution [Citation16]. The use of molecular markers such as inter simple sequence repeats (ISSR) is important to determine the polymorphism in the DNA between plants exposed to the toxicity of heavy metals [Citation17]. Cd affected the gene expression levels in the seedlings of Glycine max when the plants were treated with concentrations of 85 and 170 µmol/L [Citation18]. In squash (Cucurbita maxima L), Cd affected some DNA parts and no bands representing them appeared on the agarose gel. The results did not show any relationship between Cd concentration and the number of disappeared bands, which indicated that Cd randomly affected the DNA. Application of Cd at 120 ppm affected 53% of the 13 studied genes [Citation19].
The presence of Cd in the soil contributes to a decrease in plant growth as a result of a decrease in the photosynthesis process of the plant and the transpiration process as a result of stomata closure and low intake of CO2 into plant tissues caused by Cd toxicity [Citation20]. Cadmium can cause morphological changes in the root, causing an imbalance in the ions, as this affects the absorption of some elements, such as magnesium, which affects the transfer of magnesium to the plant [Citation21,Citation22]. Cd toxicity also causes a decrease in root system growth, which may result in an imbalance in enzyme activity and an effect on the cell division process, both of which have a negative impact on root system growth [Citation23]. Changes in growth and anatomy of roots and leaves in soybean seedlings (Glycine max L.) were examined under a concentration of 40 µmol/L CdCl2. A decrease in the growth of the entire plant, the roots and leaves, as well as the content of chlorophyll and carotene, were reported. It also affected the anatomy of the roots and leaves compared to the control [Citation24]. The toxicity of Cd can break the cytoplasmic membranes, resulting in a decrease in the potassium in the plant’s leaves. It also breaks down the lipids and this leads to a disturbance in the concentrations of elements within the plant [Citation21]. The presence of Cd in the plant leads to a decrease in chlorophyll, which is due to changes in chloroplasts by inhibiting the process of manufacturing chlorophyll [Citation20,Citation25]. The toxicity of Cd leads to reduced protein synthesis and may lead to the inability of plant tissues to synthesize proteins [Citation4]. The mechanism of closing the stomata in the leaves is affected by the toxicity of Cd, which leads to a decrease in photosynthesis and thus affects the carbohydrate content in the leaves of plants [Citation20]. Cadmium may accumulate in high concentrations in the vegetative system, which leads to an imbalance in the activity of enzymes and the inhibition of the cellular division process. It also binds to enzymes, which affects the metabolism of carbohydrates, leading to a decrease in the leaf content of sugars [Citation21,Citation23].
The aim of this study was to identify the toxic effects of Cd on DNA using ISSR molecular markers, as well as on morphological and physiological traits in pea plants (Pisum sativum).
Materials and methods
Plant material and growth conditions
To identify the effect of Cd on Pisum sativum, the seeds of the variety ‘Rondo’ were purchased from the local market and used in this experiment. Three seeds were planted in each plastic pot containing 1 kg of sterilized soil. The concentrations of Cadmium (Cd) used for soil treatment were 10 mg/kg soil (low concentration), 50 mg/kg soil (medium concentration), 100 mg/kg soil (high concentration), and 0 mg/kg soil (control). A Completely Randomized Design (CRD) was used with three replicates for each treatment. The Plants were grown in a controlled climate and the light of a greenhouse. Sampling was done after 90 days of sowing and the targeted measurements were taken.
Physiological parameters
Phosphorous content was determined according to Murphy and Riley [Citation26]. Potassium was determined using a Flame photometer Model PFP7 (Jenway, UK) according to Allen et al. [Citation27]. Determination of Ca and Mg were performed using an Atomic Absorption Spectroscopy Model AA-6200 (Shimadzu, Japan) instrument according to Stewart Allen et al. [Citation28]. Chlorophyll A, B, and total chlorophyll were determined according to Metzner et al. [Citation29]. The total sugars content was measured according to Hedge et al. [Citation30]. Reduced sugars were assayed according to Li et al. [Citation31]. The non-reducing sugars content was calculated by subtracting the value of reduced sugars from the value of total sugars. Total protein content was determined based on the nitrogen content of the sample using the Kjeldahl method [Citation32]. Soluble protein was determined according to Bradford [Citation33]. The fat content was determined using the Soxhelt method. Superoxide dismutase (SOD) activity was assayed according to Giannopolitis and Ries [Citation34]. Catalase activity (CAT) was assayed according to Aebi [Citation35]. Guaiacol peroxidase (GPX) enzyme activity was assayed according to Egley et al. [Citation36]. Ascorbate peroxidase (APX) enzyme activity was assayed according to Asada [Citation37].
Genomic DNA isolation
Reagents and chemicals
The stock solution concentrations were as follows: 3 % (w/v) cetyl trimethyl ammonium bromide (CTAB), 1 mol/L TrisCl (pH 8), 0.5 mol/L ethylenediaminetetraacetic acid (EDTA, pH 8), 5 mol/L NaCl, absolute ethanol (AR grade). The extraction buffer contained CTAB 3% (w/v), 100 mmol/L Tris-Cl (pH 8), 25 mmol/L EDTA (pH 8), and 2 mol/L NaCl, in that order. PVP and mercaptoethanol were prepared fresh and added to the extraction buffer.
DNA extraction
A modified CTAB method was used to isolate DNA from plants grown in a greenhouse [Citation38]. The leaf samples were ground into an extraction buffer (100 mmol/L tris buffer pH 8, 25 mmol/L EDTA, 2 mol/L NaCl, 3 % CTAB, 3 % PVP). The suspension was gently mixed and incubated for 20 min at 65 °C with occasional mixing. After cooling to room temperature, an equal volume of chloroform: isoamyl alcohol (24:1) was added. For 5 min, the mixture was centrifuged in a Hettich MIKRO 22 R centrifuge (Kirchlengern, Germany) at 12,000 rpm. The clear upper aqueous phase was transferred to a new tube and 2/3 volume of ice-cold isopropanol was added before incubating at −20 °C for 30 min. Centrifugation at 10,000 rpm for 10 min was used for collecting the nucleic acid. The pellet that resulted was washed twice with 80 percent ethanol. The pellet was air-dried in a sterile laminar hood before being dissolved in TE (10 mmol/L tris buffer pH 8, 1 mmol/L EDTA) at room temperature and stored at 4 °C until use. The RNA was removed by incubating the sample for 30 min at 37 °C with RNase A (10 mg/mL). The absorbance of diluted DNA samples at 260 and 280 nm was measured to determine DNA concentration and purity. The DNA’s quality was assessed using agarose gel (1%) electrophoresis stained with ethidium bromide.
PCR amplification and ISSR reaction
The ISSR primers synthesized by Sigma Company were tested for their ability to amplify genomic DNA extracted from treated and untreated seedlings and the two screened primers were used for later work (). ISSR primers were used for PCR amplification, as per Zeitkiewicz’s protocol [Citation39].
Table 1. List of ISSR primers used in this study.
The PCR reaction was carried out in 20 μL volume of master mixture (Amerson Company, UK). In the master mix, 30 ng of template DNA and 30 ng of primer were added to each tube. Tubes were vortexed and briefly centrifuged after adding template DNA and primer to the master mixture. The amplification was done on 96 well plates on a Primus PCR machine as per the following program. For amplification, denaturation was performed at 94 °C for 3 min, denaturation at 94 °C for 1 min, annealing at 45 °C for 30 s, extension at 72 °C for 1 min, and final extension at 72 °C for 3 min.
Statistical analysis
A Completely Randomized Design was employed [Citation40]. Analysis of variance was performed on the collected data using SAS 9.1, SAS Inc., North Carolina, USA [Citation41] and the least significant difference (LSD) test was used to determine differences among the mean values of the treatments and accessions.
Results
Molecular responses to cadmium toxicity
ISSR primers were tested on the genomic DNA of treated and untreated pea samples. Two of these primers (UBC-26 and UBC-35) produced clear and reproducible bands. These two primers were further used to amplify DNA fragments from all treated and untreated seedlings. The unique polymorphic bands produced with primer UBC-26 were of sizes of 250 bp, 450 bp and 600 bp in the control but disappeared in the samples from the plants grown in the presence of low, medium and high concentrations of Cd ().
Figure 1. ISSR fingerprints obtained with primer UBC-26 from pea plants (Pisum sativum L.) treated with various concentrations of cadmium. Lane M: 1 kb DNA ladder (Sigma-Aldrich); Lane c: control; Lanes 1, 2, 3: plants treated with cadmium at concentrations of 10, 50 and 100 mg/kg soil, respectively. Yellow arrows indicate the polymorphic bands.
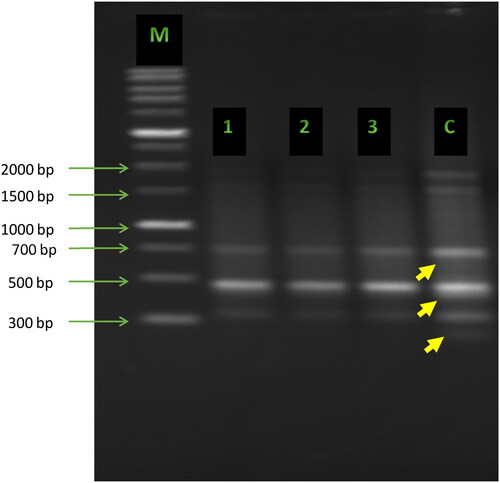
Furthermore, the unique polymorphic bands produced with primer UBC-35 were of sizes of 1500 bp and 2000 bp in the control but disappeared in the low, medium and high concentrations of Cd ().
Figure 2. ISSR fingerprints obtained with primer UBC-35 from pea plants (Pisum sativum L.) treated with various concentrations of cadmium. Lane M: 1 kb DNA ladder (Sigma-Aldrich); Lane c: control; Lanes 1, 2, 3: plants treated with cadmium at concentrations of 10, 50 and 100 mg/kg soil, respectively. Yellow arrows indicate the polymorphic bands.
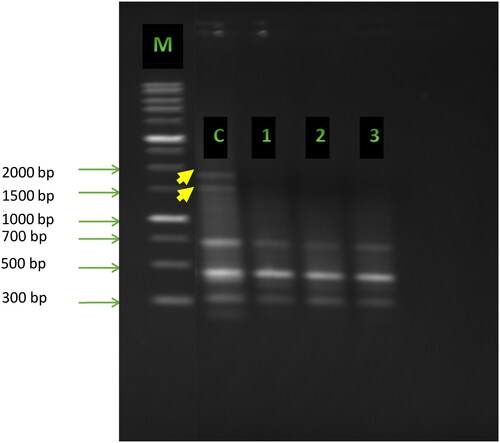
Morphological responses to cadmium toxicity
The results in showed that the control had the best growth, while the other treatments had a negative impact on growth of pea plants due to the Cd effect. Treating the soil with low, medium and high concentrations of Cd resulted in a significant decrease in the mean plant height, leafy area, and number of leaves, seeds, flowers and fruits compared to the control. It was also noticed that the reduction in plant height increased as the Cd concentration increased. The average plant height reached 54.8 cm at the low concentration, while it reached 47.1 cm at the high concentration. The average number of flowers reached 13.8 flowers at the low concentration and 9.1 flowers at the high concentration.
Table 2. Effect of different concentrations of cadmium (Cd) on plant height, leaf area and number of leaves, seeds, flowers and fruits of Pisum sativum.
The results in showed that there was a significant decrease in the average values of both fresh and dry weight of stem, leaf and root due to exposing the pea plants to low, medium and high concentrations of Cd, compared with the control. The average weight of fresh stem, dry stem, fresh leaf, dry leaf, fresh root, and dry root were 67.8 g, 11.2 g, 85.8 g, 14.1 g, 41.8 g, and 13.1 g, respectively, under high concentration of Cd.
Table 3. Effect of different concentrations of cadmium (Cd) on the fresh and dry weight of stem, leaf and root of Pisum sativum.
The results shown in illustrate that different Cd concentrations affected the seed weight. There were significant differences in response to low, medium and high concentrations of Cd. It was observed that the general trend was a decrease in the seed weight whenever the toxicity of the Cd increased compared to the control. The results also indicated that the average weight of seeds in the plants treated with the low concentration (47.8 g) was higher than those exposed to a high concentration (24.2 g). The results also revealed that there was an effect on the fruit’s fresh weight. There were significant differences between low concentrations and both medium and high concentrations. The general trend was for a decrease in the average fruit fresh weight compared to the control as the concentration of Cd increased. It reached 43.2 g and 33.1 g under low and high Cd concentrations, respectively.
Table 4. Effect of different concentrations of cadmium (Cd) on the wet and dry weight of seeds, flowers and fruit in Pisum sativum.
The results shown in indicate that various concentrations of Cd affected the flowering and fruiting dates, as the treating of soil with low, medium and high Cd concentrations led to a delay in the beginning of flowering and fruiting, and a shortening of the flowering and fruiting periods. It also led to a significant decrease in the average number of days between the beginning of flowering, the end of flowering, the duration of flowering, the beginning of fruiting, the end of fruiting and the fruiting period compared to the control. The values of these parameters reached 60.2, 94.2, 34, 65.2, 104.2, 39 days, respectively, when the plants were exposed to the high concentration of Cd.
Table 5. Effect of different concentrations of cadmium (Cd) on flowering and fruiting in Pisum sativum.
The results shown in indicated that there was an effect of the various concentrations of Cd on the mineral elements content of the leaves of pea. The addition of Cd to the soil led to a significant decrease in all mineral elements compared to the control. Because the Cd treatment reduced the K content, there were no significant differences between the low, medium and high concentrations. The decrease in K became more pronounced as the toxicity of the Cd increased. At the low and high concentrations, it reached 86.1 and 83.5 ppm, respectively. The results also indicated that there was an effect of different concentrations of Cd on the Mg content. There were significant differences between the low concentration and the medium and high concentrations. It was noticed that the Mg content in the leaves in the lower concentration was higher than in the medium and high concentrations. The values reached 27.1, 21.4 and 18.1 ppm, respectively.
Table 6. Effect of different concentrations of cadmium (Cd) on the mineral elements in the leaves of Pisum sativum.
shows the effect of various concentrations of Cd on the content of chlorophyll a and b, and carotenoids. There were significant differences between the low, medium and high concentrations for chlorophyll a and b, while there were no significant differences between the concentrations in terms of carotenoids. The reduction in the average leaf content of chlorophyll became more pronounced as the soil Cd concentration increased. It reached 0.63 and 0.39 μg/g at low and high concentrations, respectively. The leaf content of carotenoids was 0.052 and 0.50 μg/g at low and high concentrations, respectively.
Table 7. Effect of different concentrations of cadmium (Cd) on the leaf pigments of Pisum sativum.
The results in indicated that there was an effect of different concentrations of Cd on the average leaf content of reducing sugars. There were significant differences at the low, medium and high concentrations compared with the control, but not between the medium and high concentrations. In terms of the content of non-reducing sugars, there were no significant differences between the medium and high concentrations. There were significant differences between all concentrations in the average leaf content of the total sugars, and it was noticed that the average of all sugars reached the lowest content compared to the control when treated with the high concentration, as it reached 29.8, 176.2 and 206 mg/g, respectively.
Table 8. Effect of different concentrations of cadmium (Cd) on the reducing, non-reducing and total sugars in Pisum sativum.
The results in showed that there was an effect of different concentrations of Cd on the leaf content of lipids and total and soluble protein. The toxicity of Cd at low, medium and high concentrations resulted in significant differences between all the concentrations in the lipid percentage, and total and soluble protein content. The high concentration of Cd was the most toxic compared to the control. The lipid percentage reached 2.74%, the total protein reached 7.1%, and the leaf content of the total protein was 9.6 mg/g.
Table 9. Effect of different concentrations of cadmium (Cd) on the lipids, total protein, and soluble protein in Pisum sativum.
The results in revealed that there was an effect of different Cd concentrations on the antioxidant enzyme activities in the leaves. The treatment of soil with low, medium and high Cd concentrations led to a significant decrease in the average activity of all enzymes. The general trend was a decrement in the enzyme activity as the toxicity of Cd increased compared to the control. It reached 1.49, 51.55, 31.93, 10.4, and 1.4 µmol /min/mg protein of GPX, CAT, SOD, GR, APX, respectively, at high concentration.
Table 10. Effect of different concentrations of cadmium (Cd) on the activity of different enzymes in Pisum sativum.
Discussion
Plants are the subject of a wide range of environmental pollutants varying in their toxicity, concentration, and form, which mainly enter through the soil into the plant system [Citation7]. Heavy metals are the main environmental pollutants. Cadmium is considered not necessary in soil and plant nutrition due to its potential harm and toxicity to living organisms [Citation42]. It is also available in the soil in high concentrations and can cause physiological and morphological damage to plants [Citation11]. In this study, the toxic effect of Cd on the molecular, morphological, and physiological traits of Pisum sativum L. was investigated.
Using genetic engineering techniques to improve metal absorption, metal transfer, Cd accumulation, and overall Cd tolerance in plants opens up new avenues for cadmium phytoremediation [Citation43]. The emerging molecular knowledge contributes to understanding Cd uptake, translocation, and remobilization in plants [Citation44]. The results of [Citation45] offered a genomic sequence resource for functional genetic assignments in Broussonetia papyrifera, which will aid in the understanding of the molecular mechanisms underlying its Cd-stress responses. The phenylpropanoid biosynthesis pathway was reported to be overrepresented, suggesting that this pathway may play a significant role in differential Cd aggregation between two sweet sorghum genotypes [Citation46]. However [Citation47], reported that the pathways enriched under Cd treatment were DNA replication and phenylpropanoid biosynthesis. The ISSR molecular markers revealed a genotoxic effect of low, medium and high Cd concentrations on the DNA of pea. The unique polymorphic bands produced with primer UBC-26 were of sizes of 250 bp, 450 bp and 600 bp in the control disappeared in the low, medium and high concentrations of Cd, indicating genotoxicity. Moreover, the unique polymorphic bands produced with primer UBC-35 were of sizes of 1500 bp and 2000 bp in the control and disappeared in the low, medium and high concentrations of Cd, indicating the genotoxicity of Cd. These results agree with Gzyl et al. [Citation18], who reported a toxic effect of Cd toxicity at concentrations of 85 and 170 μL on the gene expression levels. It also agrees with Al Sahli et al. [Citation19], who studied the toxicity of Cd on the DNA of squash (Cucurbita maxima L.) using ISSR. The results of [Citation19] showed that the toxicity of high concentrations of Cd caused the absence of 53% of the bands, whereas lower concentrations affected 7.7–23.1 percent of the bands. Growth inhibition was observed in terms of average plant height, leafy area, number of leaves, number of seeds, number of flowers, and number of fruits compared to the control. It was also observed that there is a decrease in plant height with the increase in Cd toxicity, and this decrease may be due to a decrease in photosynthesis and transpiration as a result of the closure of the stomata and the low entry of CO2 into the plant tissue caused by Cd toxicity. These findings are consistent with Aravind and Prasad [Citation20]. It may also be attributed to an imbalance in the activity of enzymes and the inhibition of the cell division process, which affects negatively plant growth. This suggestion is in alignment with Cosio et al. [Citation23]. Al Qurainy et al. [Citation48] considered Cd as a heavy metal that causes toxicity to plants. They observed that the treatment of the date palm (Phoenix dactylifera L.) with Cd impedes its growth and development. Root length was more affected due to the accumulation of Cd in the roots. A significant decrease in the weight of the date palm roots and plants was also observed in the treated plants compared to the control [Citation48]. Our study results also agree with Sheirdil et al. [Citation49]. Their results regarding the effect of Cd on soybeans showed a negative effect on growth. It is also in parallel with the study of Sridhar et al. [Citation21], which suggested that the presence of Cd in the soil has a significant effect on the decrease in plant growth. Sridhar et al. [Citation21] also observed that there was a reduction in the wet and dry weight of the stem, leaf, and root compared with the control. The toxicity of Cd increases as the concentration of Cd increases. The reduction in the growth of the root system may be due to an imbalance in the activity of enzymes due to the toxicity of Cd and the effect on the process of cell division. These findings are in parallel with the results of Cosio et al. [Citation23]. Wang et al. [Citation22] studied the root morphological responses of soybean cultivars to Cd toxicity. They recorded a reduction in root length, root surface area, and root size of all varieties, which is in alignment with our results. Our findings are also consistent with Pérez Chaca et al. [Citation24], who conducted a trial on soybean (Glycine max L.) plats treated with CdCl2. They noticed a significant reduction in the growth of roots, leaves and the entire plant compared to the control. They are also consistent with Gzyl et al. [Citation18], who found that root growth was reduced in Glycine max and a decrease in mitotic activity at the same time due to Cd toxicity [Citation18] also observed that the decrease in the weight of seed and fruit was affected by the toxicity of Cd compared to the control. These results are in alignment with Aravind and Prasad [Citation20] and Cosio et al. [Citation23]. The days to flowering and fruiting were also negatively affected by the toxicity of Cd. Cd led to a delay in the days to flowering and fruiting and to shortening the duration of flowering and fruiting, which agrees with Sridhar et al. [Citation21] and Sheirdil et al. [Citation49]. The results of [Citation49] showed that there is an effect of Cd concentrations on the physiological traits of peas. It was observed that the content of Cu, P, K, Fe, Mg, Ca in the leaves decreased as the toxicity of Cd increased, especially at the high concentration compared with the control. The decrease in K content in the leaves may be due to the toxicity of Cd that leads to disruption of the cell membranes. These findings are consistent with Sridhar et al. [Citation21], who suggested that the Cd breaks down the lipids, which leads to a imbalance in the concentrations of elements inside the plant. Sridhar et al. [Citation21] indicated that Cd toxicity has the potential to cause an ionic imbalance through its effect on the absorption of Mg. This decrement in Mg in the plant may be due to Cd leading to morphological changes in the root which affect the transfer of Mg to the plant. Our findings are also in agreement with those of Jibril et al. [Citation50], who investigated the effect of Cd toxicity on Lactuca sativa and indicated that high levels of Cd affect the mineral elements. It has been generally proven that Cd interferes with the absorption, transport and use of many elements, such as P, K and Mg [Citation51]. Cadmium can also alter the absorption of minerals by plants through its effects on the availability of minerals in the soil [Citation52]. In our study, a decrease in leaf content of chlorophyll a and b and carotenoids was observed. This decrease may be due to the toxicity of Cd, which may lead to inhibition of the biological synthesis of chlorophyll. This speculation is in agreement with Aravind and Prasad [Citation20], who suggested that the presence of Cd in the plant leads to a reduction in chlorophyll due to changes in chloroplasts by inhibiting the synthesis of chlorophyll [Citation25]. It also agrees with Pérez Chaca [Citation24], who observed that the toxicity of Cd led to a decrease in the leaf content of chlorophyll and carotenoids in the soybean (Glycine max L.) when treated with a concentration of 40 μm of CdCl2 compared to the control. It is noted that the average of all types of sugars reached their lowest content at the high concentration of Cd compared to the control. The reason behind these reductions in the leaf content of sugars may be that Cd could bind to enzymes, which affects the metabolism of carbohydrates. These results are consistent with those of Cosio et al. [Citation23]. They also agree with the study of Aravind and Prasad [Citation20], who indicated that the toxicity of Cd leads to the closure of the stomata in the leaves, which leads to a decrease in the photosynthesis process and thus affects the leaf content of sugars. The negative effect of different Cd concentrations was observed on the leaf content of lipids, and total and soluble protein. The results of our study are consistent with the study of Fodor et al. [Citation53], who conducted a field trial on sunflower and wheat. Their results showed that Cd toxicity led to a change in the composition of lipids and fatty acids. It also agrees with Benavides et al. [Citation4] who suggested that Cd toxicity leads to a decrease in protein synthesis and may lead to the inability of plant tissues to synthesize proteins. The effect of different Cd concentrations on the leaf content of various enzymes (GPX, CAT, SOD, GR and APX) was noticed. The enzymes are considered one of the main targets of the ions of the heavy metals. The soil’s exposure to heavy metals for a long time leads to a significant decrease in the activity of soil enzymes [Citation54]. Heavy metals also affect the activity of enzymes and lead to their inhibition [Citation51]. The results of our study agree with many previous studies that have suggested that oxidative stress can occur due to Cd toxicity as a result of negatively affecting the activity of enzymes [Citation23,Citation55–61]. In contrast, the results of our study are not consistent with Al Qurainy et al. [Citation48], who studied the effect of CdCl2 on the date palm (Phoenix dactylifera L.) and observed that the activity of CAT and SOD were high at a concentration of 300 mm of CdCl2.
Conclusions
ISSR markers were used in our study to detect the toxic effect of Cd on pea plants. The distinct polymorphic bands produced by primers UBC-26 and UBC-35 vanished in different concentrations of Cd, indicating that Cd has a genotoxic effect on pea DNA. Cd toxicity also had a negative impact on root, stem, leaf, seed, flower and fruit morphology, as well as on a range of physiological indicators. In plants exposed to Cd toxicity, the concentrations of some mineral elements, chlorophyll, carotene, sugars, lipids, protein and the activity of some enzymes (GPX, CAT, SOD, GR, APX) decreased compared to the control. The present study demonstrates the environmental risks of Cd on the molecular, morphological and physiological characteristics of Pisum sativum.
Disclosure statement
The author declares that there is no conflict of interest regarding the publication of this paper.
Data availability statement
All data that support the findings reported in this study are available from the corresponding author upon reasonable request.
Funding
The author(s) reported there is no funding associated with the work featured in this article.
References
- Bourgeois M, Jacquin F, Cassecuelle F, et al. A PQL (protein quantity loci) analysis of mature pea seed proteins identifies loci determining seed protein composition. Proteomics. 2011;11(9):1581–1594.
- Dahl WJ, Foster LM, Tyler RT. Review of the health benefits of peas (Pisum sativum L.). Br J Nutr. 2012;108(S1):S3–S10.
- Mitchell DC, Lawrence FR, Hartman TJ, et al. Consumption of dry beans, peas, and lentils could improve diet quality in the US population. J Am Diet Assoc. 2009;109(5):909–913.
- Benavides MP, Gallego SM, Tomaro ML. Cadmium toxicity in plants. Braz J Plant Physiol. 2005;17(1):21–34.
- Özyiğit İ̇L, Hocaoğlu-Özyiğit A, Kurmanbekova G, et al. Cadmium stress in barley seedlings: accumulation, growth, anatomy and physiology. Int J Life Sci Biotechnol. 2021;4(2):204–223.
- Vingiani GM, Gasulla F, Barón-Sola Á, et al. Physiological and molecular alterations of phycobionts of genus trebouxia and coccomyxa exposed to cadmium. Microb Ecol. 2021;82(2):334–343. 2021
- Arshad M, Silvestre J, Pinelli E, et al. A field study of lead phytoextraction by various scented pelargonium cultivars. Chemosphere. 2008;71(11):2187–2192.
- Pourrut B, Shahid M, Dumat C, et al. Lead uptake, toxicity, and detoxification in plants. Rev Environ Contam Toxicol. 2011;213:113–136.
- Uzu G, Sobanska S, Sarret G, et al. Foliar lead uptake by lettuce exposed to atmospheric fallouts. Environ Sci Technol. 2010;44(3):1036–1042.
- Page V, Feller U. Heavy metals in crop plants: transport and redistribution processes on the whole plant level. Agronomy. 2015;5(3):447–463.
- Jegadeesan S, Yu RY, Yu K. Genetic control of cadmium concentration in soybean seeds. In: Goyal A, editor. Grain legumes. Rijeka (Croatia): InTech Open; 2016. p. 79–103.
- Ye H, Yang X, He B, et al. Growth response and metal accumulation of sedum alfredii to cd/Zn complex – polluted ion levels. Acta Bot Sin. 2003;45(9):1030–1036.
- Hattori H, Asari E, Chino M. Estimate of cadmium concentration in brown rice. In: World congress of soil science, Bangkok (Thailand), 14–21. Aug 2002.
- Hocaoglu-Ozyigit A, Genc NB. Cadmium in plants, humans and the environment. Front Life Sci. 2020;1(1):12–21.
- Tran TA, Popova LP. Functions and toxicity of cadmium in plants: recent advances and future prospects. Turk J Bot. 2013;37(1):1–13.
- Dong J, Mao W, Zhang G, et al. Root excretion and plant tolerance to cadmium toxicity-a review. Plant Soil Environ. 2008;53(5):193–200.
- Al-Qurainy F. Application of inter simple sequence repeat (ISSR marker) to detect genotoxic effect of heavy metals on eruca sativa (L.). African Journal of Biotechnology. 2010;9(4):467–474.
- Gzyl J, Chmielowska-Bąk J, Przymusiński R, et al. Cadmium affects microtubule organization and post-translational modifications of tubulin in seedlings of soybean (Glycine max L.). Front Plant Sci. 2015;6:937.
- Al Sahli A, Al-Sokari S, El-Zaidy M. Effect of cadmium on DNA of squash plant using ISSR technique. Saudi Journal of Biological Sciences. 2013;20(5):67–76.
- Aravind P, Prasad MNV. Cadmium-zinc interactions in a hydroponic system using ceratophyllum demersum L.: adaptive ecophysiology, biochemistry and molecular toxicology. Braz J Plant Physiol. 2005;17(1):3–20.
- Sridhar BBM, Han FX, Diehl SV, et al. Effects of Zn and cd accumulation on structural and physiological characteristics of barley plants. Braz J Plant Physiol. 2007;19(1):15–22.
- Wang P, Deng X, Huang Y, et al. Root morphological responses of five soybean [Glycine max (L.) merr] cultivars to cadmium stress at young seedlings. Environ Sci Pollut Res. 2016;23(2):1860–1872.
- Cosio C, Martinoia E, Keller C. Hyperaccumulation of cadmium and zinc in thlaspi caerulescens and arabidopsis halleri at the leaf cellular level. Plant Physiology. 2004;134(2):716–725.
- Chaca MP, Vigliocco A, Reinoso H, et al. Effects of cadmium stress on growth, anatomy and hormone contents in Glycine max (L.) merr. Acta Physiol Plant. 2014;36(10):2815–2826.
- Zhong-Qiu Z, Yong-Guan Z, Yun-Long C. Effects of zinc on cadmium uptake by spring wheat (Triticum aestivum, L.): long-time hydroponic study and short-time 109 cd tracing study. J Zheijang Univ Sci A. 2005;6(7):643–648.
- Murphy J, Riley JP. A modified single solution method for the determination of phosphate in natural waters. Anal Chim Acta. 1962;27:31–36.
- Allen S, Grimshaw H, Parkinson J, et al. Organic constituents. In Allen SE, editor. Chemical analysis of ecological materials. Oxford: Blackwell Scientific Publications; 1989. p. 160–200.
- Allen SE, Grimshaw HM, Parkinson JA, et al. 1974. Chemical analysis of ecological materials. Oxford: Blackwell Scientific Publications.
- Metzner H, Rau H, Senger H. Untersuchungen zur synchronisierbarkeit einzelner pigmentmangel-mutanten von chlorella. Planta. 1965;65(2):186–194.
- Hedge J, Hofreiter B, Whistler R. Carbohydrate chemistry. New York: Academic Press; 1962. p. 17.
- Li H, Long C, Zhou J, et al. Rapid analysis of Mono-saccharides and oligo-saccharides in hydrolysates of lignocellulosic biomass by HPLC. Biotechnol Lett. 2013;35(9):1405–1409.
- Jones Jr JB, Wolf B, Mills HA. Plant analysis handbook. In: A practical sampling, preparation, analysis, and interpretation guide. Athens, Georgia: Micro-Macro Publishing Inc; 1991. p. 127–189.
- Bradford M. A rapid and sensitive method for the quantification of microgram quantities of protein utilizing the principle of protein-dye binding. Anal Biochem. 1976;72(1–2):248–254.
- Giannopolitis CN, Ries SK. Superoxide dismutases: I. Occurrence in higher plants. Plant Physiol. 1977;59(2):309–314.
- Aebi H. Catalase in vitro. In: Packer L, editor. Methods in enzymology. Vol. 105. New York: Academic Press; 1984. p. 121–126.
- Egley G, Paul R, Vaughn K, et al. Role of peroxidase in the development of water-impermeable seed coats in sida spinosa L. Planta. 1983;157(3):224–232.
- Asada K. Chloroplasts: formation of active oxygen and its scavenging. In: Packer L, editor. Methods in enzymology. Vol. 105. New York: Academic Press; 1984. p. 422–429.
- Khan S, Qureshi MI, Alam T, et al. Protocol for isolation of genomic DNA from dry and fresh roots of medicinal plants suitable for RAPD and restriction digestion. Afr J Biotechnol. 2007;6(3):175.
- Zietkiewicz E, Rafalski A, Labuda D. Genome fingerprinting by simple sequence repeat (SSR)-anchored polymerase chain reaction amplification. Genomics. 1994;20(2):176–183.
- Stell R, Torrie J, Dickey D. 1980. Principles and procedures of statistics: a biometrical approach. New York:MacGraw-Hill.
- SAS Institute. SAS 9.4 output delivery system: User’s guide. Cary (NC): SAS Institute; 2014.
- Jali P, Pradhan C, Das AB. Effects of cadmium toxicity in plants: a review article. Sch Acad J Biosci. 2016;4(12):1074–1081.
- Raza A, Habib M, Kakavand SN, et al. Phytoremediation of cadmium: physiological, biochemical, and molecular mechanisms. Biology (Basel). 2020;9(7):177.
- Shiyu QIN, Hongen LIU, Zhaojun NIE, et al. Toxicity of cadmium and its competition with mineral nutrients for uptake by plants: a review. Pedosphere. 2020;30(2):168–180.
- Xu Z, Dong M, Peng X, et al. New insight into the molecular basis of cadmium stress responses of wild paper mulberry plant by transcriptome analysis. Ecotoxicol Environ Saf. 2019;171:301–312.
- Feng J, Jia W, Lv S, et al. Comparative transcriptome combined with morpho-physiological analyses revealed key factors for differential cadmium accumulation in two contrasting sweet sorghum genotypes. Plant Biotechnol J. 2018;16(2):558–571.
- Qiao K, Liang S, Wang F, et al. Effects of cadmium toxicity on diploid wheat (triticum urartu) and the molecular mechanism of the cadmium response. J Hazard Mater. 2019;374:1–10.
- Al-Qurainy F, Khan S, Tarroum M, et al. Biochemical and genetical responses of phoenix dactylifera L. to cadmium stress. Biomed Res Int. 2017;2017:9504057.
- Sheirdil RA, Bashir K, Hayat R, et al. Effect of cadmium on soybean (Glycine max L.) growth and nitrogen fixation. Afr J Biotechnol. 2012;11(8):1886–1891.
- Jibril SA, Hassan SA, Ishak CF, et al. Cadmium toxicity affects phytochemicals and nutrient elements composition of lettuce (Lactuca sativa L.). Adv Agric. 2017;2017:1–7.
- Das P, Samantaray S, Rout G. Studies on cadmium toxicity in plants: a review. Environ Pollut. 1997;98(1):29–36.
- Moreno J, Hernández T, Garcia C. Effects of a cadmium-contaminated sewage sludge compost on dynamics of organic matter and microbial activity in an arid soil. Biol Fertil Soils. 1999;28(3):230–237.
- Fodor E, Szabó-Nagy A, Erdei L. The effects of cadmium on the fluidity and H+-ATPase activity of plasma membrane from sunflower and wheat roots. J Plant Physiol. 1995;147(1):87–92.
- Tyler G, Påhlsson A-MB, Bengtsson G, et al. Heavy-metal ecology of terrestrial plants, microorganisms and invertebrates. Water Air Soil Pollut. 1989;47(3-4):189–215.
- Cho U-H, Seo N-H. Oxidative stress in Arabidopsis thaliana exposed to cadmium is due to hydrogen peroxide accumulation. Plant Sci. 2005;168(1):113–120.
- Fornazier RF, Ferreira RR, Vitoria A, et al. Effects of cadmium on antioxidant enzyme activities in sugar cane. Biologia Plant. 2002;45(1):91–97.
- Gallego SM, Benavides MP, Tomaro ML. Effect of heavy metal ion excess on sunflower leaves: evidence for involvement of oxidative stress. Plant Sci. 1996;121(2):151–159.
- Sandalio L, Dalurzo H, Gomez M, et al. Cadmium-induced changes in the growth and oxidative metabolism of pea plants. J Exp Bot. 2001;52(364):2115–2126.
- Shaw B. Effects of mercury and cadmium on the activities of antioxidative enzymes in the seedlings of phaseolus aureus. Biologia Plant. 1995;37(4):587.
- Somashekaraiah B, Padmaja K, Prasad A. Phytotoxicity of cadmium ions on germinating seedlings of mung bean (Phaseolus vulgaris): involvement of lipid peroxides in chlorphyll degradation. Physiol Plant. 1992;85(1):85–89.
- Stohs SJ, Bagchi D. Oxidative mechanisms in the toxicity of metal ions. Free Radic Biol Med. 1995;18(2):321–336.