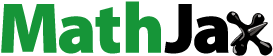
Abstract
Nanodiamonds (ND) are nano-particles with a size of 4–5 nm which are intensively investigated for a number of properties that make them suitable for bio-applications. They could be chemically modified, they have a high adsorption capacity, high mechanical and chemical stability. Because ND have all these properties, they are also suitable for application in specific wastewater treatment and especially when the treated water is polluted with toxic compounds. The effect of ND applied in laboratory sand biofilter was investigated in this research. Synthetic wastewater with a model toxicant, the azo-dye amaranth, was used. The results showed that, in the presence of ND, the efficiency of amaranth removal remained high (more than 90%) in concentrations close to the critical one. The level of carbon-containing pollutants (assessed as chemical oxygen demand (COD) and total organic carbon (TOC)) decreased. The number of bacteria from the key detoxification groups increased 98 times for Pseudomonas sp. and 105 times for azo-degrading bacteria, while aerobic heterotrophs increased only 6 times after ND were applied. The abundance of Pseudomonas spp. after ND addition was confirmed with fluorescence in-situ hybridization.The activities of the key enzymes increased with 26–57%. Also, the activities of cathechol-1,2-dioxygenase and catechol-2,3-dioxygenase were found after ND addition but not before that. The obtained data showed that after ND were introduced in the wastewater treatment system, the number of biodegrading bacteria increased. They synthesized large amounts of azoreductases and oxygenases that led to the improved parameters of azo-detoxification process.
Introduction
The treatment of industrial wastewater polluted with recalcitrant xenobiotics is a challenging task. Thus, industries always need innovations leading to improved efficiency and stabile parameters of the water purification process. Nanotechnologies offer a new range of materials with promising characteristics. Nanodiamonds (ND) are a good example of this. They are nano-sized particles with high adsorbtion capacity and low reactivity, unless modified [Citation1,Citation2]. Among their advantages are also affordability, biocompatibility, low toxicity [Citation3–5] and a wide range of modifications [Citation6–12].
ND have complex structure. They possess a hard core of carbon atoms in the form of diamond lattice and shell consisted of carbon atoms arranged as graphite layered structure [Citation13]. The average size of the nanodiamond particles is 4–5 nm, which enables them to exist in the form of colloidal suspensions [Citation14]. There are several ways for their synthesis: detonation [Citation7,Citation15–17] high-energy ball milling of high pressure [Citation18], laser ablation [Citation19,Citation20], plasma-assisted chemical vapor deposition [Citation21,Citation22], ion irradiation of graphite [Citation21,Citation23] and ultrasound cavitation [Citation24,Citation25].
Detonation ND are already commercialized. This brings forward their applications toward the real practice in different fields (medical [Citation3,Citation26–32], biological [Citation4,Citation5,Citation8,Citation33–40], energy [Citation41–44] and environmental applications [Citation45–47]).
The mentioned investigations and characteristics of ND demonstrate potential for application of ND not only in the bio-medical field but also in other areas in the biology sciences [Citation48,Citation49]. The environmental pollution with toxic xenobiotic compounds poses many critical problems. The main one maybe is the low efficiency of their biodegradation. A very good example of this is the widely used azo-dyes [Citation50,Citation51].
Since it is not possible to bind the entire amount of the dyes applied to tissues, a significant proportion of the colorants go into the wastewater (between 2% and 50%) [Citation52]. The textile industry, due to the specifics of dyeing technology, ranks among the most water-consuming industries [Citation53]. The significant wastewater quantity produced in the coloring process represents a serious environmental risk due to the carcinogenic and mutagenic effects of the synthetic dyes [Citation54–56]. The biodegradation of the recalcitrant azo-dyes could be accelerated using different augmentation strategies. The new nanomaterials like ND have great potential because of their unique properties and low price. That is why the present research was focused on the effects of ND on a lab-scale azo-dye biodegradation process and their possible application as abioaugmenting factor.
Materials and methods
Nanodiamonds
The effect of ND was studied by using detonation ND produced by prof. Stavri Stavrev (ATM Istanbul, Turkey). Briefly, 40 mL of 2.5% ND suspension in synthetic wastewater was applied on the top of the biofilter. It was retained in it for 60 min. After that the unadsorbed ND were washed away with synthetic wastewater.
Biofilter
A laboratory model of submerged downflow biological filter was used for carrying out the azo-detoxification process (). The inert carrier, which supports the formation of the active biofilm, was quartz sand (the size of the sand particles was 0.08–0.16 сm). It was kindly provided by Bistritza Drinking Water Plant (Sofiyska voda, Veolia Group).
The volume of the biofilter was 190 cm3 and its height was 3 cm. In the microbiological, enzymological and FISH analysis, it was divided into three sand layers: upper layer (0–1 cm), middle layer (1–2 cm) and bottom layer (2–3 cm).
Xenobiotic compound
The model xenobiotic used in this study was the azo-dye amaranth (C.I. No. 16185), supplied by Fluka Analytical. This azo-dye was chosen because it is often applied in the textile and food industries. It is very suitable for fast and rough visual estimation of the xenobiotic removal because of its discoloration during the biodegradation process. It was also very important that the biochemical steps of amaranth biodegradation are known, and therefore their activity could be studied during the process ()
Figure 2. Main biochemical steps in amaranth biodegradation (AzoR – azoreductase; C12DO – catechol-1,2-dioxygenase, C23DO – catechol-2,3-dioxygenase). The scheme illustrates the first stage of decolorization (catalyzed by AzoR) and the second stage in which the detoxification is performed. The key biochemical step in it is the decyclization of the intermediate metabolites by the ortho-cleavage (catalyzed by C12DO) or meta-cleavage (catalyzed by C23DO).
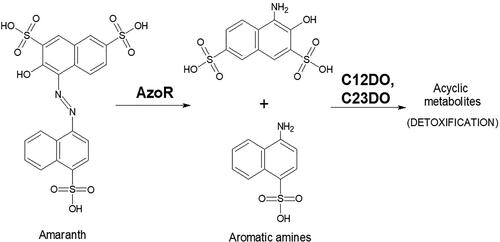
Synthetic wastewater
In the described experiments, synthetic wastewater was used. It was composed of synthetic saline solution [Citation57] with the addition of yeast extact 5 g/L [Citation58] and definite amount of amaranth (from 10 mg/L up to 55 mg/L).
Biological system
Activated sludge was used as inoculation material for biofilm formation. It was kindly provided by Sofiyska Voda AD (Veolia Group, Bulgaria) from the wastewater treatment plant of Sofia city. Before the immobilization the flocks of the activated sludge were disintegrated via ultrasound sonication for 3 × 10 s with a frequency of 22 kHz (UD-20 automatic, Techpan, Warszawa). The immobilization of the cell to the sand particles was based on adsorption. The microbial suspension was incubated for 2 h in the biofilter sand layer. The biofilm performing the azo-detoxification process was formed on the sand particles surface in the model biofilter. This type of attached microbial growth permits the formation of substrate, oxygen and redox gradients. It ensures the simultaneous activity of the anaerobic and aerobic stages of the amaranth biodegradation.
Nanodiamonds (ND)
In this study, detonation ND particles with a size of 2–10 nm were used in 2.5% water suspension. The ND were produced by detonation of TNT and hexogen and were provided by prof. Stavrev, managing director of ATM Istanbul, Turkey. They were applied as 10% of the volume of the biofilter.
Water treatment detoxification process
The model sand biofilter functioned in a semi-continuous regime for 623 h. The flow rate was 550 mL/day. The COD (chemical oxygen demand) of the influent was 590 mgO2/L and the TOC (total organic carbon) of the influent was 240 mg/L. In the first 24 h, the model water passing through the sand layer contained only synthetic saline and nutrient solutions in the described concentrations. This ensured fast biofilm growth in the initial stage of the experiment and stability of the system in the next stages.
From the second day up to the end of the experiment, certain amount of amaranth was included in the synthetic wastewater (from 10 mg/L up to 55 mg/L). The amaranth concentration was gradually raised ensuring stepwise adaptation of the biofilm toward amaranth degradation. The flow rate, COD and TOC of the influent, the residual amaranth concentration, efficiency and rate of its removal and the influent turbidity were monitored 2–3 times per day.
Samples from the biofilm community were taken four times during the azo-degradation process: at the beginning (0 h), in the early stage of functioning (191 h) before ND addition, in the late stage of functioning (455 h) after ND addition and at the end of the experiment (623 h).
ND were applied in the early stage (269 h) when signs of destabilization of the water treatment system were detected.
Alongside the described biofilter, another control sand biofilter without ND performed the same azo-degradation process.
Methods and analysis
In this experiment, the influence of the ND on a biological azo-detoxification process was investigated with four different types of analysis.
Technological analysis
COD was determined according to the APHA method [Citation59]. TOC was monitored using a TOC-VCPH/CPN analyzer (Shimadzu, Japan). The residual amaranth concentration was sectrophotometrically determined at 520 nm (Ultrospec 3000, Pharmacia Biotech Ltd.) [Citation60]. The effluent turbidity at 450 nm was measured as an indicator for the biomass washed away from biofilter. The efficiency of amaranth removal was calculated according to the following equation [Citation58]:
(1)
(1)
where Cin is the concentration of amaranth in the influent in mg/L, Ceff is the concentration of amaranth in the effluent in mg/L.
The rate of amaranth removal was calculated according to equation (2):
(2)
(2)
where Cin is the concentration of amaranth in the influent in mg/mL, Ceff is the concentration of amaranth in the effluent in mg/mL, Q is the flow in mL/h.
Microbiological analysis
The plate count technique was used for the examination of the number of microorganisms from the key groups for detoxification [Citation61]. Aerobic heterotrophs were cultured on Nutrient agar (Himedia Laboratories, India). For anaerobic heterotrophs, the same culture media was used, and the Petri dishes were incubated in Anaerostat (Merck, Germany) with Anaerocult (Merck, Germany). The microorganisms from genus Pseudomonas were examined using Glutamate Starch Pseudomonas Agar (Himedia Laboratories, India). The number of the azo-degrading bacteria was determined by using Nutrient agar (Himedia Laboratories, India) with 50 mg/L amaranth. Only the colonies with a discolored zone around them were counted.
Enzymological analysis
The activities of several key enzymes from the amaranth biodegradation pathway were investigated. The azoreductase activity was determined by the method described by Grekova-Vasileva [Citation60]. This enzyme is responsible for the reduction of the azo-bond and causes discoloration of the dye. The next critical step in the detoxification of amaranth is the benzene rings opening by catechol-1,2-dioxygenase and catechol-2,3-dioxygenase. Their activities were investigated by the methods of Willets and Cain [Citation62] and Farr and Cain [Citation63]. The total metabolic activity in the biological system was estimated by the succinate dehydrogenase activity by the method of Veeger [Citation64]. For all the samples, the specific enzyme activities were calculated on the basis of the protein content according to the Lowry method [Citation65].
Fluorescence in-situ hybridization (FISH)
Two types of fluorescence analysis were performed on the biofilm samples taken from the sand biofilter: DAPI staining for visualization of the whole biofilm particles, and FISH for investigation of the quantity and the spatial distribution of the microorganisms from genus Pseudomonas. DAPI (4′,6-diamidine-2′-phenylindole) was applied in a concentration of 1 µg/mL. FISH was performed with 5′-Cy5 stained oligonucleotide probe specific for the genus Pseudomonas (5′- GCT GGC CTA GCC TTC -3′) [Citation66]. The probes specificity was confirmed by testing with a non-sense probe with a sequence of 5′- ACT CCT ACG GGA GGC AGC -3′ [Citation60]. The hybridization was performed according to Nielsen [Citation67]. The fluorescence pictures were taken with an epifluorescence microscope equipped with a digital camera (Leica Micro Systems DFC 310 FX).
Statistical analysis
The data from the chemical analyses are the arithmetic mean of three independent replicates, and from the microbiological ones, of 3 or 4 replicates. The results were processed according to Student and Fisher [Citation68]. Standard deviations were calculated with a 95% confidence level.
Results
To study the effect of ND on the water detoxification process, the purification biological system was studied on four levels: 1/ purification process characteristics were monitored and compared with control biological system; 2/ the biodegrading microorganisms were studied; 3/ their activity was estimated by the activity of the key enzymes; 4/ the group of the key unculturable bacteria was assessed by FISH.
The influent amaranth concentration and the efficiency of its removal are shown in . From the beginning of the experiment up to 218 h, the concentration of the xenobiotic was gradually increased from 10 mg/L up to 40 mg/L. At a concentration of 40 mg/L, destabilization of the system occurred. This indicated that the critical xenobiotic concentration was almost reached. The optical density at 450 nm increased from 0.088 up to 0.116 for the biofilter with ND and from 0.116 up to 0.177 for the control biofilter showing an increase in the bacterial removal from the biofilter. The discussed effect is a typical reaction toward toxic stress. In this way, the biofilm lost part of its biodegrading capacity. This was confirmed by the optical density of the effluent in the subsequent period (245–411 h). It was more than two times lower for the two biofilters (2.4 times for the biofilter with ND and 2.2 times for the control biofilter).
Figure 3. Efficiency of amaranth removal during the azo-degradation process. The ND (ND) application at 269 h is shown in red.
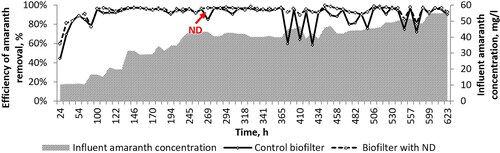
As a consequence of the biofilm destabilization, the efficiency in the control biofilter dropped from 96.03% to 84.73% at 269 h. At this moment, ND were applied in the other biofilter. In that biofilter, there was no reduction in the efficiency: from 96.30% it slightly increased to 97.33% and remained over 97% up to 315 h ().
The presence of the ND in the amaranth-treating biofilter had beneficial influence over a longer period than expected: the efficiency of the xenobiotic removal remained high up to 555 h. This suggested that the stimulating effect of the ND involves more complex mechanisms than just immobilization of active molecules through adsorption.
The presence of the ND also had a stimulating effect on COD and TOC. The COD of the effluent decreased with 23.56% for the period of 18 days after ND addition (from 442.77 mgO2/L to 338.64 mgO2/L) and TOC decreased with 8.43% (from 100.68 mg/L to 92.19 mg/L). The overall reduction of COD of the effluent was 31.61% and for TOC it was 55.94%.
The microbiological investigations showed that after ND were applied in the sand biofilter, the number of the microorganisms in the upper part of the biofilter increased. This effect was observed for all bacterial groups which are involved in amaranth biodegradation (aerobic heterotrophs with 6-fold increase, anaerobic heterotrophs with 15-fold increase, bacteria from g. Pseudomonas with two orders of magnitude, azo-degraders also with two orders of magnitude – ). The microbiological data suggested that the biofilm had grown in the biofilter, which was confirmed by SEM analyses (). It also indicates that the microbial community was specialized for azo-degradation: the increase in bacterial colony forming units was highest in the groups of azo-degraders and bacteria from genus Pseudomonas.
Figure 4. Key microbial groups in the biofilter before (191 h) and after (455) ND addition (AeH – aerobic heterotrophs; AnH – anaerobic heterotrophs; Pseud. sp. – Pseudomonas sp.; AzoDegr – azo-degrading bacteria).
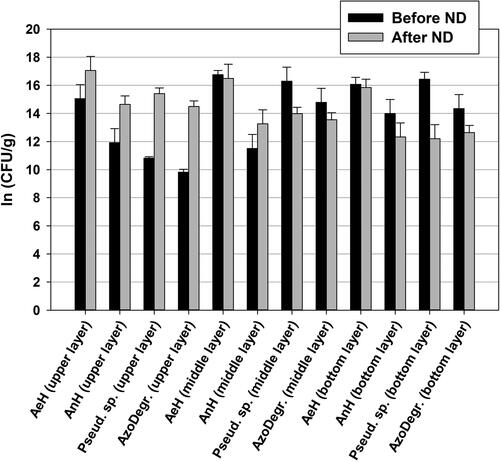
Figure 5. SEM image of the sand biofilter at 455 h: biofilm (a); quarz sand particle (b). Scanning electron microscope JSM-5510 (JEOL, Japan).
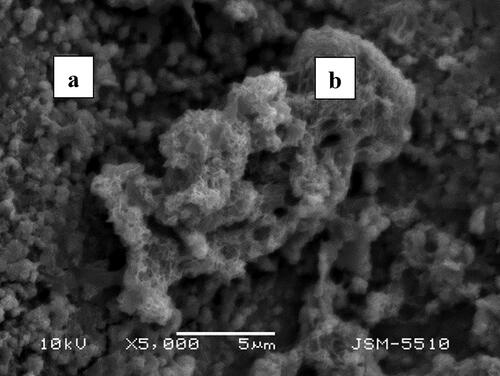
The augmentation effect of the ND on the key microbial groups was concentrated in the upper part of the biofilter probably because of the depletion of nutrients in the depth of the sand layer.
The biofilm was thick and well formed in the bioreactor where ND were applied (). The higher stability of the process in that biofilter and the higher efficiency of amaranth removal, discussed above, were probably related to the retainment of the biomass despite the raised concentrations of the xenobiotic. This was confirmed by fluorescence in-situ hybridization. The molecular technique showed an increase in the bacteria of the genus Pseudomonas, which probably contributes to a fast increase in the rate of amaranth degradation.
The enzyme analysis showed higher activity of the azoreductase (56.48%) and of the succinate dehydrogenase (25.81%) in the upper part of the biofilter after ND addition. So, after the addition of ND, not only the bacteria counts were more but also their activity was higher.
Before ND addition, the activities of the two catechol oxygenases were non-detectable (). These enzymes perform a key detoxification step – the benzene ring cleavage. After ND were added, catechol-1,2-dioxygenase and catechol-2,3-dioxygenase activities were found in the community. They metabolized 1.4 − 2.7 µmols of their substrate per minute per mg protein and their activities were almost uniformly distributed in the depth of the biofilter. This is probably due to the fact that these enzymes use aromatic amines generated in the azo-reduction. Therefore, they have to be first produced with the aid of the azoreductase and after that, metabolized by the dioxygenases.
Figure 6. Activities of the key enzymes from the biodegradation pathway of amaranth before (121 h) and after ND addition (455 h): azoreductase activity (a); succinate dehydrogenase activity (b); catechol-1,2-dioxygenase activity (c); catechol-2,3-dioxygenase activity (d).
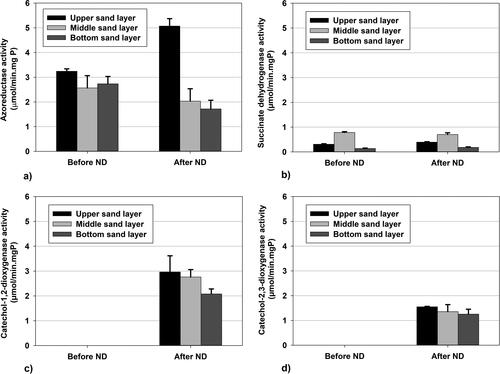
The catechol-1,2-dioxygenase showed higher activity than the catechol-2,3-dioxygenase with 80%. The ortho-pathway of benzene ring cleavage predominates in most biodegradation systems, which explains the obtained results.
The data from the enzyme assays showed that the presence of ND in the biofilter activates the first step of the biodegradation of amaranth – the azo-reduction. It also contributes to the onset of the second biochemical stage – the removal of the cyclic intermediate metabolites – aromatic amines.
The augmentation effect of the nanоdiamonds on the microbial azo-degrading community was confirmed by fluorescence in-situ hybridization. The results showed significant fluorescence after ND application (). This demonstrates that the bacteria from the key biodegrading genus Pseudomonas increased their numbers in the biofilm in the time period following ND addition.
Figure 7. Fluorescence images of biofilm samples with DAPI and FISH taken before ND addition, at 191 h (a,c); and after ND application, at 455h (b,d).
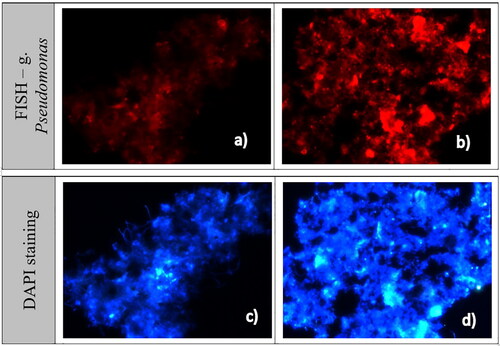
The registered effects of ND were probably related to the high adsorption capacity of the nanoparticles. By adsorption they immobilize enzymes and co-factors, which improves the biodegradation of the pollutants. Such high affinity of the ND toward protein adsorption was described by Wehling et al. [Citation39]. This effect of the ND probably lowers the toxicity and assists in the formation of a stabile microbial community.
Discussion
The study of biofilms is of great importance for their applications in various areas of biotechnology. In some cases, obtaining stable biofilms with regulated, increased activity compared to planktonic microorganisms is pursued. Such are the cases with the application of biofilms constructed by specialized microorganisms and microbial communities, which participate and accelerate the purification detoxification processes [Citation67,Citation69,Citation70].
In our research, we sought to find possibilities for the stabilization of biofilm communities at the structural level and for increasing their activity based on the induction and manifestation of the activity of diazoreductase and oxygenase enzymes – catechol-1,2-dioxygenase and catechol-2,3-dioxygenase. This is accomplished by the addition of positive biofilm modulators such as ND. They ensure the stability of biofilm functioning in high detoxification activity against the toxic pollutant amaranth.
In the research practice, there is another trend – to search for mechanisms and modulators that prevent the formation, stabilization and regeneration of biofilms. These antibiofilm approaches are applicable in the inhibition of biofilms of pathogenic microorganisms. In recent years, these approaches have been far more researched than the approaches for stabilization and positive modulation of biofilms. Control with antibiofilm regulators is a rapidly developing trend in therapeutic clinical practice [Citation71,Citation72].
Our research concerns the stabilization of biofilms, which are an important factor in water purification, and here the goal is to conduct control that ensures the stability of sustainable functioning, increasing and expanding the biodegradation detoxification potential of biofilm communities without introducing new pollutants into the system. Such are the non-toxic ND. They themselves are used to redistribute the concentrations of biologically active molecules in therapeutic practice, as well as a transport factor of such molecules to target sites [Citation49,Citation73].
The application of ND as a positive modulator of sustainable biofilm renewal with highly specialized microbial consortia aimed at complete biodegradation of amaranth diazo dye. This approach provides an increase in the detoxification potential of purification modules of the type of biofilters with sand particles or other carriers. In all cases of functioning of biofilters, there are repeated moments in which the biofilm is destabilized due to renewal. At this point, the speed and efficiency of the purification process is reduced. This is clearly seen from the data in . The amaranth elimination efficiency becomes unstable and decreases significantly in the destabilization phases after 240 h and after 350 h.
Finding the appropriate algorithm to overcome these critical moments in the functioning of biofilters and biofilms is of key technological importance. In this case, the following elements of the search algorithm are important: to find the appropriate modulator, to specify its concentration, the appropriate moment of addition to the system. It is no less important to clarify the mechanism of the stimulating action of this positive modulator. In some of the water purification modules, as such a modulator, specialized microbial preparations with regulated enzyme activity against the target pollutants, enzymes, or other more complex biological additives are used. They are rather more expensive [Citation74,Citation75].
In our results, the addition of ND at 245 h of biofilm operation clearly delineates two positive trends affecting water purification and biodegradation of diazo dyes in laboratory biofilters operating in semi-continuous mode. In a situation of prolonged functioning of the biofilm in the stages of renewal and destabilization, the addition of ND improves the technological characteristics of the purification process: the stability of the efficiency of the elimination of diazo dyes is preserved [Citation76]. In the long-term functioning of the biofilm, the addition of an external factor (ND) plays the role of a binding factor of the released (planktonic) bacteria and preserves the synergistic relations of the consortia, thus surviving in the separated fragments [Citation77,Citation78]. It is possible because ND possess very strong absorption and binding activity and a very high surface area for adsorption. Moreover, some active enzymes are also absorbed in these newly formed consortia, as well as some subcellular elements with embedded enzymes. Active enzymes and highly active bacteria ensure a high detoxification activity of the biofilm in the destabilization phase. This also deeply affects the mechanisms of detoxification processes involved in the general purification process and the purification process in general [Citation70,Citation73,Citation79–81].
At the level of the mechanism of functioning of the microbial consortia in the biofilm, the following occurs: the disintegration of the biofilm is associated with the dispersal of synergistic communities of microbial fragments. This is clearly seen in the FISH analysis (). Nevertheless, free-swimming azo-degrading bacteria in the liquid biofilm phase are assumed to survive and function homogeneously. They are not as effective in their biodetoxification potential, as they have to carry out all the biodegradation processes individually. This will go on and on until new synergistic structures are formed [Citation82].
Figure 8. Images of disintegrated biofilm from the initial stage of the experiment (191 h): DAPI (a); FISH for genus Pseudomonas (b); light microscopy (c). Images of disintegrated biofilm from the final stage of the experiment (623 h): DAPI (d); FISH for genus Pseudomonas (e); light microscopy (f).
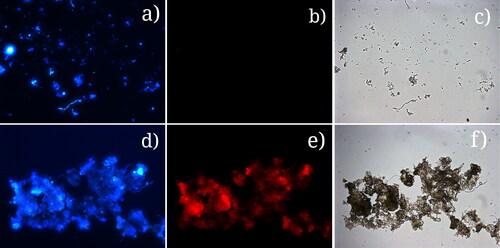
A critical moment for the functioning of the biofilm is the increase in the concentration of amaranth as a toxic compound in the water phase due to the impaired purification activity. By introducing an external factor, such as ND, at the time of increasing toxicity, we register the following effects: 1/ The absorption of homogeneous cells increases and the synergistic relationship in the consortium of active bacteria, active enzymes and active subcellular structures with included enzymes is preserved; 2/ In this formed upper layer of the biofilm, the NDs added at the right moment adsorb the amaranth as a toxic factor, i.e. the high detoxification activity is also stimulated by the higher concentration of the toxic substrate. This toxic press forces highly plastic pseudomonads to unlock new additional detoxification pathways and mechanisms.
The approach ensures a reduction of its toxic effect on amaranth for the more sensitive homogenous cells. At the same time, the concentration of amaranth increases around the ND structures and Pseudomonas cells with a high potential for biodegradation are adsorbed there – highly efficient clusters are formed (). This is clearly seen in the FISH images ().
Figure 9. Mechanism of formation of high-performance clusters of nanodiamonds, bacteria and amaranth in the biofilm, after the addition of nanodiamonds to the system.
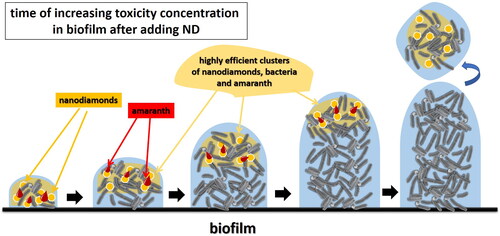
Another positive effect of all the processes described above is that the clusters formed around the ND are more quickly adsorbed on the sand particles. They remain stable and with increased detoxification activity. In this way, sustainable renewal of the biofilm and sustainable functioning of the biofilter is ensured. The described mechanisms and processes are illustrated in and are reflected in the steady-state elimination efficiency of amaranth after ND supplementation, illustrated in .
The high preserved degree of synergism of the diagnosed microbial consortia in the biofilm and in the preserved biofilm fragments leads to an augmentation at the enzymological level. The increase in the increase in azo-reductase activity in the upper layer of the biofilm is clearly illustrated. The high azo-reductase activity ensures quickly and in higher concentrations aromatic metabolic products from the accelerated initial degradation of amaranth. The increased concentrations of the aromatic products, the increased amount of plastic pseudomonads and the high degree of synergism between them lead to the induction and manifestation of important enzymes that cleave the benzene ring in all three investigated layers of the biofilm – significant activities of catechol1,2- and the catechol-2,3-dioxygenase. Enzyme activities are highest in the upper, but are at a sufficiently high level in the middle and lower layers of the biofilm, as illustrated in , c, d).
The results confirm that ND as an augmenting factor expand the metabolic potential of biofilm organisms. They retain and increase the degree of synergism and lead to the complete detoxification of amaranth in both phases: 1) reduction of the diazo bond and generation of aromatic amines and 2) cleavage (decyclization) of the benzene ring of the aromatic amines and complete detoxification of the toxic amaranth dye. ND as a positive modulator of the purifying detoxification activity of the biofilm act at the following levels – structurally, at the level of relationships between the key biodegradants, they strengthen the synergism without increasing the number of pseudomonads ( and Citation7), at the functional level, regulate the concentration of the toxic substance and its metabolites by ensuring their higher concentration in the stable clusters, at the enzymological level – they unlock the activity of enzymes that are not registered without the addition of ND, i.e. provide elimination of amaranth and its toxic aromatic metabolites.
The proven effects of the applied approach of adding ND as an augmenting factor show that the control of the sustainable renewal and functioning of biofilms is an important mechanism for the management of purification processes with an included detoxification element. The investigated water treatment module can be scaled up and applied as pre-treatment or post-treatment of water treatment technologies in the dye industry.
Conclusions
This study demonstrated demonstrates that ND play the role of an external stimulating, bioenhancing factor of amaranth biodegradation in a biofilm reactor: 1) The dye is adsorbed during increasing concentration and toxicity; 2) They accelerate and maintain the clustering of the active pseudomonads around the ND. 3) They help to increase the azo-reduction and unlock the complete detoxification of the amaranth metabolic products after breaking the diazo bond by inducing the manifestation of catechol 1,2- and catechol 2,3-dioxygenase activities in all three layers of the studied biofilm. 4) They play the role of a positive modulator of the sustainable renewal and functioning of the biofilm, ensuring the detoxification of amaranth. All this increases the stability of the diazo elimination efficiency and of the purification process as a whole. The proposed mechanism for the control of biofilm communities asserts that the fine-tuning, control and regulation of processes in the biofilm through augmenting factors opens up new opportunities for technological management based on the deepest known mechanisms of biodegradation processes in real biotechnological systems and ecobiotechnologies.
Author contributions
YIT conceptualized and designed the study; MVB and IDY performed the experiments; YIT, MVB and IDY analyzed and interpreted the data, wrote, revised and approved the final version of the manuscript.
Data availability statement
The data that support the findings of this study are available from the corresponding author, Ivaylo Yotinov, upon reasonable request.
Disclosure statement
No potential conflict of interest was reported by the authors.
Additional information
Funding
References
- Ho DN. Applications in biology and nanoscale medicine. Springer US. 2010;10:978–971.
- Turcheniuk K, Mochalin VN. Biomedical applications of nanodiamond. Nanotechnology. 2017;28(25):252001.
- Liu KK, Cheng CL, Chang CC, et al. Biocompatible and detectable carboxylated nanodiamond on human cell. Nanotechnology. 2007;18(32):325102.
- Moore L, Grobárová V, Shen H, et al. Comprehensive interrogation of the cellular response to fluorescent, detonation and functionalized nanodiamonds. Nanoscale. 2014;6(20):11712–11721.
- Yu SJ, Kang MW, Chang HC, et al. Bright fluorescent nanodiamonds: no photobleaching and low cytotoxicity. J Am Chem Soc. 2005;127(50):17604–17605.
- Arnault JC, Girard HA. Hydrogenated nanodiamonds: synthesis and surface properties. Curr Opin Solid State Mater Sci. 2017;21(1):10–16.
- Betz P, Krueger A. Surface modification of nanodiamond under Bingel–Hirsch conditions. ChemPhysChem. 2012;13(10):2578–2584.
- Fessele C, Wachtler S, Chandrasekaran V, et al. Thiourea-bridged nanodiamond glycoconjugates as inhibitors of bacterial adhesion. Eur J Org Chem. 2015;2015(25):5519–5525.
- Jarre G, Heyer S, Memmel E, et al. Synthesis of nanodiamond derivatives carrying amino functions and quantification by a modified Kaiser test. Beilstein J Org Chem. 2014;10(1):2729–2737.
- Jarre G, Liang Y, Betz P, et al. Playing the surface game—Diels–Alder reactions on diamond nanoparticles. Chem Commun. 2011;47(1):544–546.
- Liang Y, Meinhardt T, Jarre G, et al. Deagglomeration and surface modification of thermally annealed nanoscale diamond. J Colloid Interface Sci. 2011;354(1):23–30.
- Petit T, Arnault JC, Girard HA, et al. Oxygen hole doping of nanodiamond. Nanoscale. 2012;4(21):6792–6799.
- Shenderova OA, Zhirnov VV, Brenner DW. Carbon nanostructures. Crit Rev Solid State Mater Sci. 2002;27(3-4):227–356.
- Aleksenskii AE, Baidakova MV, Vul AY, et al. The structure of diamond nanoclusters. Phys Solid State. 1999;41(4):668–671.
- Kovalenko I, Bucknall DG, Yushin G. Detonation nanodiamond and onion-like-carbon-embedded polyaniline for supercapacitors. Adv Funct Mater. 2010;20(22):3979–3986.
- Krueger A, Stegk J, Liang Y, et al. Biotinylated nanodiamond: simple and efficient functionalization of detonation diamond. Langmuir. 2008;24(8):4200–4204.
- Yeap WS, Tan YY, Loh KP. Using detonation nanodiamond for the specific capture of glycoproteins. Anal Chem. 2008;80(12):4659–4665.
- Boudou JP, Curmi PA, Jelezko F, et al. High yield fabrication of fluorescent nanodiamonds. Nanotechnology. 2009;20(23):235602.
- Amans D, Chenus AC, Ledoux G, et al. Nanodiamond synthesis by pulsed laser ablation in liquids. Diamond Relat Mater. 2009;18(2-3):177–180.
- Wang CX, Yang YH, Yang GW. Thermodynamical predictions of nanodiamonds synthesized by pulsed-laser ablation in liquid. J. Appl. Phys. 2005;97(6):066104.
- Gottlieb S, Wöhrl N, Schulz S, et al. Simultaneous synthesis of nanodiamonds and graphene via plasma enhanced chemical vapor deposition (MW PE-CVD) on copper. Springerplus. 2016;5(1):1–16.
- Yamada T, Ishihara M, Kim J, et al. A roll-to-roll microwave plasma chemical vapor deposition process for the production of 294 mm width graphene films at low temperature. Carbon. 2012;50(7):2615–2619.
- Dunlop A, Jaskierowicz G, Ossi PM, et al. Transformation of graphite into nanodiamond following extreme electronic excitations. Phys Rev B. 2007;76(15):155403.
- Al-Tamimi BH, Jabbar II, Al-Tamimi HM. Synthesis and characterization of nanocrystalline diamond from graphite flakes via a cavitation-promoted process. Heliyon. 2019;5(5):e01682.
- Khachatryan AK, Aloyan SG, May PW, et al. Graphite-to-diamond transformation induced by ultrasound cavitation. Diamond Relat Mater. 2008;17(6):931–936.
- Iyer JK, Dickey A, Rouhani P, et al. Nanodiamonds facilitate killing of intracellular uropathogenic E. coli in an in vitro model of urinary tract infection pathogenesis. PLoS ONE. 2018;13(1):e0191020.
- Lee DK, Kee T, Liang Z, et al. Clinical validation of a nanodiamond-embedded thermoplastic biomaterial. Proc Natl Acad Sci USA. 2017;114(45):E9445–E9454.
- Liu KK, Zheng WW, Wang CC, et al. Covalent linkage of nanodiamond-paclitaxel for drug delivery and cancer therapy. Nanotechnology. 2010;21(31):315106.
- Mogilnaya OA, Puzyr AP, Baron AV, et al. Hematological parameters and the state of liver cells of rats after oral administration of aflatoxin B1 alone and together with nanodiamonds. Nanoscale Res Lett. 2010;5(5):908–912.
- Wang X, Low XC, Hou W, et al. Epirubicin-adsorbed nanodiamonds kill chemoresistant hepatic cancer stem cells. ACS Nano. 2014;8(12):12151–12166.
- Xi G, Robinson E, Mania-Farnell B, et al. Convection-enhanced delivery of nanodiamond drug delivery platforms for intracranial tumor treatment. Nanomed Nanotechnol Biol Med. 2014;10(2):381–391.
- Zhang XQ, Chen M, Lam R, et al. Polymer-functionalized nanodiamond platforms as vehicles for gene delivery. ACS Nano. 2009;3(9):2609–2616.
- Barras A, Martin FA, Bande O, et al. Glycan-functionalized diamond nanoparticles as potent E. coli anti-adhesives. Nanoscale. 2013;5(6):2307–2316.
- Cao W, Peng X, Chen X, et al. Facile synthesis of cationic polymer functionalized nanodiamond with high dispersity and antibacterial activity. J Mater Sci. 2017;52(4):1856–1867.
- Gaillard C, Girard HA, Falck C, et al. Peptide nucleic acid–nanodiamonds: covalent and stable conjugates for DNA targeting. RSC Adv. 2014;4(7):3566–3572.
- Nicolau E, Méndez J, Fonseca JJ, et al. Bioelectrochemistry of non-covalent immobilized alcohol dehydrogenase on oxidized diamond nanoparticles. Bioelectrochemistry. 2012;85:1–6.
- Puzyr AP, Burov AE, Bondar VS, et al. Neutralization of aflatoxin B1 by ozone treatment and adsorption by nanodiamonds. Nanotechnol Russia. 2010;5(1-2):137–141.
- Turcheniuk V, Raks V, Issa R, et al. Antimicrobial activity of menthol modified nanodiamond particles. Diamond Relat Mater. 2015;57:2–8.
- Wehling J, Dringen R, Zare RN, et al. Bactericidal activity of partially oxidized nanodiamonds. ACS Nano. 2014;8(6):6475–6483.
- Wu Y, Weil T. Nanodiamonds for biological applications. Phys Sci Rev. 2017;2(6):20160104.
- Baccarin M, Rowley-Neale SJ, Cavalheiro ÉT, et al. Nanodiamond based surface modified screen-printed electrodes for the simultaneous voltammetric determination of dopamine and uric acid. Microchim Acta. 2019;186(3):1–9.
- Bian L, Zong H, Li C, et al. Amperometric determination of hydroquinone and catechol based on nanodiamond powder electrode. Int J Electrochem Sci. 2019;14:186–195.
- Cheng XB, Zhao MQ, Chen C, et al. Nanodiamonds suppress the growth of lithium dendrites. Nat Commun. 2017;8(1):1–9.
- Varley TS, Hirani M, Harrison G, et al. Nanodiamond surface redox chemistry: influence of physicochemical properties on catalytic processes. Faraday Discuss. 2014;172:349–364.
- Alfaro MAQ, Ferro S, Martínez-Huitle CA, et al. Boron doped diamond electrode for the wastewater treatment. J Braz Chem Soc. 2006;17(2):227–236.
- Chen C, Nurhayati E, Juang Y, et al. Electrochemical decolorization of dye wastewater by surface-activated boron-doped nanocrystalline diamond electrode. J Environ Sci. 2016;45:100–107.
- Khataee A, Khataee A, Fathinia M, et al. Kinetic modeling of photoassisted-electrochemical process for degradation of an azo dye using boron-doped diamond anode and cathode with carbon nanotubes. J Ind Eng Chem. 2013;19(6):1890–1894.
- Yotinov I, Belouhova M, Schneider I, et al. Application of nanodiamonds in wastewater treatment technologies. Ecol Eng Environ Protect. 2014;1:51–60.
- Yotinov I, Todorova Y, Schneider I, et al. The effect of nanodiamonds on phenol biodegradation by Pseudomonas sp. strain isolated from polluted sediments. J Nanosci Nanotechnol. 2016;16(7):7696–7706.
- Jin XC, Liu GQ, Xu ZH, et al. Decolorization of a dye industry effluent by Aspergillus fumigatus XC6. Appl Microbiol Biotechnol. 2007;74(1):239–243.
- Sandhya S. Biodegradation of azo dyes under anaerobic condition: role of azoreductase. In: Atacag Erkurt, H., editor. BT - Biodegradation of azo dyes. Springer Berlin Heidelberg; 2010. p. 39–57.
- Singh P, Iyengar L, Pandey A. Bacterial decolorization and degradation of azo dyes. In: Singh, S. N., editor. BT - Microbial degradation of xenobiotics. Springer Berlin Heidelberg; 2012. p. 101–133.
- Saratale RG, Saratale GD, Chang JS, et al. Bacterial decolorization and degradation of azo dyes: a review. J Taiwan Inst Chem Eng. 2011;42(1):138–157.
- de Aragao Umbuzeiro G, Freeman HS, Warren SH, et al. The contribution of azo dyes to the mutagenic activity of the Cristais River. Chemosphere. 2005;60(1):55–64.
- Puvaneswari N, Muthukrishnan J, Gunasekaran P. Toxicity assessment and microbial degradation of azo dyes. Indian J Exp Biol. 2006;44(8):618–626.
- Rajaguru P, Vidya L, Baskarasethupathi B, et al. Genotoxicity evaluation of polluted ground water in human peripheral blood lymphocytes using the comet assay. Mutat Res. 2002;517(1-2):29–37.
- Furukawa K, Simon JR, Chakrabarty AM. Common induction and regulation of biphenyl, xylene/toluene, and salicylate catabolism in Pseudomonas paucimobilis. J Bacteriol. 1983;154(3):1356–1362.
- Topalova Y. Biological control and wastewater management. Sofia (Bulgaria): Publish-Sai-Set-Eco, 2009.
- Eaton AD, Clesceri LS, Rice EW, Greenberg AE. 2005. Standard methods for the examination of water and wastewater. 21st ed. Washington, DC: American Public Health Association (APHA) Press.
- Grekova-Vasileva M. 2009. Bioalgorithms for management of wastewater treatment in textile industry [PhD thesis]. Sofia, Bulgaria: Sofia University.
- Kuznetsov SI, Dubinina GA. Methods of investigation of aquatic microorganisms, Nauka, Moscow. 1989;
- Willetts AJ, Cain RB. Microbial metabolism of alkylbenzene sulphonates. Bacterial metabolism of undecylbenzene-p-sulphonate and dodecylbenzene-p-sulphonate. Biochem J. 1972;129(2):389–402.
- Farr DR, Cain RB. Catechol oxygenase induction in Pseudomonas aeruginosa. Biochem J. 1968;106(4):879–885.
- Veeger C, DerVartanian DV, Zeylemaker WP. 1969. [16] succinate dehydrogenase: [EC 1.3. 99.1 succinate:(acceptor) oxidoreductase]. In Methods in enzymology. (Vol. 13, pp. 81–90). New York: Academic Press.
- Lowry OH, Rosebrough NJ, Farr AL, et al. Protein measurement with the Folin phenol reagent. J Biol Chem. 1951;193(1):265–275.
- Loy A, Maixner F, Wagner M, et al. probeBase—an online resource for rRNA-targeted oligonucleotide probes: new features 2007. Nucleic Acids Res. 2007;35(Database):D800–D804.
- Nielsen PH, Lemmer H, Daims H. 2009. Identification and quantification of microorganisms in activated sludge and biofilms by FISH: introduction. In FISH handbook for biological wastewater treatment. London (United Kingdom): Iwa Publishing.
- Ott RL, Longnecker MT. 2015. An introduction to statistical methods and data analysis. Boston, USA: Cengage Learning.
- Belouhova MV, Schneider ID, Topalova YI. Effect of nanodiamonds on model biphasic azo-detoxification process applied in the critical technological situations. Bulg J Agric Sci. 2013;19(2):
- Haque MM, Haque MA, Mosharaf MK, et al. Decolorization, degradation and detoxification of carcinogenic sulfonated azo dye methyl orange by newly developed biofilm consortia. Saudi J Biol Sci. 2021;28(1):793–804.
- Mishra R, Panda AK, De Mandal S, et al. Natural anti-biofilm agents: strategies to control biofilm-forming pathogens. Front Microbiol. 2020;11:566325.
- Nadar S, Khan T, Patching SG, et al. Development of antibiofilm therapeutics strategies to overcome antimicrobial drug resistance. Microorganisms. 2022;10(2):303.
- Yotinov I, Belouhova M, Foteva A, et al. Application of nanodiamonds in modelled bioremediation of phenol pollution in river sediments. Processes. 2022;10(3):602.
- Schneider ID, Topalova YI. Effect of bioaugmentation on anaerobic wastewater treatment in the dairy industry. J Dairy Sci. 2011;94(9):4389–4397.
- Schneider I, Topalova Y. Microbial structure and functions of biofilm during wastewater treatment in the dairy industry. Biotechnol Biotechnol Equip. 2013;27(3):3782–3786.
- Belouhova M, Schneider I, Chakarov S, et al. Microbial community development of biofilm in Amaranth decolourization technology analysed by FISH. Biotechnol Biotechnol Equip. 2014;28(4):635–642.
- Iqbal A, Ali N, Shang ZH, et al. Decolorization and toxicity evaluation of simulated textile effluent via natural microbial consortia in attached growth reactors. Environ Technol Innov. 2022;26:102284.
- Karnwal A. Textile azo dye decolorization and detoxification using bacteria isolated from textile effluents. BioTechnologia. 2019;100(4):373–385.
- Ihsanullah I, Jamal A, Ilyas M, et al. Bioremediation of dyes: current status and prospects. J Water Process Eng. 2020;38:101680.
- Maqbool Z, Shahid M, Azeem F, et al. Application of a dye-decolorizing Pseudomonas aeruginosa strain ZM130 for remediation of textile wastewaters in aerobic/anaerobic sequential batch bioreactor and soil columns. Water Air Soil Pollut. 2020;231(8):1–18.
- Samuchiwal S, Gola D, Malik A. Decolourization of textile effluent using native microbial consortium enriched from textile industry effluent. J Hazard Mater. 2021;402:123835.
- Belouhova M, Topalova Y. Investigating the functional and structural adaptation changes of biofilm communities toward better azo-dye wastewater treatment. BJECC. 2016;6(4):309–318. Article no. BJECC.2016.028