Abstract
As a medicinal plant, Sophora tonkinensis is mainly distributed in Guangxi, Yunnan, Guizhou and northern Vietnam. In recent years, due to the strong demand for S. tonkinensis, wild resources have become decreased, which has placed great pressure on their protection. Therefore, the introduction of S. tonkinensis was carried out in Guangxi. Although the flowering period of S. tonkinensis is long, its seed setting rate is low. To promote flower production, it is necessary to do basic research on flowering in S. tonkinensis. In this study, the contents of various mineral elements in the roots, stems and leaves of S. tonkinensis were measured during the period of flowering, and cluster analysis was carried out on the content changes. At the same time, the correlations among the elements were analyzed. It was found that As and Hg inhibited the absorption of some important elements related to flower development. It is necessary to pay attention to the effects of these toxic elements on flowering during cultivation. RNA-seq of flower buds and flower organs was carried out, and the weighted gene co-expression network analysis (WGCNA) method was used to mine the key genes. These genes may play an important role in flowering control and flower development in S. tonkinensis.
Supplemental data for this article is available online at https://doi.org/10.1080/13102818.2021.1988707 .
Introduction
Sophora tonkinensis is a leguminous plant that is mainly distributed in Guangxi, Guizhou and Yunnan provinces of China and northern Vietnam [Citation1]. According to the Chinese pharmacopeia, its dry roots and stems are used as a traditional medicine in the treatment of a sore throat. Many studies have found that it has anti-tumor, anti-virus and immune-enhancing effects [Citation2–5]. In recent years, there has been growing demand for S. tonkinensis, resulting in a decrease in its wild resources [Citation1]. The artificial cultivation of S. tonkinensis is an essential measure for sustainable utilization. The introduction and domestication of S. tonkinensis have been carried out all over Guangxi in recent years. In this process, we found that there are some problems such as poor quality of the flowers and no flowering of old branches, which restrict the seed production of S. tonkinensis seriously. However, studies on the flowering mechanism of S. tonkinensis were still missing.
Many studies have shown that the development of flowers and fruiting are closely related to mineral elements. The low potassium (K) concentrations in leaves were correlated with a large number of sterile female flowers in Solanum sisymbriifolium [Citation6]. Copper (Cu) is an essential element for pollen development [Citation7]. Zinc (Zn) and manganese (Mn) also play important roles in maize pollen development [Citation8,Citation9]. Iron (Fe) contributes to inflorescence formation and pollen production [Citation10,Citation11]. Molybdenum (Mo) affects pollen production and viability [Citation12]. Boron (B) is an essential element for pollen tube growth [Citation13]. Some mineral elements such as arsenic (As), cadmium (Cd), chromium (Cr), lead (Pb) and mercury (Hg) disturb the growth and development of plants, as well as the normal physiological metabolism of plants [Citation14]. These elements are also harmful to the human body, so it is necessary to control their contents in the roots and stems of S. tonkinensis. Thus, it is important to study the dynamic changes of mineral element contents in plants to understand the demand dynamics, absorption capacity and nutrient regulation of plants, which can provide theoretical guidance for the regulation of flowering and fruiting and improve the quality of medicinal materials. Therefore, we determined the contents of 25 mineral elements in the roots, stem and leaves of S. tonkinensis during florescence. Self-organizing map (SOM) cluster analysis and correlation analysis were used to obtain the correlations between each element content in our study.
Weighted gene co-expression network analysis (WGCNA) is a commonly used biological approach to identify the patterns of correlations among genes [Citation15]. In recent years, many studies involving WGCNA analysis have been reported in plant science, such as studies of desiccation tolerance in Boea hygrometrica [Citation16], carotenoid accumulation in Chrysanthemum × morifolium [Citation17], secondary biosynthetic pathways in Camellia sinensis [Citation18] and others. To further understand the molecular mechanism regulating the flowering period of S. tonkinensis, transcriptome data of flower buds at different developmental stages were obtained. These data have been analyzed, and some genes have been mined based on the known related genes in other plants [Citation19]; however, considering the unique genetic background of S. tonkinensis, this study uses the WGCNA [Citation20] method to re-analyze the data and find the specific genes involved in the flowering of S. tonkinensis.
Materials and methods
Plant materials
The materials from S. tonkinensis used for determining the contents of mineral elements were collected from the culture populations in the Guangxi Botanical Garden of Medicinal Plants (22°51’26.6′’N, 108°23’11.5” E). During the period from the flower bud appearing in April to the last flower blooming in June, roots, stems and leaves of S. tonkinensis for determining the mineral elements content were collected at 10 time points (April 8th, April 17th, April 23rd, April 30th, May 7th, May 14th, May 21st, May 28th, June 4th and June 12th) in 2018. The materials for transcriptome analysis were collected from 8 different developmental stages of flowers and flower buds from the same culture populations in 2019. The morphology of each stage is shown in .
Figure 1. Morphologies of 8 different developmental stages. A: Flower bud formation; B: The initial inflorescence primordia formation; C: New inflorescence primordia formed continuously; D: The primordium of initial inflorescence began to differentiate, the calyx formed, and the primordium began to expand and separate from the calyx; E: The floral primordia expanded further and separated from the calyx, and the primordium had a tendency toward primary differentiation; F: Three primordia: petal, stamen and carpel; G: The petals began to stratify, the anthers began to expand, and the pistils formed ovules and the polar nucleus; H: The flower morphology was completed, the number of petals was determined, the anther development was completed, the pollen was wrapped, and the pistil structure was established.
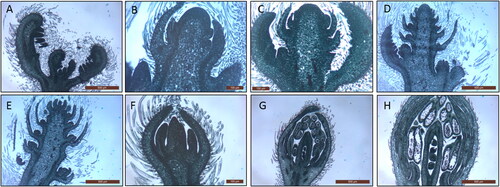
Determination of mineral element content
In total, 25 mineral elements were detected using ICP-MS from plant materials. The 25 mineral elements were K, calcium (Ca), sodium (Na), magnesium (Mg), Zn, Cu, Fe, Mo, Mn, B, cobalt (Co), Cd, tin (Sn), nickel (Ni), antimony (Sb), aluminium (Al), thallium (Tl), Pb, titanium (Ti), vanadium (V), strontium (Sr), Hg, As, selenium (Se) and Cr.
After drying and grinding, the materials were weighed to 0.23-0.26 g for acid digestion (6 mL of HNO3 and 1 mL of H2O2 were added at 90 °C for 30 min). Then, the products of predigestion were digested in a microwave instrument (MILSTONE ETHOS ONE). The specific digestion procedure is shown in . The mineral elements were analyzed using an Agilent (Santa Clara, CA, USA) 7700 ICP-MS following a serial dilution of the standard solution. The specific working parameters of ICP-MS and the linearity, content and detection limit of each element are shown in the supplementary materials (Supplemental Tables S1 and S2).
Table 1. The microwave digestion procedure.
Analysis of mineral element content data
According to the content changes of each element at different time points, cluster analysis was carried out. In this paper, self-organizing competitive neural network (SOM) clustering analysis [Citation21] with 16 nodes was used instead of traditional K-means clustering. The main purpose was to avoid the influence of the K value on the result. The software used for clustering analysis was the Kohonen package in R software [Citation22]. The correlations of each element change were analyzed by R software, and the results were visualized by the package corrplot in R software [Citation23].
RNA extraction and construction of the cDNA library
The total RNA was extracted from flower buds using a Hipure HP Plant RNA Mini Kit (Magen, Guangzhou, China), with three biological replicates at each stage. The quality of the RNA samples was assessed using an RNA 6000 Nano LabChip Kit and a Bioanalyzer 2100 (Agilent Technologies, Santa Clara, CA, USA). The mRNA was purified using a NEBNext® Poly (A) mRNA Magnetic Isolation Module (New England Biolabs, Ipswich, MA, USA). A fragmentation buffer was added to disrupt the mRNA strands into short fragments, which were used as templates to synthesize cDNA using reverse transcriptase and random hexamer primers. The double-stranded cDNA fragments were subjected to end repair and adapter ligation. The cDNA library was achieved using the NEBNext® Ultra™ RNA Library Prep Kit for Illumina® (New England Biolabs, Ipswich, MA, USA).
Illumina sequencing, assembly, annotation, and gene expression analysis
The cDNA library was sequenced on an Illumina HiSeq 2500 sequencing platform (Illumina, San Diego, CA, USA) to yield 2 × 150-bp paired-end raw reads. The adapter sequences and low-quality sequences were removed from the raw reads using Trimmomatic [Citation24]. The sequencing reads were de novo assembled using pooled reads from all replications and stages by Trinity software [Citation25], and the results were further clustered and assembled using TGICL [Citation26]. The assembled transcriptome sequences were searched against the Swiss-Prot, KEGG and TrEMBL databases using Blastp with a cut-off E-value of 10 − 5. GO annotation was conducted using InterProScan [Citation27]. RSEM [Citation28] was executed to estimate the fragments per kilobase of exon per million mapped fragments (FPKM) value of each unigene.
Weighted gene co-expression network analysis (WGCNA)
WGCNA analysis was performed by the WGCNA package in R software. In the data from RNA sequencing, there were more than 100,000 genes; however, to improve the computational efficiency, WGCNA analysis only examined the genes with the top 20,000 expression levels. These genes were further divided into 67 modules using WGCNA, and the correlations between modules and morphologies of flowers in different developmental stages were determined. The genes in one module with the top 100 relationship weights were used to construct a coexpression network in Cytoscape software version 3.8.0. In the network, genes that are connected to a greater number of other genes are considered to play important roles in the development of flowers.
Results
Changes in element contents
To study whether there are certain relationships between the content changes of each element at different time points, the changes were classified by SOM clustering analysis. The results showed that the changes of elements in the roots and stems were divided into 14 groups; however, those of the leaves were divided into only 13 groups (). The changes of Pb, Ti, V, Fe and Co in the roots were clustered into one group (in one neuron). There was a peak of Al and Tl in early and middle florescence in roots. Al, V and Fe in the stems were clustered into one group, and their changes were similar to those of Sr, Ti and Cr, showing a peak in late florescence. Co, Fe, Cr and V in the leaves were clustered into one group with multiple peaks, which was very similar to the trend of Al, Cu and Ni during flowering.
Based on the changes of element contents, correlation analysis of each element was carried out (). The results showed that there are significant positive correlations among Co, Tl, Al, Pb, V, Ti and Fe in roots, excluding Al and Co, which indicated that these elements promoted each other in terms of absorption and accumulation in the roots. Additionally, there were significant positive correlations among Cu, B and Sr. There was also a significant correlation between Mo and Sr. As and Mo showed a significant negative correlation, which suggests that As restricted the absorption and accumulation of Mo in the roots.
The correlation study in the stems showed that there were significant positive correlations among Ti, Cr, Sr, Fe, V and Al. These elements may share mutual dynamic changes. At the same time, there were also some positive correlations among other elements (Cu and Zn | Pb and Co | Co and Cd | Co and Mn | V and Mn | Al and Mn | B and Ca), whereas As and Mo had an obvious negative correlation, which suggests that As could limit the accumulation of Mo in stems too. There was a significant correlation among Cr, Fe, Co, Ni and V in leaves. At the same time, positive correlations also existed for other elements (Ti and Cr | Tl and Na | Cu and B | Cu and Sr | Cu and Zn | Sr and Zn | Cd and Mo | B and Mo | Cu and Zn | Se and K). There were two significant negative correlations between Cu, B and As and between Sr, Zn and Hg, which indicated that As and Hg had a limiting effect on the essential trace elements B, Cu, Sr [Citation29] and Zn absorbed by leaves.
Important genes controlling flower development
According to WGCNA analysis, these genes were divided into 67 modules according to their coexpression patterns (). Here, the data from RNA-seq were collected from 8 developmental stages of flowers and were used for correlation analysis between genes and phenotypes. According to the results, we further analyzed the most positively related modules, which were named lightcyan, skyblue, midlightblue and magenta (). The gene coexpression networks of these four modules were analyzed, respectively (), and some important control genes were screened. These genes were c66984_g1, c130725_g1, c119764_g2, c99932_g1, c6168_g1, c63291_g1, c110050_g1, c108307_g1, c115342_g1, c115062_g1, c123287_g1 and c127266_g4. Among them, c66984_g1, c130725_g1, c119764_g2 and c99932_g1 are important genes in flower bud development (); c6168_g1, c63291_g1 and c110050_g1 are important genes in inflorescence primordium (); c108307_g1, c115062_g1 and c115342_g1 are important genes in flower primordium development (), and c123287_g1 and c127266_g4 are important genes in flower development ().
S. tonkinensis is an endemic species distributed in the tropical karst mountains of China and Vietnam, and its research foundation is weak. To further understand the flowering molecular mechanism of S. tonkinensis, we obtained the gene sequences of flower buds and flowers by the RNA-seq method. Through WGCNA analysis, we found some genes that may play a key role in controlling flower development. Among them, there are four important genes in flower bud developent (c66984_ g1, c130725_ g1, c119764_ g2 and c99932_ g1). c66984_g1 is likely to encode the translation initiation factor. The c130725_ g1 gene is similar to the LSH10 gene of Lupinus micranthus and its product belongs to the ALOG protein family, which plays an important role in the development of plant floral organs [Citation30,Citation31]. c119764_ g2 is similar to PILS3 (PIN-LIKES 3), which may be involved in the auxin signalling pathway [Citation32]. c99932_g1 may be involved in the ubiquitination degradation of proteins from the sequence domain.
Three genes are related to inflorescence primordia (c6168_ g1, c63291_ g1 and c110050_ g1), among which c6168_ g1 may encode a peptidyl-prolyl cis-trans isomerase (PPIase) in plants and play an important helping role in protein folding [Citation33]. Interestingly, a study has shown that PPIase is involved in the regulation of the flowering time in Arabidopsis thaliana [Citation34]. c63291_ g1 may encode a 28 s ribosomal protein. c110050_ g1 may be an SRSF protein kinase, which is involved in the regulation of mRNA splicing [Citation35–37].
In the formation of floral primordia, c108307_ g1 may be an IPTK family gene involved in the phosphatidylinositol signalling pathway [Citation38], and c115342_ g1 may be a member of the FRIGIDA-like gene family, which is considered to be related to flowering time control in Arabidopsis thaliana [Citation39]. Therefore, c115342_g1 is considered to be related to flowering time control. c115062_ g1 may also be a hub gene in WGCNA; however, its functional annotation is absent.
In flower development, we screened the c123287_ g1 and c127266_ g4 genes. c123287_ g1 may be a galacturonosyl transferase (GAUT) gene, which plays an important role in pectin synthesis [Citation40]. It has been reported that GAUT may be related to the growth of pollen tubes [Citation41], and another gene, c127266_ g4, may be a DAYSLEEPER-like gene, which is a transposase-derived gene and may influence the transcription of other genes [Citation42].
Discussion
Some of the elements in this study play important roles in the growth and development of plants, while some are not essential or even disturb plants. The cluster analysis of the changes of elements suggests that some elements have a common accumulation trend. The cluster analysis of elements in the roots shows that Pb, Ti, V, Fe and Co have similar accumulation rules (all are in a single neuron). The atomic weights of Ti, V, Fe and Co have little difference (47-58), so their absorption mechanisms in the roots may be similar, but the atomic weight of Pb is much larger than those of these four elements (207), and it is not beneficial to the growth and development of plants. This result indicates that the absorption and accumulation of Pb in roots by S. tonkinensis may be related to the four elements, but the mechanism is not clear.
In the correlations of the content changes among the elements in the roots, Fe, V, Al, Ti and Pb are positively correlated, which is consistent with the results of SOM clustering (located in two adjacent neurons with the same color). In the roots, B and Sr are also positively correlated with Cu, and Sr is positively correlated with Mo. Some elements are quite different from others in atomic weight (B and Sr compared to Pb), which suggests that absorption and accumulation do not depend entirely on the atomic weight. Other studies also show that there are correlations between elements with large atomic weight differences. The content of Cd2+ was positively correlated with the contents of Fe3+, Zn2+, Mn2+ and Cu2+ in rice roots, and a positive correlation was found in rice leaves between Fe3+, Zn2+ and Cu2+ [Citation43]. The Zn carrier protein in Thalspi caerulescens can enhance the transport of Cd [Citation44]. There was a strong correlation among the contents of some elements in Arabidopsis thaliana, but the correlations between different tissues were weak [Citation45]. The correlations of element variations in the stem and leaves were different from those in the roots, but Al, V and Fe showed a positive correlation in all three tissues. The absorption and accumulation of these three elements may depend on the same mechanism.
Plant element contents are related to genes, on the one hand, and to the environment and gene interactions [Citation45]. The interaction between elements has also been studied in other plants, and the most reported is the antagonistic effect of Se on Hg absorption [Citation46]. The element correlations in Arabidopsis thaliana are not consistent with our results [Citation45], which indicates that the genetic background and growth environment of S. tonkinensis are different from those of the plants studied above. It is also found that As and Hg in the roots of S. tonkinensis may have negative effects on the absorption and accumulation of some essential trace elements (Mo, Zn, Cu, Sr, and B), which means that these harmful elements can inhibit the normal growth and development of plants by affecting the absorption and accumulation of essential microelements. Especially, Mo, Zn, Cu and B play important roles in the physiological functions related to flowers, so these harmful elements may affect the flowering and fruiting of S. tonkinensis. Phosphate-dependent metabolism can be interrupted by As and some enzymes can be inactivated by As [Citation47,Citation48]. Hg affect photosynthesis and oxidative metabolism [Citation49]. These effects of As and Hg may also affect flowering.
The results of annotation from c130725_ g1 and c115342_g1 show that the pathways involving ALOG proteins and FRIGIDA-like proteins may play a major role in flowering control and flower development in S. tonkinensis. Genes such as c110050_g1, c66984_ g1, c99932_ g1, c6168_ g1 and c63291_ g1 are involved in the process of protein synthesis (mRNA splicing and translation), degradation and folding, which indicates that there is a strict regulation mechanism of protein synthesis and folding in the flowering process of S. tonkinensis. The functions of c119764_ g2 and c108307_ g1 suggest that the auxin signalling pathway and phosphatidylinositol signalling pathway play a more important role in the flowering of S. tonkinensis. The function of the c123287_ g1 gene indicates that GAUT may play a more important role in late pollination due to its important role in pollen tube formation during flower formation. The gene c127266_ g4 may regulate the transcription of other genes in the formation of flowers ().
Conclusions
In this study, we examined the absorption and accumulation of mineral elements during the flowering period of S. tonkinensis and discovered some important genes involved in the flowering and flower development of S. tonkinensis through WGCNA. The next step is to study the effects of the As and Hg contents in soil on the flowering and seed setting rate of S. tonkinensis. According to the identified genes and their functions, the effect of auxin on flower development needs to be studied in S. tonkinensis. On the other hand, we should further analyze the ALOG gene family and FRIGIDA-like gene family of S. tonkinensis. Further collection of transcriptomic and genomic data is needed for more accurate gene sequences. Finally, the mechanism of flower bud differentiation of S. tonkinensis will be revealed through these studies, which will lay a theoretical foundation for the regulation of the flowering and fruiting of S. tonkinensis in the cultivation process.
Data
The datasets generated in this study are available from the corresponding author upon reasonable request. The contents of all elements in our study and the data for WGCNA have been published in Mendeley Data and are available at http://dx.doi.org/10.17632/4gz4cj59yh.1
Supplemental Material
Download MS Word (16.1 KB)Disclosure statement
No potential conflict of interest was reported by the authors.
Additional information
Funding
Notes on contributors
Lin-xuan Li
Lin-xuan L wrote the manuscript and analyzed the data. Zhu Q conceived ideas for data analysis and conducted the RNA-seq. Jin-yuan C and Xiao-yu G both performed the ICP-MS. Namuhan C, Ying L and Xiao-yun G both collected the samples in the study. Kunhua W and Jianhua M both conceived ideas and experimental design.
References
- Huang B, Nong D, Huang X, et al. Resources investigation report on Chinese materia medica sophora tonkinensis. Modern Chinese Med. 2014;16:740–744.
- Chae H-S, Yoo H, Choi YH, et al. Maackiapterocarpan B from Sophora tonkinensis suppresses inflammatory mediators via nuclear factor-κB and mitogen-activated protein kinase pathways. Biol Pharm Bull. 2016;39(2):259–266.
- Ding P-L, Huang H, Zhou P, et al. Quinolizidine alkaloids with anti-HBV activity from Sophora tonkinensis. Planta Med. 2006;72(9):854–856.
- Wang W, Nakashima K-I, Hirai T, et al. Anti-inflammatory effects of naturally occurring retinoid X receptor agonists isolated from sophora tonkinensis gagnep. via retinoid X receptor/liver X receptor heterodimers. J Nat Med. 2019;73(2):419–430.
- Wang Y, You M. Chemoprevention of oral cancer by a mixture of Chinese herbs (anti-tumor mixture B). Prog Chem. 2013;25(09):1594.
- Wakhloo JL. Studies on the growth, flowering, and production of female sterile flowers as affected by different levels of foliar potassium in Solanum sisymbrifolium lam: II. Interaction between foliar potassium and applied gibberellic acid and 6-furfurylaminopurine. J Exp Bot. 1975;26(3):433–440.
- Sancenón V, Puig S, Mateu-Andrés I, et al. The arabidopsis copper transporter COPT1 functions in root elongation and pollen development. J Biol Chem. 2004;279(15):15348–15355.
- Sharma CP, Sharma PN, Chatterjee C, et al. Manganese deficiency in maize affects pollen viability. Plant Soil. 1991;138(1):139–142.
- Sharma PN, Chatterjee C, Sharma CP, et al. Zinc deficiency and anther development in maize. Plant Cell Physiol. 1987;28(1):11–18.
- Pandey N, Sharma CP. Iron deficiency induced changes in reproductive biology of green gram. Indian J Plant Physiol (India). 2001; 6(1):107–110.
- Takahashi M, Terada Y, Nakai I, et al. Role of nicotianamine in the intracellular delivery of metals and plant reproductive development. Plant Cell. 2003;15(6):1263–1280.
- Kaiser BN, Gridley KL, Ngaire Brady J, et al. The role of molybdenum in agricultural plant production. Ann Bot. 2005;96(5):745–754.
- Holdaway-Clarke TL, Weddle NM, Kim S, et al. Effect of extracellular calcium, pH and borate on growth oscillations in Lilium formosanum pollen tubes. J Exp Bot. 2003;54(380):65–72.
- Dubey S, Shri M, Gupta A, et al. Toxicity and detoxification of heavy metals during plant growth and metabolism. Environ Chem Lett. 2018;16(4):1169–1192.
- Zhu M, Xie H, Wei X, et al. WGCNA analysis of salt-responsive core transcriptome identifies novel hub genes in rice. Genes. 2019;10(9):719.
- Lin C-T, Xu T, Xing S-L, et al. Weighted gene co-expression network analysis (WGCNA) reveals the hub role of protein ubiquitination in the acquisition of desiccation tolerance in boea hygrometrica. Plant Cell Physiol. 2019;60(12):2707–2719.
- Lu C, Pu Y, Liu Y, et al. Comparative transcriptomics and weighted gene co-expression correlation network analysis (WGCNA) reveal potential regulation mechanism of carotenoid accumulation in Chrysanthemum × morifolium. Plant Physiol Biochem. 2019;142:415–428..
- Tai Y, Liu C, Yu S, et al. Gene co-expression network analysis reveals coordinated regulation of three characteristic secondary biosynthetic pathways in tea plant (Camellia sinensis). BMC Genomics. 2018;19(1):616.
- Chen N, Qiao Z, Xu J, et al. Physiological mechanism and developmental events in differentiating floral buds of Sophora tonkinensis gagnep. Pharmacognosy Magazine. 2020;(67):83–91.
- Langfelder P, Horvath S. WGCNA: an R package for weighted correlation network analysis. BMC Bioinform. 2008;9(1):559.
- Kohonen T, Lehtio P. Storage and processing of information in distributed associative memory systems. In GE Hinton & JA Anderson (eds.), Parallel models of associative memory, Chap. 4, pp. 105-143. Erlbaum, Hillsdale: New Jersey. 1981:129–167.
- Wehrens R, Kruisselbrink J. Flexible self-organising maps in Kohonen 3.0. J Stat. 2018;87(7):1–18.
- Wei T, Simko V. 2017. R package “corrplot”: visualization of a correlation matrix (version 0.84). Available from: https://github.com/taiyun/corrplot
- Bolger AM, Lohse M, Usadel B. Trimmomatic: a flexible trimmer for illumina sequence data. Bioinformatics. 2014;30(15):2114–2120.
- Grabherr MG, Haas BJ, Yassour M, et al. Full-length transcriptome assembly from RNA-Seq data without a reference genome. Nat Biotechnol. 2011;29(7):644–652.
- Pertea G, Huang X, Liang F, et al. TIGR gene indices clustering tools (TGICL): a software system for fast clustering of large Est datasets. Bioinformatics. 2003;19(5):651–652.
- Zdobnov EM, Apweiler R . InterProScan-an integration platform for the signature-recognition methods in InterPro. Bioinformatics. 2001;17(9):847–848.
- Li B, Dewey CN. RSEM: accurate transcript quantification from RNA-Seq data with or without a reference genome. BMC Bioinform. 2011;12(1):323.
- Isermann K. Uptake of stable strontium by plants and effects on plant growth. In: Stanley Skoryna (ed.), Handbook of stable strontium. Boston, MA: Springer; 1981. p. 65–86.
- MacAlister CA, Park SJ, Jiang K, et al. Synchronization of the flowering transition by the tomato terminating flower gene. Nat Genet. 2012;44(12):1393–1398.
- Yoshida A, Sasao M, Yasuno N, et al. TAWAWA1, a regulator of rice inflorescence architecture, functions through the suppression of meristem phase transition. Proc Natl Acad Sci U S A. 2013;110(2):767–772.
- Barbez E, Kubeš M, Rolčík J, et al. A novel putative auxin carrier family regulates intracellular auxin homeostasis in plants. Nature. 2012;485(7396):119–122.
- Lang K, Schmid FX, Fischer G. Catalysis of protein folding by prolyl isomerase. Nature. 1987;329(6136):268–270.
- Wang Y, Liu C, Yang D, et al. Pin1At encoding a peptidyl-prolyl cis/trans isomerase regulates flowering time in arabidopsis. Mol Cell. 2010;37(1):112–122..
- Gui J-F, Lane WS, Fu X-D. A serine kinase regulates intracellular localization of splicing factors in the cell cycle. Nature. 1994;369(6482):678–682.
- Nakagawa O, Arnold M, Nakagawa M, et al. Centronuclear myopathy in mice lacking a novel muscle-specific protein kinase transcriptionally regulated by MEF2. Genes Dev. 2005;19(17):2066–2077.
- Wang H-Y, Lin W, Dyck JA, et al. SRPK2: a differentially expressed SR protein-specific kinase involved in mediating the interaction and localization of pre-mRNA splicing factors in mammalian cells. J Cell Biol. 1998;140(4):737–750.
- Shears SB. How versatile are inositol phosphate kinases? Biochem J. 2004;377(Pt 2):265–280.
- Shindo C, Aranzana MJ, Lister C, et al. Role of frigida and flowering locus C in determining variation in flowering time of arabidopsis. Plant Physiol. 2005;138(2):1163–1173.
- Atmodjo MA, Sakuragi Y, Zhu X, et al . Galacturonosyltransferase (GAUT)1 and GAUT7 are the core of a plant cell wall pectin biosynthetic homogalacturonan:galacturonosyltransferase complex. Proc Natl Acad Sci U S A. 2011;108(50):20225–20230.
- Wang L, Wang W, Wang Y-Q, et al. Arabidopsis galacturonosyltransferase (GAUT) 13 and GAUT14 have redundant functions in pollen tube growth. Mol Plant. 2013;6(4):1131–1148.
- Bundock P, Hooykaas P. An arabidopsis hAT-like transposase is essential for plant development. Nature. 2005;436(7048):282–284.
- Liu JG, Liang JS, Li KQ, et al. Correlations between cadmium and mineral nutrients in absorption and accumulation in various genotypes of rice under cadmium stress. Chemosphere. 2003;52(9):1467–1473.
- Lasat MM, Pence NS, Garvin DF, et al. Molecular physiology of zinc transport in the Zn hyperaccumulator Thlaspi caerulescens. J Exp Bot. 2000;51(342):71–79.
- Baxter I, Hermans C, Lahner B, et al. Biodiversity of mineral nutrient and trace element accumulation in Arabidopsis thaliana. PLoS One. 2012;7(4):e35121.
- Yang DY, Chen YW, Gunn JM, et al. Selenium and mercury in organisms: interactions and mechanisms. Environ Rev. 2008;16:71–92.
- Finnegan PM, Chen W. Arsenic toxicity: the effects on plant metabolism. Front Physiol. 2012;3:182.
- Meharg AA, Hartley-Whitaker J. Arsenic uptake and metabolism in arsenic resistant and nonresistant plant species. New Phytol. 2002;154(1):29–43.
- Poschenrieder C, Barcel J. Water relations in heavy metal stressed plants. In: Prasad MNV, editor. Heavy metal stress in plants. 3rd ed. Berlin: Springer; 2004. p. 207–270.