Abstract
A serious problem associated with orthopedic bone drilling manipulation is the so-called thermal osteonecrosis (TO). This problem occurs because the drilling manipulation generates heat, and the amount of heat depends on the drilling conditions. This work presents the experimental evaluation of the temperature during automated bone drilling by using a handheld Orthopedic bone Drilling Robot ODRO. Up to now, such experimental results related to temperature evaluation in bone drilling by robots have not been presented. Fresh porcine and bovine femur bones were specimens in the experiments. The increase in bone temperature during drilling was measured by a thermal infrared camera. Thermographic sequence images were recorded, processed and visualized using specialized software, which allows to capture accurately the moment of drill bit penetration through the bone and its extraction when going back to initial position. The results showed that under given feed rate and drill speed, when using a new drill bit, the temperature value during porcine femur drilling exceeded the critical threshold of 50 °C for less than 2 s, and thermal osteonecrosis does not occur. When using a worn-out drill bit, the temperature value during bovine femur drilling significantly exceeded the threshold of 50 °C, and thermal osteonecrosis occurs. Thus, to guarantee bone drilling without the risk of thermal osteonecrosis, the automated drilling should be executed with the simultaneous control of speed of drill bit and feed rate. This is possible to be achieved only by robotic execution of the manipulation.
Introduction
In surgical practice, an important manipulation concerning the treatment of bone fractures is the drilling of bones. That process includes various input and output parameters.
When a drilling manipulation is performed, most of the input parameters are defined in advance. These are the object of the manipulation, drill bit, drilling techniques, cooling mode and drill depth. Some of them, such as drill speed and feed rate, can be changed during the manipulation and they are the most important for the outcome, namely the mechanical or thermal damage to the bones. Mechanical damage arises due to micro cracks that occur during drilling as a result of excessive force of pressure (thrust force). Thermal damage arises due to temperature elevation during drilling, which causes thermal osteonecrosis of bone tissue. Thermal osteonecrosis is the death of bone tissue resulting from extremely high temperatures. It is a problem in orthopedic surgery, as it can have negative effects for the fracture fixation by implants in bones [Citation1]. Near the hole, the drilling process often causes thermal and mechanical damage of bones and may even lead to the loosening or fracturing of bone screws [Citation2].
The temperature when thermal osteonecrosis starts is referred to as critical temperature, and such thermal osteonecrosis depends on the temperature and on the duration as well. The level that marks the beginning of thermal osteonecrosis is still not a clear question. It is still under discussion in the scientific reports [Citation3].
As a starting point in a number of experimental studies, an increase in temperature to 47 °C for 1 min of exposure has been shown to cause bone necrosis. However, not so many studies present some results of clinically relevant outcomes in orthopedic surgery resulting from such exposure [Citation4]. In addition, when performing the manipulation manually, the duration of the bicortical drilling in the operating room is within 8–12 s, that is, such exposure cannot be achieved in practice. Many studies have focused on the determination of the critical temperature threshold, after which osteonecrosis occurs. The death of bone cells because of heat occurs instantly at 70 °C [Citation1, Citation5, Citation6], while a temperature value lower than 55 °C for a duration of 30 s could cause irreversible bone cell death [Citation6]. Necrosis occurs as a result of heat exposure of cells to 50 °C for 5 s, but not when cells are exposed to 50 °C for 2 s [Citation5]. The exposure to 50 and 60 °C for 2 s allows recovery [Citation5].
From the point of view of temperature, it is well-known that increasing in the feed rate reduces the drilling time, which in turn reduces the duration of heating; hence, the risk of thermal osteonecrosis becomes smaller [Citation7, Citation8]. In addition, the increase in drill speed leads to temperature increase during drilling [Citation7, Citation9, Citation10], that is, the risk of thermal osteonecrosis becomes higher; experimental results reveal a proportional connection between temperature and drilling speed [Citation10].
It should be borne in mind, however, that the increase in feed rate causes an increase in the thrust force, that is, higher risk of bone damage [Citation11], and also the increase in drill speed leads to a decrease in the thrust force [Citation11], thus, narrowing the time interval in which bone damage is possible to occur.
To prevent thermal osteonecrosis, it is necessary to apply a high feed rate together with low drill speed. To avoid traumatic osteonecrosis, it is necessary to apply a lower feed rate together with high drill speed. Thus, the optimal combination of conditions to prevent thermal osteonecrosis, can at the same time cause a high risk for traumatic osteonecrosis. In addition, given that all other conditions are the same, there are some other factors that are specific to each different drilling process, for instance such as the extent of wear of the drill bit. Such wear of the drill bit can lead to a critical increase in temperature. The extent of the temperature increase is proportional to the wear of the used drill bit [Citation12].
To improve the quality of the drilling manipulation, bone-drilling handheld robotized systems are being developed in the recent years. A handheld robot is a manipulator, either in parallel, serial or hybrid structure, where the base is held by the human operator [Citation13]. Handheld robots have high potential and similar functionality like conventional robots, low cost and are easy to handle by the human operator [Citation13]. Although some of these handheld robots are already being implemented in orthopedic surgical practice [Citation14–16], and others are under development [Citation17, Citation18], up to now no experimental results related to the increase in temperature have been presented during the robotic execution of bone drilling.
This work presents the experimental temperature evaluation during bicortical bone drilling executed by using a handheld robot ODRO.
Materials and methods
During bone drilling, some part of the mechanical work is converted into heat [Citation19]. Cutting the bone surface by a drill bit can destroy the intermolecular bonds, which leads to a release of energy as heat [Citation7]. Another source of heat is the friction of drill bit non-cutting surfaces in the hole [Citation7].
Heat is generated at the tool and bone interface while drilling. This heat leads to an increase in the temperature of the drill bit and the bone simultaneously. The temperature value which a material can reach is related to its specific coefficients of heat capacity and thermal conductivity [Citation7]. The specific heat capacity of the drill bit is much smaller (up to three times) than that of bone [Citation20]. As a result, the drill bit temperature rises much faster than that of the bone. The difference between the bone temperature and the drill bit temperature can reach up to 20 °C [Citation21], because the maximal temperature during drilling is at the drill bit top cutting edge [Citation21].
A heat gradient occurs between the bone and the drill bit, causing additional heat transfer to the bone. The transfer continues until the gradient exists and as long as contact is maintained between the bone and the drill bit. The longer it lasts, the more heat is transferred to the bone.
The bone heats up during drilling for three reasons: cutting the surface layer of the material by the drill bit; friction between the drill bit surface and the bone; and additional transfer of heat from the drill bit to the bone.
The final temperature that the bone reaches during drilling is a result of received and transmitted heat. If they are equal, the pattern reaches equilibrium. The temperature of a sample is a function of the exposure time and thermal conductivity. However, equilibrium of temperature usually will not occur if bones are exposed to heat in a real situation, where the period of exposure is practically short [Citation7]. When performing an operation manually, the duration of bicortical drilling in the operating room is 8–12 s.
The bone structure is anisotropic and has a low coefficient of thermal conductivity. That does not allow fast heat dissipation while drilling and increases the bone temperature [Citation3]. The bone temperature on the hole surface slowly increases along its axial axis and the highest temperature is at the end of the hole [Citation22].
Bicortical drilling, due to the higher density of cortical bone, is the most risky manipulation in terms of increasing the temperature in the drilled area and the potential likelihood of thermal osteonecrosis. The heat is accumulated as the hole becomes deeper and reaches its maximal value when the drill bit exits the hole [Citation5]. Therefore, the temperature measurement is focused on the drill bit penetration zone when the breakthrough of the second cortex occurs.
Specimens
The specimens for the experiments were fresh porcine and bovine femur bones taken from state-approved licensed meat stores, available freely from markets. Porcine bones are considered a model of the human bone and they both are similar in bone mineral density [Citation2, Citation23]. The thermal and mechanical characteristics of bovine bones are similar to those of human cortical bone [Citation24, Citation25]. However, human bone has lower density and cortical thickness than bovine bone.
A transverse incision was made in the middle of the diaphysis so that the movement of the drill bit inside the medullary canal could be traced after drilling the first cortex, as well as its emergence out of the second bone cortex when the drilling was finished.
Experimental setup
When performing experimental studies, the temperature during drilling is usually measured in two ways: by thermocouple and by thermal infrared (IR) camera. However, some reports have observed that using a thermocouple is not so practical in medical applications, because the thermal infrared camera is preferred as a non-invasive method [Citation26].
The experiments were executed using the handheld robot ODRO, which autonomously executes drilling on the basis of developed software that is incorporated [Citation27, Citation28]. The experimental setup with a thermal infrared camera is shown in .
To measure the increase in temperature in the bone while drilling, we used a thermal infrared camera FLIR P640 with an infrared detector of 640 × 480, thermal sensitivity of 0.06 °C at 30 °C and temperature range from −40 to 120 °C. In this study, thermographic sequence images of bone drilling were recorded. The duration of the sequence images was 16 s, with a frame rate of 15 fps. In the sequence images boxes, maximal and minimal temperatures were placed with markers. The constant distance was 0.5 m between the IR camera and the object when the experiment was executed. The emissivity coefficient “ε” of bone was set to 0.96 [Citation29].
The recorded thermographic sequence images were processed and visualized using Flir Tools software, which allows to accurately capture the moment of penetration through the bone and the extraction of the drill bit from the bone.
Experimental conditions
When executing bicortical drilling in “Cortex II” mode, the drill bit translational movement stops automatically after drilling of the second cortex. The maximal penetration of the drill bit out of the second cortex was within 1 mm (up to 1 mm): only the chisel edge (tip) outside of the second cortex could be seen. Therefore, the experiments were performed in the "Desired Depth" drilling mode, so the desired depth that must be achieved during the drilling was set 3–4 mm greater than the real depth of the hole in the bone in the drilling area. This way, significant penetration of the drill through the wall of the second cortex can be achieved before stopping the drilling and, respectively, register both the maximal temperature of the bone tissue and the drill bit during the drilling operation. The maximal feed rate during drilling was 4 mm/s [Citation28]. The experiments were performed under the conditions presented in .
Table 1. Experimental conditions.
The measurements were executed in a room where the bone temperature was on average 20 °C. The initial temperature of the drill bit and the bone is not an important factor, and during the drilling process it increased both in the bone and in the screw from 20 °C (room temperature) to body temperature (37 °C). That does not change the bone properties and does not influence the maximal temperature elevation [Citation30].
Results
Experiment 1
Bicortical drilling of porcine femur. New drill bit. Initial temperature T0 = 22.5 °C. The temperature was measured below the bone, in the area where the drill penetrates after drilling the second cortex ().
Figure 2. Temperature value immediately before drill bit penetration through porcine femur bone. Bx1, box 1 (Max 29.3 °C; Min 17.8 °C; Average 19.6 °C).
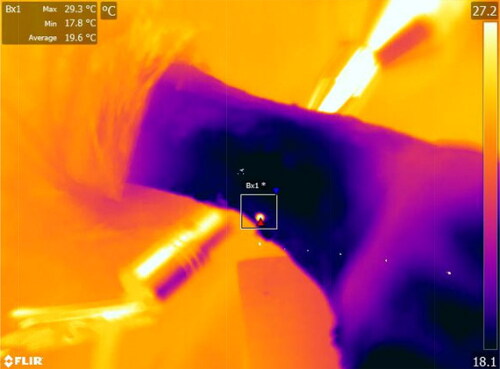
Before the drill bit penetration through the bone (), the temperature was 29.3 °C at 17:03.11 s (here and later the digits, which specify the moment of time in this record, mean 17th minute, 3rd second and 11 hundredths of a second). At the moment when the drill bit penetrated through the bone, the temperature had peaks from 45.9 °C at time 17:03.18 s to 66.8 °C. It must be noted that until this moment, the bone temperature was below the critical level of 50 °C. After removing the drill bit (), the bone temperature was 53.7 °C at 17:04.18 s (). At the end of the measurement, the temperature dropped to 26.6 °C at 17:10.87 s.
Figure 4. Temperature value immediately after removing the drill bit from the porcine femur bone. Bx1, box 1 (Max 53.7 °C; Min 18.2 °C; Average 23.5 °C).
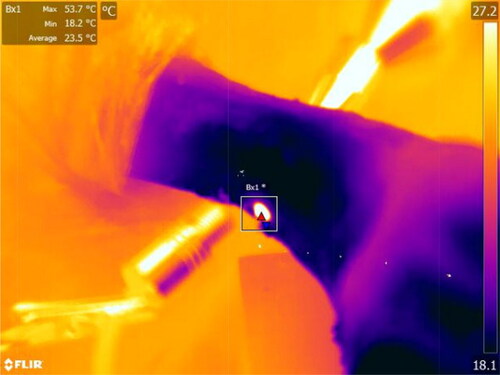
The sharp changes in temperature can be seen in the recorded graph for the drill bit. The change (deviation) in bone temperature showed a gradual increase, respectively, reduction. The maximal value of the temperature that the bone can reach during bicortical drilling corresponds to the moment of extraction of the drill from the second cortex after completion of drilling. Until then, the bone temperature increased constantly (heat absorption) due to the friction between the drill bit cutting edges and the bone, as well as due to the temperature gradient between them. Then it gradually decreased, and for a period of 6 s the temperature decreased from 53.7 to 26.6 °C at 17:10.87 s.
The maximal bone temperature was below 70 °C, and the time duration above the critical threshold of 50 °C was less than 0.4 s; at such conditions thermal osteonecrosis does not occur [Citation5].
Experiment 2
Bicortical drilling of porcine femur; 2.8-mm drill bit used 40 times. Initial temperature T0 = 21 °C.
Temperature measurement was performed in two zones. The first zone is the area of penetration of the drill bit inside the medullary canal after drilling of the first cortex. The second zone is in the area of penetration of the drill immediately after the drilling of the second cortex.
Experiment 2 captured two sides of the bone (). The camera started recording when the drill reached the inner wall of the first cortex (, Bx1). The peak of the graph in Box 1 is shown in . At the end of the experiment, the recorded temperature was 29.7 °C at 31:52.94 s. The peak of the graph for the second box (, Bx2) was at 31:43.96, with a maximal measured temperature of 69 °C on the drill after penetration through the bone (). When the drill was removed, the temperature was 57.1 °C at 31:45.04 s. At the end of the measurement, the temperature was 30.2 °C.
Figure 6. Maximal registered temperature during drilling below the second cortex of porcine femur. Bx1, box 1 (Max 32.7 °C; Min 19.2 °C; Average 23.9 °C). Bx2, box 2 (Max 69.0 °C; Min 19.6 °C; Average 31.3 °C).
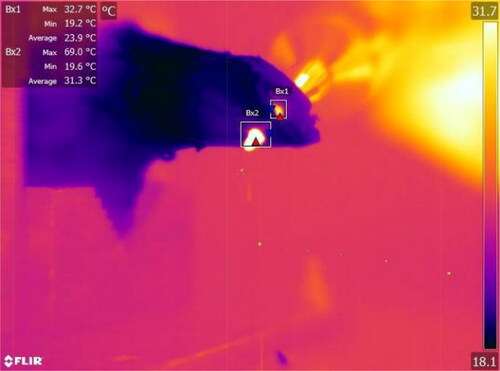
Figure 8. Graph of the temperature in Box 2 (see ). The temperature after removing the drill bit was 57.1 °C.
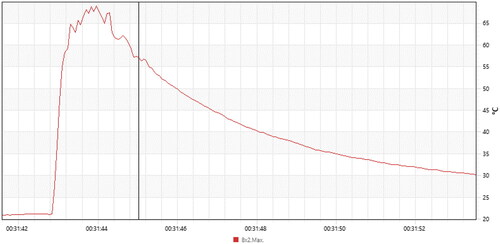
The maximal bone temperature was below 70 °C, and the time duration above the critical threshold of 50 °C was less than 2 s, in this case approximately 1 s, and thermal osteonecrosis did not occur.
Experiment 3
Bicortical drilling of bovine femur; 6-mm standard surgical two-flute drill bit, used 80 times. Initial temperature T0 = 21.5 °C.
The temperature was measured below the bone, in the area where the drill bit penetrates the far cortex, then the process was designed to stop automatically and the drill bit returned to its initial state (). A temperature graph during the experiment is shown in . The maximal recorded temperature was 103.2 °C on the drill bit, when the drill bit penetrated through the second cortex. When the drill bit was removed in 13:28:49.77 s, the measured temperature of the bone was 70.5 °C. From this time point on, the bone began to cool down, and at the end of the measurement, the temperature was 37.8 °C. As the maximal bone temperature was above 70 °C and the time duration above the critical threshold of 50 °C was more than 4 s, these were conditions for thermal osteonecrosis to occur.
Discussion
The aim of many experimental studies on the bone drilling process is to determine a combination of input parameters that will guarantee an optimal result in terms of the output parameters [Citation19, Citation31].
Up to now there are no in vivo studies with human bones, therefore the temperature of human bone tissue death is unknown [Citation32, Citation33]. Exposure of the bone to 50 °C for 2 s is accepted to be a critical level, as bone regeneration is almost entirely impossible when this level is exceeded [Citation1]. There are many reports about the influence of input parameters on the temperature increase during the drilling of bones. For instance, in SCOPUS there are over 3000 publications concerning the drilling of bones since 2000 [Citation19]. Different studies have reported a wide range of experimental results due to different test conditions concerning bone type, drill speed, feed rate and drill bit design. Experimental studies mainly focus on the influence of the input parameters of the process on its output parameters.
There appears to be a conflict between cutting conditions, such as feed rate and drill speed. A certain value of feed rate is optimal with respect to a certain parameter of the drilling process, but negatively affects another. A similar effect is observed with drill speed. The robot-assisted or mechatronic surgery system with feedback has significant advantages in bone drilling as compared to the conventional manual execution of the drilling process [Citation34] because this conflict between feed rate and drill speed can be solved by simultaneously controlling the values of these parameters in the conditions of robotic execution of this process.
Defining a combination of optimal values of the input parameters to ensure the optimal result of the drilling process is a task that is not so easy to be solved in practice.
That is for two reasons. First, a certain combination of input parameters may be optimal for a given output parameter but may also adversely affect another [Citation35]. Second, there are many input parameters, each of them with a range of possible values. The input parameters and the possible variations in their values are illustrated in .
Table 2. Input parameters, their values and possible variations.
As seen from , many of the input parameters are continuous variables with a wide range of possible values. By converting the continuous variables into categorical ones (), a simplified estimation of the possible combinations will give 128 combinations.
Table 3. Possible input parameter values.
Our experimental studies showed that during the automatic drilling of porcine bone the measured temperature remained below 70 °C, when the drill bit was new, the feed rate 4 mm/s and drill speed 1000 rpm. In such conditions, immediate osteonecrosis does not occur. The time duration at temperatures above 50 °C was less than 2 s. However, when the drill bit had been used before (40 or 80 times), the maximal bone temperature was above 70 °C during the drilling of the bovine bone, and the time duration above the critical threshold of 50 °C was more than 4 s. Such conditions lead to immediate osteonecrosis of the bone tissue in the drilling area.
The temperature increase during the bovine-bone drilling experiment could be attributed to some specifics of bovine bones and the drilling conditions: (i) the bone density of bovine bone is greater than that of human bone; (ii) the thickness of the cortical walls is significantly greater, that is, the depth of the drilled holes is greater; (iii) the drill used for the experiments was significantly worn out. These conditions were chosen quite deliberately. In surgical practice, there is no guarantee that only new or relatively new drills will always be used, that is, there is no guarantee that the temperature in the drilling zone will not exceed the critical threshold.
Conclusions
The experimental evaluation of the temperature during bicortical bone drilling by using a handheld robot ODRO showed that the temperature remained below 70 °C at a feed rate of 4 mm/s and drill speed of 1000 rpm when using a new drill bit. The duration of drilling over the critical threshold of 50 °C was less than 0.4 s, preventing thermal osteonecrosis. However, when using a worn-out drill bit, the temperature was above 70 °C, and the duration of drilling above the critical threshold of 50 °C exceeded 4 s, creating conditions for immediate osteonecrosis of the bone tissue in the drilling zone. The experiments demonstrate that the simultaneous control of drill bit speed and feed rate based on real-time data processing by relevant sensors can be achieved only by robotic execution of the manipulation because controlled feed rate and speed can be realized by automatic drilling. The robot ODRO allowed to avoid thermal osteonecrosis and to execute the drilling manipulation safely.
Authors’ contributions
Conceptualization, G.B. and R.K.; methodology, T.B. and N.S.; software, T.B.; validation, G.B., R.K. and K.D.; investigation, T.B., N.S. and G.B.; writing—original draft preparation, writing—review and editing, T.B., G.B. and K.D. All authors have read and agreed to the published version of the manuscript.
Disclosure statement
No competing interests to report.
Data availability statement
The data that support the findings reported in this study are available from the corresponding author upon reasonable request.
Additional information
Funding
References
- Timon C, Keady C. Thermal osteonecrosis caused by bone drilling in orthopedic surgery: a literature review. Cureus. 2019;11(7):e5226.
- Zhang Y, Xu L, Wang C, et al. Mechanical and thermal damage in cortical bone drilling in vivo. Proc Inst Mech Eng H. 2019;233(6):621–635.[Mismatch]
- Akhbar MFA, Sulong AW. Surgical drill bit design and thermomechanical damage in bone drilling: a review. Ann Biomed Eng. 2021;49(1):29–56.
- Feldmann A, Wandel J, Zysset P. Reducing temperature elevation of robotic bone drilling. Med Eng Phys. 2016;38(12):1495–1504.
- Shu L, Bai W, Shimada T, et al. Thermographic assessment of heat-induced cellular damage during orthopedic surgery. Med Eng Phys. 2020;83:100–105.
- Pazarci O, Torun Y, Ozturk A, et al. Comparative study of different drills for bone drilling: a systematic approach. Malays Orthop J. 2020;14(2):83–89.
- Augustin G, Davila S, Mihoci K, et al. Thermal osteonecrosis and bone drilling parameters revisited. Arch Orthop Trauma Surg. 2008;128(1):71–77.
- Chen Y, Hsiao C, Ciou J, et al. Effects of implant drilling parameters for pilot and twist drills on temperature rise in bone analog and alveolar bones. Med Eng Phys. 2016;38(11):1314–1321.
- Fernandes M, Fonseca E, Jorge R, et al. Effect of drill speed on the strain distribution during drilling of bovine and human bones. J Biomech Eng. 2018;2(5):69–74.
- Hou Y, Li C, Ma H, et al. An experimental research on bone drilling temperature in orthopaedic surgery. TOMSJ. 2015;9(1):178–188.
- Lughmani W, Bouazza-Marouf K, Ashcroft I. Drilling in cortical bone: a finite element model and experimental investigations. J Mech Behav Biomed Mater. 2015;42:32–42.
- Staroveski T, Brezak D, Udiljak T. Drill wear monitoring in cortical bone drilling. Med Eng Phys. 2015;37(6):560–566.
- Yen P, Ho T. Shared control for a handheld orthopedic surgical robot. IEEE Robot. Autom. Lett. 2021;6(4):8394–8400.
- Welcome BM, Gilmer BB, Lang SD, et al. Comparison of manual hand drill versus an electric dual-motor drill for bedside craniotomy. Interdiscip Neurosurg: Adv Tech Case Manag. 2021;23:100928.
- SMARTdrill 6.0 Now Available for Orthopedic Surgery, https://www.prnewswire.com/news-releases/smartdrill-6-0-now-available-for-orthopedic-surgery-300809777.html.
- IntelliSense Drill Technology, https://www.mcginleyorthopedicinnovations.com/.
- Louredo M, Diaz I, Gil J. DRIBON: a mechatronic bone drilling tool. Mechatronics. 2012;22(8):1060–1066.
- Gil J, Díaz I, Accini F. Inferring material properties in robotic bone drilling processes. Acta Bioeng Biomech. 2019;21(3):109–118.
- Jamil M, Rafique S, Khan AM, et al. Comprehensive analysis on orthopedic drilling: a state-of-the-art review. Proc Inst Mech Eng H. 2020;234(6):537–561.[Mismatch]
- Akhbar MFA, Yusoff AR. Drilling of bone: effect of drill bit geometries on thermal osteonecrosis risk regions. Proc Inst Mech Eng H. 2019;233(2):207–218.[Mismatch]
- Hou Y, Li C, Ma H, et al. A theoretical analysis on bone drilling temperature field of superhard drill. TOMEJ. 2016;10(1):109–125.
- You F, Li J, Cui H, et al. Study of the influence of models on the drilling temperature of bone measured by thermocouples. Adv Compos Lett. 2020;29:2633366X2092140–2633366X2092149.
- Ben-Zvi Y, Reznikov N, Shahar R, et al. 3D architecture of trabecular bone in the porcine mandible and femur: inter-trabecular angle distributions. Front Mater. 2017;4:29.
- Sarparast M, Ghoreishi M, Jahangirpoor T, et al. Modelling and optimization of temperature and force behaviour in high speed bone drilling. Biotechnol Biotechnol Equip. 2019;33(1):1616–1625.
- Sarparast M, Ghoreishi M, Jahangirpoor T, et al. Experimental and finite element investigation of high‑speed bone drilling: evaluation of force and temperature. J Braz Soc Mech Sci. 2020;42:349.
- Islam M, Kamarrudin N, Daud R, et al. Temperature measurement methods in an experimental setup during bone drilling: a brief review on the comparison of thermocouple and infrared thermography. J. Phys.: Conf. Ser. 2021;2129(1):012096. ICMProTech 2021
- Boiadjiev T, Boiadjiev G, Delchev K, et al. Far cortex automatic detection aimed for partial or full bone drilling by a robot system in orthopaedic surgery. Biotechnol Biotechnol Equip. 2017;31(1):200–205.
- Boiadjiev T, Boiadjiev G, Delchev K, et al. Feed rate control in robotic bone drilling process. Proc Inst Mech Eng H. 2021;235(3):273–280.[Mismatch]
- Feldmann A, Zysset P. Experimental determination of the emissivity of bone. Med Eng Phys. 2016;38(10):1136–1138.
- Augustin G, Davila S, Udiljak T, et al. Determination of spatial distribution of increase in bone temperature during drilling by infrared thermography: preliminary report. Arch Orthop Trauma Surg. 2009;129(5):703–709.
- Shakouri E, Sadeghi MH, Maerefat M, et al. Experimental and analytical investigation of the thermal necrosis in high-speed drilling of bone. Proc Inst Mech Eng H. 2014;228(4):330–341.[Mismatch]
- Niehues SM, Elezkurtaj S, Bresssem KK, et al. Evaluation of potential tissue heating during percutaneous drill-assisted bone sampling in an in vivo porcine study. Skeletal Radiol. 2022;51(4):829–836.[Mismatch]
- Augustin G, Zigman T, Davila S, et al. Cortical bone drilling and thermal osteonecrosis. Clin Biomech (Bristol, Avon). 2012;27(4):313–325.
- Wang W, Shi Y, Yang N, et al. Experimental analysis of drilling process in cortical bone. Med Eng Phys. 2014;36(2):261–266.
- Boiadjiev T, Boiadjiev G, Delchev K, et al. Orthopedic bone drilling robot ODRO: basic characteristics and areas of applications. In Latest developments in medical robotics systems. IntechOpen. 2021, p. 1–21.