Abstract
Fungi produce a wide repertoire of secondary metabolites with pharmaceutical impacts on human health. However, the low expression of secondary metabolites limits their clinical application. Triterpenoids produced by Sanghuangporus baumii possess anti-cancer properties, however, their biosynthetic pathway and regulatory mechanism remain unclear. In this study, squalene synthase gene (SbSQS), a key gene involved in the mevalonate pathway, was cloned and analyzed. Sequence analysis identified SbSQS as a typical squalene synthase with three conserved catalytic structures related to binding and catalysis. The SbSQS gene encodes a 489 amino acid polypeptide with a predicted protein molecular weight of 55.05 kDa. The promoter of SbSQS contains cis-acting regulatory elements involved in the light, abscisic acid and MeJA responsiveness, which is consistent with the promoters of other genes in the mevalonate pathway. Furthermore, SbSQS was cloned into the pET-32a vector and overexpressed in Escherichia coli. During mycelium fermentation of S. baumii, the biomass increased as fermentation progressed, and peaked on day 18 (1.20 g/250 mL). The triterpenoid content did not accumulate during the fermentation process and was 20.8 mg · g−1 on day 18. The SbSQS expression and the triterpenoid content were peaked on day 6, suggesting a correlation between SbSQS expression level with triterpenoid content. This study proposes a key candidate gene for genetic manipulation of the triterpenoid synthesis to enhance the triterpenoid content in S. baumii.
Introduction
Secondary metabolites produced by fungi have a long and intimate connection with humans [Citation1]. The medicinal properties of fungi have been discovered and exploited since ancient times. More recently, some effective fungal secondary metabolites (e.g. penicillin, statins, triterpenoid, and Ganoderic Acid) have been used to treat disease and extend human life [Citation2]. However, the low expression of secondary metabolites limits the progress of fungal metabolites in clinical application. Sanghuangporus baumii is a medicinal mushroom that produces a variety of secondary metabolites with medicinal effects. Triterpenoids are the most notable of these metabolites. Research has shown that triterpenoids in S. baumii have anti-tumour, anti-oxidative and anti-inflammatory properties [Citation3–5]. However, the low expression of triterpenoids also limits their clinical application.
Triterpenoids are synthesized via the mevalonate (MVA) pathway, in which acetyl-CoA is converted through a series of chemical reactions to several different terpenoids, such as volatile scented monoterpenoids, sesquiterpenoids with antimalarial properties and triterpenoids with antineoplastic characteristics [Citation6–8]. Squalene synthase (SQS) is a key enzyme in the MVA pathway, which is responsible for condensing two molecules of farnesyl diphosphate (FPP) into squalene, the precursor of sterols and triterpenoids. SQS genes have been identified from a wide range of species, including bacteria [Citation9], fungi [Citation10, Citation11], animals and plants [Citation12]. It is a key enzyme, represents a putative branch point managing carbon flux from isoprenoids biosynthetic pathway into triterpenoid biosynthesis [Citation13]. Therefore, many researches attempt to influence the triterpenoid biosynthesis by changing the expression level of SQS. In the adventitious roots of medicinal plants Panax ginseng, overexpression of SQS was followed by the up-regulation of all the downstream genes in the triterpenoid synthesis pathway, and resulted in remarkable increase of ginsenoside (triterpene saponins) contents [Citation14]. In alfalfa (Medicago truncatula), SQS expression was up-regulated by methyl jasmonate (MeJA) treatment, which led to the increase of SQS enzyme content and the accumulation of triterpenes. Moreover, overexpression of SQS by transgenic alfalfa will also increase the content of terpenoids in alfalfa [Citation15]. Similarly, overexpression of SQS can cause the accumulation of target terpenes in the medicinal fungi Poria cocos [Citation10], the metabolically engineered strain Bacillus subtilis [Citation9], and the medicinal plant Bupleurum falcatum [Citation16]. These results indicated that SQS is important in the regulation of the terpenoids biosynthetic pathway, and it is feasible to overexpress SQS to increase the content of terpenoids. However, little is known about the key enzyme SQS and the triterpenoid synthesis pathway in S. baumii.
Investigation of the triterpenoid biosynthesis pathway may promote the production of triterpenoids in S. baumii, which would improve the medicinal effect of S. baumii. In our previous work, the transcriptome of S. baumii was sequenced using Illumina high-throughput technology, and 30,051 Unigenes were assembled and annotated [Citation17]. Among them, 12 Unigenes were found to be involved in triterpenoid biosynthesis, including SQS gene. In the experiment of triterpenoid synthesis induced by MeJA, we obtained the optimal concentration of 150 mmol/L MeJA, which could increase the triterpenoid content about 4 times [Citation18]. In this study, we cloned and analyzed the structure of the SQS from S. baumii, and successfully expressed SbSQS in Escherichia coli. Fermentation is an efficient method for triterpenoid production [Citation19]. Therefore, the biomass, triterpenoid content, and SbSQS transcript levels during fermentation of S. baumii were also measured.
Materials and methods
Strains and culture conditions
The strain used in this study was identified as S. baumii (GenBank accession number KP974834) by ITS sequence alignment. As previously described [Citation20], mycelia were cultured on potato dextrose agar (PDA) plates to rejuvenate, and then transferred to potato dextrose broth (PDB) medium, and cultured in a shaking incubator at 25 °C and 160 r/min, to harvest mycelia. Escherichia coli DH5α and BL21 (DE3) were from TIANGEN (Beijing, China) and were cultured on Luria-Bertani (LB) medium at 37 °C, as previously described [Citation20].
Nucleic acids preparation and gene cloning
The experimental process of nucleic acid extraction and gene cloning was as previously described [Citation20]. Total DNA and RNA were extracted with the cetyl trimethylammonium bromide method and TRIzol reagent (TaKaRa, Dalian, China). Complementary DNA (cDNA) was synthesized with PrimeScript II first strand cDNA synthesis kit (TaKaRa). Primers SQS-F and SQS-R were designed to obtain the complete full-length cDNA and DNA sequence. Promoter-F and promoter-R were designed to obtain the homologous promoter of SbSQS ().
Table 1. List of primers used in the study.
Bioinformatics analysis
In parallel to our previous report [Citation20], here we investigated the gene sequence, promoter sequence and phylogenetic analysis of SQS. In addition, the theoretic molecular weight, isoelectric point, absorption coefficient, subcellular localization and protein structure of SQS protein were also predicted.
The following tools were used: the basic local alignment search tool (BLAST) on the NCBI website (http://www.ncbi.nlm.nih.gov/blast), with the SbSQS sequence as a query; MEGA 5.0 software [Citation3] to construct a neighbor-joining tree; ORF Finder (http://www.ncbi.nlm.nih.gov/orffinder/) for open reading frame (ORF) prediction; PlantCARE (http://bioinformatics.psb.ugent.be/webtools/plantcare/html/) for promoter analysis; DNAMAN (Version 7) (Lynnon Corporation, Quebec, Canada) for sequence alignment; ExPASy Proteomics Server (http://www.expasy.ch/tools/protparam.html), ExPASy PROSITE (http://www.expasy.ch/prosite/), PSIPRED (http://bioinf.cs.ucl.ac.uk/psipred/), TMHMM (http://www.cbs.dtu.dk/services/TMHMM/) and SWISS-MODEL online homologous modelling software (http://swissmodel.expasy.org) to predict the protein characteristics; and ProtComp 9.0 (http://www.softberry.com/berry.phtml) for subcellular localization prediction.
Heterologous expression of SbSQS in E. coli
The entire SbSQS ORF was incorporated into the pET-32a vector (TaKaRa) and transferred into E. coli DH5α for plasmid propagation. Afterwards, the recombinant plasmid pET-32a-SbSQS was transferred into E. coli BL21, and was induced by isopropyl β-D-1-thioga-lactopyranoside (IPTG) to express the recombinant protein. Samples were collected every two hours during induction. The induction results were observed by 12% sodium dodecyl sulphate polyacrylamide gel electrophoresis (SDS-PAGE).
Determination of biomass, triterpenoid and SbSQS expression during fermentation
The experimental procedure was as previously described [Citation20]. During fermentation, samples were collected at 3, 6, 9, 12, 15 and 18 days. Mycelia dried at 45 °C were used to measure biomass and triterpenoid, and mycelia frozen at −80 °C were used to extract total RNA. The total triterpenoid was determined by the vanillin-acetic acid method. Primers SQS-qF and SQS-qR were used for the Real-Time Quantitative PCR (RT-qPCR) amplification, and α-tubulin was used as a reference gene ().
Data analysis
Quantitative data are presented as mean values from three independent experiments with standard deviation of the means. Statistical analysis was performed using Tukey tests (STATGRAPHICS PLUS, STATPOINT TECHNOLOGIES, INC., Virginia) and values were considered statistically significantly different at the p < 0.05 level.
Results
Sequence analysis
Bioinformatics analysis was performed on the cloned gene sequence. Based on protein BLAST search results, the sequence encodes a squalene synthase, and shares 77.39% percent identity with the squalene synthase of Inonotus obliquus. Thus, it was named as SbSQS (GenBank accession number: MT040049).
SbSQS contains four exons (nucleotides 1–231, 294–1092, 1148–1275,1344–1660) and three introns (232–293, 1093–1147, 1276–1343; ). The 1,470 bp ORF encodes a 489 amino acid polypeptide (). Using the SbSQS protein sequence as a query, sequences of orthologs from 12 species () were selected to construct a phylogenetic tree (), of which 11 belong to Basidiomycota, and one (Penicillium brevicompactum) belongs to Ascomycota. The red, blue and dark green branches of the phylogenetic tree correspond to the orders Hymenochaetales, Polyporales and Agaricales, respectively. The results indicated that SbSQS shares the closest relative with the SQS enzyme in highly similar species Inonotus obliquus, and the furthest relative with the SQS enzyme in P. brevicompactum.
Figure 1. Sequence analysis of SbSQS and its promoter region. (A) Exon and intron sites of SbSQS. (B) Phylogenetic analysis of SQS from S. baumii and other species. (C) Functional elements of the SbSQS promoter.
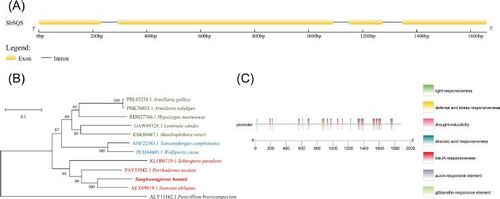
Table 2. Origins of homologous amino-acid sequences.
The 1,879 bp SbSQS promoter contains common cis-acting elements (CAAT-boxes) and core promoter elements (TATA boxes). In addition, it also includes cis-acting regulatory elements involved in light responsiveness (G-boxes), abscisic acid responsiveness (ABRE), MeJA-responsiveness (CGTCA-motif), defense and stress responsiveness (TC-rich repeats), gibberellin responsiveness (P-box) and auxin responsiveness (TGA-element) ().
Protein structure analysis
The molecular formula of the protein encoded by SbSQS is C2461H3852N672O706S28, and the molecular weight of the protein is 55.05 kDa. The protein has a theoretical isoelectric point of 6.21 and contains 62 negatively charged amino acid residues (Asp + Glu) and 57 positively charged amino acid residues (Arg + Lys). The SbSQS is a stable protein with an instability index of 34.10. A signal peptide is predicted at the position 1 to 21 of the amino acid sequence, and the most likely cleavage site is between position 21 and 22, which indicated that SbSQS is a secretory protein ().
Figure 2. Sequence alignment of the 12 amino acid sequences of SQS from the indicated species. Homology level was highlighted by shading in color: black for 100%, pink for ≥ 75% and blue for ≥ 50% identity. Conserved functional segments (A, B and C) are framed in red.
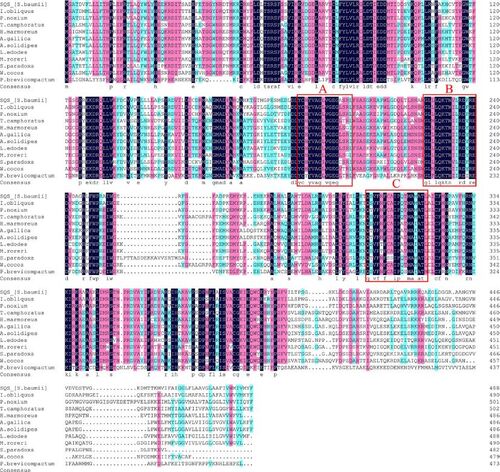
Comparison of the amino acid sequences of SbSQS with those of other species () showed that the sequences of these SQSs were highly similar especially in the three functional segments (A, B and C, ) that are considered essential for the catalytic function of SQS [Citation21]. Topological analysis of transmembrane proteins revealed that SbSQS had two transmembrane regions, amino acids 308–330 and 462–484 (). These two regions show strong hydrophobicity (), despite the grand average of hydropathicity of the protein is −0.065. Subcellular localization prediction indicated that SbSQS localized in the endoplasmic reticulum (). The secondary structure of SbSQS contains 62.37% alpha helix, 26.58% random coil, 7.36% extended strand and 3.68% beta sheet region (). The predicted SbSQS three-dimensional structure is predominantly composed of alpha helices, which are arranged in near parallel and form a channel running through the center of the protein ().
Figure 3. Analysis of the secondary structure and predicted three-dimensional structure of SbSQS. (A) The predicted transmembrane regions of SbSQS. (B) Annotation of the secondary structure of SbSQS. (C) Predicted three-dimensional structure of SbSQS.
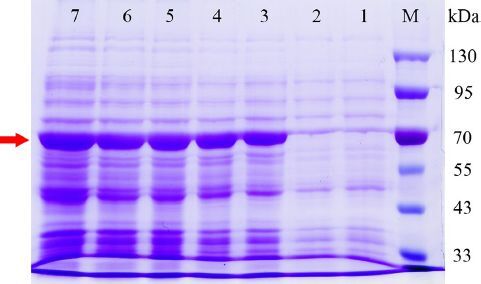
Figure 4. Heterologous expression of SbSQS in E. coli following IPTG induction (1 mmol/L). The bands indicated by the red arrow are target proteins. Lane M, protein molecular weight markers; Lane 1, blank control group: E. coli harboring empty vector; Lane 2–7, E. coli harboring pET-32a-SbSQS induced by IPTG at 0 h, 2 h, 4 h, 6 h, 8 h, 10 h.
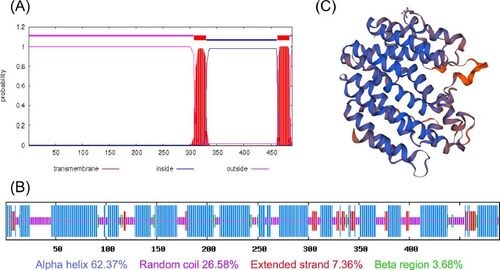
Heterologous expression of SbSQS in E. coli
We used E. coli as a host for heterologous expression of SbSQS, and the expressed protein could be used to study the catalytic function after purification. A clear protein band with an expected molecular weight (55.05 kDa plus a 22.68 kDa tag protein) was observed in the 12% SDS-PAGE gel (). The expression of recombinant protein increased with the extension of induction time, and there was no target band in the blank control group. The results showed that the squalene synthase of S. baumii was successfully expressed in E. coli.
Biomass and triterpenoid content in fermentation
shows the kinetics of biomass and triterpenoid content of S. baumii mycelia in the fermentation process. The mycelia remained in a lag phase at the initial stage of fermentation, and the biomass grew slowly in the first 12 days. On day 12, mycelia entered the rapid growth stage, and the biomass increased rapidly from 0.50 g/250 mL to 1.05 g/250 mL dry weight (DW) in three days. The biomass kept increasing at the late fermentation stage and reached the highest level of 1.20 g/250 mL. The bar chart shows the triterpenoid content of mycelia during fermentation. The triterpenoid content of mycelia fluctuated between 19.63 mg · g−1 (day 15) and 27.48 mg · g−1 (day 6) during the whole fermentation period, and stabilized at about 20 mg · g−1 at the end of fermentation. Overall, the triterpenoid content of mycelia did not accumulate with the fermentation process.
SbSQS expression during S. baumii fermentation
RT-qPCR was used to determine the expression of SbSQS in S. baumii during fermentation. The relative expression of SbSQS reached the highest value of 1.92-fold on day 6 (compared with day 3) and decreased to the lowest value of 0.78-fold on day 12 (). The results showed that the expression level of SbSQS had a trend of decreasing in early period (day 6 to day 12) and increasing in late period (day 12 to day 18) during 18-day fermentation period.
Discussion
Triterpenoids produced by S. baumii are important secondary metabolites with medicinal value, but their content is low in the fruiting body. Constructing engineered strains through molecular biology is an effective way to obtain sufficient metabolites. Therefore, it is necessary to explore the terpenoids biosynthesis pathways in S. baumii. In this study, the squalene synthase gene of S. baumii was cloned and characterized. It is the first squalene synthase encoding gene identified from S. baumii. In addition, the SbSQS expression level is associated with the triterpenoid content during S. baumii fermentation. This is in agreement with our previous report [Citation20] on the expression pattern of phosphomevalonate kinase gene (another key gene in the MVA pathway) during fermentation. Our results suggest that the low expression of key genes in the MVA pathway is responsible for the low triterpenoid content in S. baumii.
The phylogenetic analysis showing SQSs in basidiomycetes were grouped separately from the subclass of SQS in ascomycetes. This is consistent with morphology because ascomycetes, unlike basidiomycetes in this study, ascomycetes are filamentous fungi that do not produce large fruiting bodies. The overall architecture of SQS enzyme has been reported to be highly identical in eukaryotes. For example, the alpha helix and random coil are the main components of SQS secondary structure, accounting for 68.66% and 22.00% of total peptide, respectively. In addition, SQS proteins share two transmembrane domains and three conserved domains (A, B, and C) that are essential for catalysis. In summary, our study suggests that SbSQS encodes a canonical squalene synthase sharing highly conserved functional domains with its orthologs in other species.
Our previous work has shown that MeJA was indeed a potent inducer of triterpenoid biosynthesis. It up-regulated genes in the MVA pathway and increased the content of triterpenoid of Inonotus baumii [Citation18]. Ren et al. also reported that MeJA could induce the biosynthesis of ganoderic acid, a type of triterpenoid, through the transcriptional induction of genes in Ganoderma lucidum [Citation22]. The SbSQS promoter includes responsive elements involved in the MeJA-responsiveness (CGTCA-motif). In addition, according to our previous studies, similar MeJA response elements were found in the promoters of the phosphomevalonate kinase gene [Citation20], acetyl transferase gene [Citation23] and farnesyl-diphosphate synthase gene [Citation24] in the MVA pathway. These studies suggest that MeJA may act on MeJA responsive elements in the promoters to regulate triterpenoid biosynthesis by altering gene expression.
Submerged fermentation appears to be an efficient method to produce biomass. During S. baumii fermentation, the biomass kept increasing and reached the highest level of 1.20 g/250 mL. However, triterpenoids did not accumulate during the fermentation process, but stabilized at about 20 mg · g−1 at the later stage of fermentation. The low triterpenoid content also limits the progress in the clinical application of other medicinal fungi such as S. sanghuang [Citation25] and G. lucidum [Citation22]. Future work is to improve triterpenoid production by regulating triterpenoid synthesis pathway.
Conclusions
In our study, the SbSQS of S. baumii was cloned and analyzed for the first time. Meanwhile, the SbSQS transcription level and triterpenoid content during mycelium fermentation were also obtained. The results provide the basis for the regulation of triterpenoid biosynthesis pathway in S. baumii. To this end, strains overexpressing SbSQS are being constructed in our laboratory.
Authors’ contributions
S. W., X. W., Z. L. performed and analyzed most of the experiments in this study. T. S. and L. Z. designed this work and provided all financial support. The manuscript was written by S. W.
Supplemental Material
Download PDF (1.2 MB)Data availability statement
All data that support the findings reported in this study are available from the corresponding author upon reasonable request.
Disclosure statement
The authors report no conflict of interest.
Additional information
Funding
References
- Keller NP. Fungal secondary metabolism: regulation, function and drug discovery. Nat Rev Microbiol. 2019;17(3):167–180.
- Muszyńska B, Grzywacz-Kisielewska A, Kała K, et al. Anti-inflammatory properties of edible mushrooms: a review. Food Chem. 2018;243:373–381.
- Liu C, Zhao C, Pan HH, et al. Chemical constituents from inonotus obliquus and their biological activities. J Nat Prod. 2014;77(1):35–41.
- Kim YJ, Park J, Min BS, et al. Chemical constituents from the sclerotia of inonotus obliquus. J Korean Soc Appl Biol Chem. 2011;54(2):287–294.
- Handa N, Yamada T, Tanaka R. An unusual lanostane-type triterpenoid, spiroinonotsuoxodiol, and other triterpenoids from inonotus obliquus. Phytochemistry. 2010;71(14–15):1774–1779.
- Landolfo S, Zara G, Zara S, et al. Oleic acid and ergosterol supplementation mitigates oxidative stress in wine strains of Saccharomyces cerevisiae. Int J Food Microbiol. 2010;141(3):229–235.
- Ajikumar PK, Xiao WH, Tyo KEJ, et al. Isoprenoid pathway optimization for taxol precursor overproduction in Escherichia coli. Science. 2010;330(6000):70–74.
- Muntendam R, Melillo E, Ryden A, et al. Perspectives and limits of engineering the isoprenoid metabolism in heterologous hosts. Appl Microbiol Biotechnol. 2009;84(6):1003–1019.
- Song YF, Guan Z, van Merkerk R, et al. Production of squalene in Bacillus subtilis by squalene synthase screening and metabolic engineering. J Agric Food Chem. 2020;68(15):4447–4455.
- Wang JR, Lin JF, Guo LQ, et al. Cloning and characterization of squalene synthase gene from poria cocos and its up-regulation by methyl jasmonate. World J Microbiol Biotechnol. 2014;30(2):613–620.
- Song JF, Shang N, Baig N, et al. Aspergillus flavus squalene synthase as an antifungal target: Expression, activity, and inhibition. Biochem Biophys Res Commun. 2019;512(3):517–523.
- Qian JY, Liu Y, Ma CT, et al. Positive selection of squalene synthase in cucurbitaceae plants. Int J Genomics. 2019;2019:5913491.
- Devarenne TP, Ghosh A, Chappell J. Regulation of squalene synthase, a key enzyme of sterol biosynthesis, in tobacco. Plant Physiol. 2002;129(3):1095–1106.
- Lee MH, Jeong JH, Seo JW, et al. Enhanced triterpene and phytosterol biosynthesis in panax ginseng overexpressing squalene synthase gene. Plant Cell Physiol. 2004;45(8):976–984.
- Kang JM, Zhang QY, Jiang X, et al. Molecular cloning and functional identification of a squalene synthase encoding gene from alfalfa (Medicago sativa L.). Int J Mol Sci. 2019;20(18):4499.
- Kim YS, Cho JH, Park S, et al. Gene regulation patterns in triterpene biosynthetic pathway driven by overexpression of squalene synthase and methyl jasmonate elicitation in bupleurum falcatum. Planta. 2011;233(2):343–355.
- Zou L, Sun TT, Li DL, et al. De novo transcriptome analysis of inonotus baumii by RNA-seq. J Biosci Bioeng. 2016;121(4):380–384.
- Sun TT, Zou L, Zhang LF, et al. Methyl jasmonate induces triterpenoid biosynthesis in inonotus baumii. Biotechnol Biotechnol Equip. 2017;31(2):312–317.
- Wu MD, Cheng MJ, Chen YL, et al. Secondary metabolites from the fermented whole broth of fungal strain sanghuangporus sanghuang. Chem Nat Compd. 2019;55(1):36–40.
- Wang SX, Liu ZC, Wang XT, et al. Cloning and characterization of a phosphomevalonate kinase gene from sanghuangporus baumii. Biotechnol Biotec Equip. 2021;35(1):934–942.
- Gu P, Ishii Y, Spencer TA, et al. Function-structure studies and identification of three enzyme domains involved in the catalytic activity in rat hepatic squalene synthase. J Biol Chem. 1998;273(20):12515–12525.
- Ren A, Qin L, Shi L, et al. Methyl jasmonate induces ganoderic acid biosynthesis in the basidiomycetous fungus Ganoderma lucidum. Bioresour Technol. 2010;101(17):6785–6790.
- Wang XT, Wang SX, Xu XR, et al. Molecular cloning, characterization, and heterologous expression of an acetyl-CoA acetyl transferase gene from Sanghuangporus baumii. Protein Expr Purif. 2020;170:105592.
- Ding YX, Ou-Yang X, Shang CH, et al. Molecular cloning, characterization, and differential expression of a farnesyl-diphosphate synthase gene from the basidiomycetous fungus Ganoderma lucidum. Biosci Biotechnol Biochem. 2008;72(6):1571–1579.
- Cai CS, Ma JX, Han CR, et al. Extraction and antioxidant activity of total triterpenoids in the mycelium of a medicinal fungus, Sanghuangporus sanghuang. Sci Rep. 2019;9(1):7418.