Abstract
The human microbiome plays a very important role in the normal functioning of both metabolic and immune systems. Changes in gut microbiota (dysbiosis) possibly contribute to the development of metabolic and autoimmune diseases. There is close association between the human gut microbiome and Vitamin D metabolism. The objective of our study was to characterize the composition of the gut microbiome in patients with type 2 diabetes mellitus (T2DM) in comparison to controls with normal glucose tolerance, and to correlate the Vitamin D status with the changes in the gut microbiome. Totally, 15 patients with T2DM and 16 controls were enrolled in the study. The complex gut microbiome was analysed by next generation sequencing (NGS) in both groups, additionally divided into subgroups according to serum 25-hydroxyVitamin D (25[OH]D) levels. We found a significant decrease in butyrate-producing bacteria Eubacterium spp. and an increase in lipopolysaccharide (LPS) - positive bacteria Enterobacter spp., Escherichia spp. and Pseudomonas spp. in T2DM patients compared to controls. Control individuals with 25[OH]D levels of over 80 nmol/L were characterized with significantly decreased amount of Escherichia spp. and Pseudomonas spp. and increased Eubacterium spp. On the other hand, 25[OH]D < 80nmol/L levels in diabetic patients were related with lower content of butyrate-producing bacteria and higher levels of LPS-positive bacteria. The results showed an influence of gut microbiome on the glucose homeostasis and a relationship between Vitamin D levels and the gut microbiome.
Introduction
The human microbiome includes about 100 trillion microbes, most of which live in the gastrointestinal tract. This microbiome is composed of communities of bacteria, viruses and fungi and plays a very important role in the normal functioning of the methabolism and the immune system. Changes and dysregulation of gut microbiota (dysbiosis) can contribute to the development of some metabolic and autoimmune diseases [Citation1]. Variations in diet could change by more than 10% the gut microbiome as well [Citation2, Citation3]. Interventions on gut microbiome in mice disturbed the glucose metabolism, accompanied by macrophage accumulation in white adipose tissue, and led to insulin insensitivity [Citation3, Citation4]. It is known that the microbiome could contribute to triggering chronic low-grade inflammation that is seen in patents with insulin resistance and type 2 diabetes mellitus (T2DM) [Citation1].
Vitamin D modulates the functioning of the gut microbiome. It regulates cytokines and T-cell differentiation and function during the process of cell inflammation. The active form of Vitamin D (1,25(OH)2D) stimulates macrophages in producing antimicrobial peptides [Citation5, Citation6]. It also suppresses T cells producing interferon-γ and interleukin-17, leading to decreased inflammatory activity [Citation7, Citation8]. On the other hand, the gut microbiome is regulated by the complex interplay between T-cells and dendritic cells [Citation9]. By modulation of cell-mediated immune response, Vitamin D prevents the development of dysbiosis, which is detrimental for glucose homeostasis. Glucose intolerance is developed along with dysbiosis and the recovery of healthy gut microbiota can improve glucose sensitivity in patients with T2DM [Citation10].
Over the course of evolution, humans live in symbiosis with vast numbers of microbial species. The evaluation of the variety of effects of human-related microbes, their functional gene capacity and the spectrum of biomolecules they generate in the human body has been assessed only relatively recently, largely due to advances in high-generation and high-performance sequencing platforms and mass spectrometry [Citation11]. Sequencing platforms allow assessment of microbiota composition by sequencing of gene biomarkers, e.g. sequencing and classification of 16S ribosomal RNA or specific amplicons, to assess the identity and distribution of microbial, bacterial or fungal diversity. These techniques can be used for studies that identify the characteristics of the microbiota associated with specific clinical conditions. Changes in microbial diversity lead to intestinal dysbiosis and can cause inflammation, lipid accumulation and insulin resistance [Citation12].
The objective of our study was to characterize the composition of the gut microbiome in patients with type 2 diabetes mellitus in comparison to controls with normal glucose tolerance, and to assess the relationship between their Vitamin D status and the gut microbiome changes.
Subjects and methods
Ethics statement
All subjects signed an informed consent form before the investigation.
Data collection
A total of 15 patients (11 females and 4 males) with type 2 diabetes mellitus (T2DM) and 16 controls (11 females and 5 males) with normal glucose tolerance were included in the study. The average age in the group of T2DM patients was 57.3 ± 10 and in the group of controls, 51.4 ± 5.6. The selection criteria for our patients and controls were as follows:
Patients with type 2 diabetes mellitus and good glycemic control; non-obese; no antibiotics and prebiotics in the last 3 months before analysis; medication with metformin, uniform in all patients
Controls with normal glucose tolerance, sex and age matched with the patients; non-obese; no antibiotics and prebiotics in the last 3 months before analysis; no diseases; no medication.
They were additionally divided according to the levels of 25(OH)D into (i) T2DM patients with low 25(OH)D levels (49.3 nmol/L ± 11.3) and normal 25(OH)D levels (91.0 nmol/L ± 11.6) and (ii) controls with low 25(OH)D levels (49.5 nmol/L ± 24.0) and normal 25(OH)D levels (96.8 nmol/L ± 11.3).
Personal and laboratory data were collected for all participants, there was no difference between the groups in cholesterol and triglycerides levels, as shown in .
Table 1. Laboratory parameters in patients and controls (on average ± standard deviation).
Sampling and gut microbiome analysis
The feces were collected in special sterile containers (with stabilizing media), following the instructions of the manufacturer – material was taken from three different places. Feces was sampled and delivered to the laboratory within a period of 24-48 h (including transportation), kept at 4 °C, without freezing. The complex gut microbiome was analysed via next generation sequencing (NGS) of 16S rRNA, which was performed by an external diagnostics provider (Ganzimmun Diagnostics AG, Mainz, Germany) in patients with type 2 diabetes and disease-free controls. The study of the microbiome included assessment of bacterial dysbiosis, analysis of the species composition of the intestinal microflora, determination of the enterotype and distribution of bacterial strains, including assessment of the mucosa-protective microflora. Determination was made for: enterotype (predominance of type/genus Bacteroides, Prevotella or Firmicutes); distribution of the most important bacterial strains; the Firmicutes/Bacteroidetes ratio; muco-protective microflora. Investigated bacteria are listed in . Shannon index, which provides information about both richness and evenness within a sample, was used for the determination of alpha-diversity. Beta-diversity analysis was done by comparing the abundance of species in each separate sample.
Table 2. List of investigated bacteria.
The amount of each bacterium was recorded and presented in percentage for each sample. The participants were distributed in different groups. First, the groups of T2DM and controls were determined and average levels in % for each species were calculated. Then, controls and T2DM patients were divided into two subgroups according to levels of 25(OH)D (low and normal) and calculations for average levels (and standard deviations) were done. Student’s T- test was used for statistical analysis. Differences were considered statistically significant at the p < 0.05 level.
Determination of Vitamin D serum levels
Determination of 25(OH)D was performed by a validated liquid chromatography-tandem mass spectrometry method developed in house, utilizing extraction with hexane, as it was described by Filipov et al. [Citation13]. The method was calibrated via commercial calibrators traceable to the US National Institute of Standards and Technology Standard Reference Material 972 and was validated according to US Food and Drug Administration guidance requirements, with documented selectivity and matrix effects within 15%, accuracy and precision within 7.5%, extraction recoveries averaging 57% to 73%, and linearity range of 3.0 to 300.0 nmol/L (R2 > 0.99). The method was certified by the UK Vitamin D External Quality Assessment Scheme.
Results
In our study, the analysis of biodiversity (Shannon index for alpha-diversity) and other bioindicators (pH-value of feces, Firmicutes/Bacteroidetes ratio, Prevotella/Bacteroidetes ratio) in T2DM patients and controls did not show a significant deviation from the reference values and did not differ statistically between patients and controls.
The comparison between patients and controls with the same levels of Vitamin D showed some changes in the microbiome associated with T2DM itself. In the groups with low Vitamin D levels, there was a statistically significant reduction in butyrate-producing bacteria Eubacterium spp. in the group of patients compared to the control subjects (p = 0.023, ).
Figure 1. Significantly lower amount of Eubacterium spp. in T2DM patients compared to controls in groups with low 25(OH)D levels (p = 0.023).
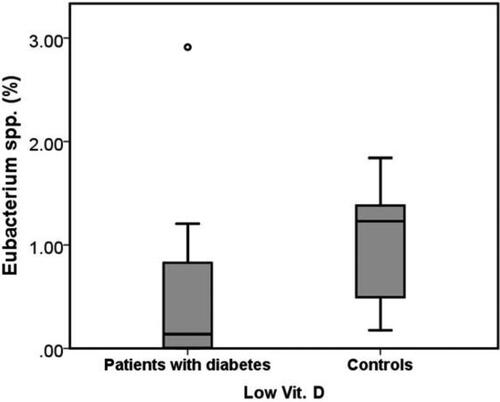
Compared to controls, T2DM patients had approximately 4 times more representatives of Enterobacter spp., Escherichia spp. and Pseudomonas spp. As shown in , the abundance of Enterobacter spp. was statistically significantly higher in T2DM patients compared to controls both in the groups with low and normal 25(OH)D levels (p = 0.004 and p = 0.02, respectively). In diabetic patients with low 25(OH)D status, there was an increased number of sulfate-reducing bacteria Bilophila wadsworthia, albeit statistically non-significant.
Figure 2. Amount of Enterobacter spp. and Bilophila wadsworthia in T2DM patients and controls.
Asterisks indicate statistically significant differences (p < 0.05).
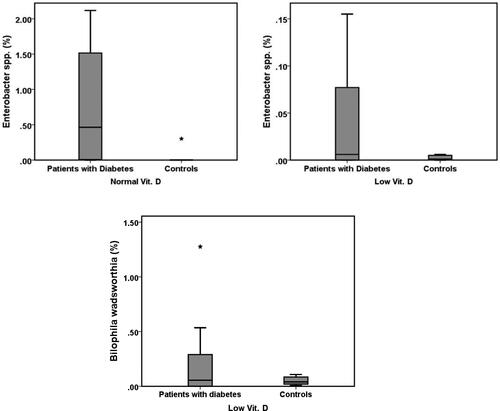
The amounts of Escherichia spp. and Pseudomonas spp. were higher in T2DM patients compared to controls in the low 25(OH)D levels groups. For Escherichia spp. the increase was statistically significant (р = 0.013); whereas for Pseudomonas spp. the increase was non-significant (p = 0.074), .
Figure 3. Increase in the amount of Escherichia spp. and Pseudomonas spp. in patients with T2DM compared to controls in groups with low Vitamin D levels.
Asterisks indicate statistically significant differences (p < 0.05).
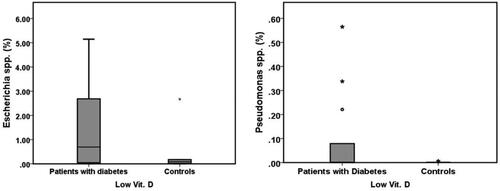
Next, we analyzed the microbiota in different subgroups, depending on the levels of 25(OH)D. The comparison of the subgroups with low (<80 nmol/L) and normal (>80 nmol/L) 25(OH)D levels between patients and controls showed changes in the microbiome associated with 25(OH)D. Regarding the bioindicators, there were no significant differences related to Vitamin D, although there was a slight increase in biodiversity and Firmicutes/Bacteroidetes and Prevotella/Bacteroidetes ratios, mainly in controls with normal levels of 25(OH)D.
An increase in Butyrivibrio crossotus was observed in controls and T2DM patients without Vitamin D deficiency compared to controls and patients with low Vitamin D levels, respectively, which was statistically non-significant. There was some increase in the amount of butyrate-producing bacteria Eubacterium spp. (p = 0.065) and a significant decrease in the amount of Escherichia spp. (p = 0.012) and Pseudomonas spp. (p = 0.05) was observed in diabetic patients with 25(OH)D levels > 80 nmol/L ().
Discussion
The intestinal flora consists of 1014 bacteria, whose total genome is called ‘microbiome’. The spectrum of intestinal bacteria varies greatly, but more than 99% of their total number consists of 30-40 bacterial species. They are classified into three main categories according to their pathogenic effect: beneficial bacteria (30%), harmful bacteria (10%) and conditionally pathogenic bacteria (60%) [Citation14, Citation15]. Currently, a substantial body of studies have investigated the intestinal flora in relation to the energy metabolism, providing evidence for the potential role of gut microbiota in the development of T2DM. The modification of intestinal flora by different food and drugs has been investigated in the prevention and treatment of diabetes [Citation16, Citation17]. In this study we compared the composition of the intestinal microbiome between T2DM patients and control subjects in relation to 25(OH)D serum levels. The alpha-diversity analysis, determined by Shannon index, did not show significant differences between T2DM patients and controls, as well as between the groups with higher and lower Vitamin D levels. This is consistent with most other studies, performing comparative analysis of microbiota in diabetic patients and healthy controls and in people with different levels of Vitamin D. A recent study reported an inverse correlation between alpha-diversity and Vitamin D-deficiency in obese women [Citation5], but all participants in our study had BMI <30 kg/m2 and did not show differences for this parameter. A study on microbiota—vitamin D—diabetes interactions found a significant decrease in the relative abundance of bacteria from the phylum Firmicutes, class Clostridia in prediabetic individuals with increased vitamin D level [Citation18]. That study, however, included only African American men and has considerable sex and ethnic limitations to be conclusive.
The first important observation from the beta-diversity analysis in our study was the difference in the abundance of intestinal butyrate producing bacteria between T2DM patients and control subjects, especially with 25(OH)D levels less than 80 nmol/L. It was most pronounced for Eubacterium spp., which belongs to the group of beneficial bacteria producing short-chain fatty acids (SCFAs). SCFAs exist at higher content in healthy subjects compared to diabetic patients [Citation19]. Butyrate is an important substance which keeps the colon healthy and reduces intestinal inflammatory processes through stimulating the formation of regulatory T cells and inhibiting the production of proinflammatory cytokines by macrophages and dendritic cells. Butyrate also increases the oxygen consumption of colonocytes and maintains the phenomenon of mucosal ‘physiological hypoxia’, which contributes to the maintenance of the intestinal barrier function. Recently it has also been shown that butyrate has important immunomodulatory functions [Citation20]. Reduced number of butyrate producers can stimulate inflammatory processes, increase the permeability of the intestinal mucosa and promote the manifestation of inflammatory diseases, irritable bowel syndrome, food intolerance and celiac disease [Citation21]. It was found that SCFAs could directly control the number and function of islet β cells and glucose metabolism [Citation22].
The content of Eubacterium spp. differed significantly between T2DM patients with low and higher Vitamin D serum levels as well, with a close to significant increase in the latter (p = 0.065). Recent studies demonstrated that human gut microbiome and Vitamin D metabolism are closely related and interdependent [Citation23, Citation24]. There is a dynamic bidirectional interplay between the active Vitamin D metabolites and butyrate-producing bacteria [Citation25–27]. Providing with specific butyrate-producing bacteria or with a substrate for them can stimulate the mechanisms for Vitamin D absorption and activation [Citation24]. Other studies suggest that the Vitamin D intake and its circulating levels are important for maintaining the immune homeostasis, partially via modulating the gut microbiome [Citation28].
We found a significantly higher amount of Enterobacter spp., Pseudomonas spp. and Escherichia coli in T2DM patients. These bacteria belong to the LPS (lipopolysaccharide)-positive family of gram-negative bacteria that carry LPS acting as endotoxin, which after penetration into the intestinal mucosa induces inflammatory processes. Bacterial lipopolysaccharides (LPS) activate the immune system by inducing an inflammatory response, cytokine production and accumulation of inflammatory cells [Citation29, Citation30]. Increase in plasma LPSs, defined as metabolic endotoxemia, has been associated with the onset of insulin resistance and type 2 diabetes [Citation31]. Several studies report a rise in the content of Escherichia coli in diabetic patients with higher glycosylated hemoglobin [Citation32]. The amount of Pseudomonas spp. and Escherichia coli was significantly lower in T2DM patients with higher Vitamin D serum levels. It has been shown that Vitamin D may influence the gut microbiota and exert anti-inflammatory and immune-modulating effects [Citation33, Citation34].
Sulfate-reducing bacteria are anaerobic bacteria that produce energy by reducing sulfate and forming large amounts of sulfides. The metabolite of these bacteria is hydrogen sulfide, which has cytotoxic properties and can inhibit butyric oxidation, which is essential for the energy supply of colonocytes. The spread of sulfate-reducing bacteria can lead to chronic inflammation of the intestinal epithelium [Citation35]. We discovered higher levels of Bilophila wadsworthia in diabetic patients, especially in the Vitamin D deficient group. An increased number of sulfate-reducing bacteria in T2DM patients was also reported [Citation36].
This study has several limitations, including a small number of patents and control subjects, optimized but not full range of microbiome investigations and relatively simple statistical analysis, all of which could explain why some of the observations were only trends that did not reach statistical significance. Nevertheless, the relationship between gut microbiota and Vitamin D status in T2DM patients and some differences in comparison to healthy controls are demonstrated and could support the role of Vitamin D supplementation in this population.
Within the limitations of the study, the gut microbiome in patients with T2DM was characterized by a decrease in butyrate-producing bacteria and an increase in sulfate-reducing and LPS-positive bacteria [Citation37]. Higher Vitamin D levels were associated with a significant increase in butyrate-producing Eubacterium spp. and a decrease in Escherichia spp. and Pseudomonas spp. Accumulating evidence of the influence of microbiome on the inflammatory processes and glucose homeostasis suggests that modulation by probiotics/prebiotics and Vitamin D supplementation could be strongly beneficial in the prevention and treatment of T2DM.
Conclusions
Our study analysed gut microbiota by 16S rRNA sequencing and revealed significant beta-diversity differences between T2DM patients and controls with normal glucose tolerance. The results suggest that modulation by probiotics/prebiotics and Vitamin D supplementation may be beneficial in T2DM patients. We take note for the limitation of our study because of the small size of the analysed population, but to the best of our knowledge, this is the first microbiota study in Bulgarian diabetic patients. There is large variability in the gut microbiota across studies from different populations (ethnicity) worldwide and here we presented the first report for Bulgarian subjects, worthy to be extended by studies of larger size.
Author contributions
All authors have read and approved the paper, and meet met the criteria for authorship as established by the International Committee of Medical Journals Editors.
Disclosure statement
The authors report no conflict of interest.
Additional information
Funding
References
- Wang PX, Deng XR, Zhang CH, et al. Gut microbiota and metabolic syndrome. Chin Med J (Engl). 2020;133(7):1–8.
- Salonen A, Lahti L, Salojärvi J, et al. Impact of diet and individual variation on intestinal microbiota composition and fermentation products in obese men. ISME J. 2014;8(11):2218–2230.
- Sanz Y, Olivares M, Moya-Pérez Á, et al. Understanding the role of gut microbiome in metabolic disease risk. Pediatr Res. 2015;77(1-2):236–244. Epub 2014 Oct 14. PMID: 25314581.
- Caesar R, Reigstad CS, Bäckhed HK, et al. Gut-derived lipopolysaccharide augments adipose macrophage accumulation but is not essential for impaired glucose or insulin tolerance in mice. Gut. 2012;61(12):1701–1707.
- Al-Khaldy NS, Al-Musharaf S, Aljazairy EA, et al. Serum vitamin D level and gut microbiota in women. Healthcare (Basel). 2023;11(3):351.
- Charoenngam N, Shirvani A, Kalajian TA, et al. The effect of various doses of oral vitamin D3 supplementation on gut microbiota in healthy adults: a randomized, double-blinded, dose-response study. Anticancer Res. 2020;40(1):551–556.
- Waterhouse M, Hope B, Krause L, et al. Vitamin d and the gut microbiome: a systematic review of in vivo studies. Eur J Nutr. 2019;58(7):2895–2910.
- Aranow C. Vitamin d and the immune system. J Investig Med. 2011;59(6):881–886.
- Weiss ST. Bacterial components plus vitamin D: the ultimate solution to the asthma (autoimmune disease) epidemic? J Allergy Clin Immunol. 2011;127(5):1128–1130.
- Gérard C, Vidal H. Impact of gut microbiota on host glycemic control. Front Endocrinol (Lausanne). 2019;10:29.
- Malla MA, Dubey A, Kumar A, et al. Exploring the human microbiome: the potential future role of next-generation sequencing in disease diagnosis and treatment. Front Immunol. 2018;9:2868.
- Fan Y, Pedersen O. Gut microbiota in human metabolic health and disease. Nat Rev Microbiol. 2021;19(1):55–71.
- Filipov JJ, Petrova M, Metodieva T, et al. Vitamin D influences the prevalence of non-cutaneous carcinomas after kidney transplantation? Biotechnol Biotechnol Equip. 2018;32(5):1266–1270.
- Qin J, Li R, Raes J, et al. A human gut microbial gene catalogue established by metagenomic sequencing. Nature. 2010;464(7285):59–65.
- Magne F, Gotteland M, Gauthier L, et al. The firmicutes/bacteroidetes ratio: a relevant marker of gut dysbiosis in obese patients? Nutrients. 2020;12(5):1474.
- Parada Venegas D, De la Fuente MK, Landskron G, et al. Short chain fatty acids (SCFAs)-mediated gut epithelial and immune regulation and its relevance for inflammatory bowel diseases. Front Immunol. 2019;10:277. Erratum in: front Immunol. 2019;10:1486.
- Yip W, Hughes MR, Li Y, et al. Butyrate shapes immune cell fate and function in allergic asthma. Front Immunol. 2021;12:628453.
- Ciubotaru I, Green SJ, Kukreja S, et al. Significant differences in fecal microbiota are associated with various stages of glucose tolerance in African American male veterans. Transl Res. 2015;166(5):401–411.
- Gorvitovskaia A, Holmes SP, Huse SM. Interpreting Prevotella and Bacteroides as biomarkers of diet and lifestyle. Microbiome. 2016;4:15. 10.1186/s40168-016-0160-7.
- Kovatcheva-Datchary P, Nilsson A, Akrami R, et al. Dietary fiber-induced improvement in glucose metabolism is associated with increased abundance of Prevotella. Cell Metab. 2015;22(6):971–982.
- Leite AZ, Rodrigues NC, Gonzaga MI, et al. Detection of increased plasma interleukin-6 levels and prevalence of Prevotella copri and Bacteroides vulgatus in the feces of type 2 diabetes patients. Front Immunol. 2017;8:1107.
- Evivie SE, Huo GC, Igene JO, et al. Some current applications, limitations and future perspectives of lactic acid bacteria as probiotics. Food Nutr Res. 2017;61(1):1318034.
- Thomas RL, Jiang L, Adams JS, et al. Vitamin D metabolites and the gut microbiome in older men. Nat Commun. 2020; 11(1):5997.
- De Filippis F, Pasolli E, Ercolini D. The food-gut axis: lactic acid bacteria and their link to food, the gut microbiome and human health. FEMS Microbiol Rev. 2020;44(4):454–489.
- Karlsson FH, Tremaroli V, Nookaew I, et al. Gut metagenome in european women with normal, impaired and diabetic glucose control. Nature. 2013;498(7452):99–103.
- Forslund K, Hildebrand F, Nielsen T, MetaHIT consortium, et al. Disentangling type 2 diabetes and metformin treatment signatures in the human gut microbiota. Nature. 2015;528(7581):262–266. Epub 2015 Dec 2. Erratum in: nature. 2017;545(7652):116.
- Teixeira TS, Grześkowiak LM, Salminen S, et al. Faecal levels of bifidobacterium and Clostridium coccoides but not plasma lipopolysaccharide are inversely related to insulin and HOMA index in women. Clin Nutr. 2013;32(6):1017–1022.
- Ignacio A, Fernandes MR, Rodrigues VA, et al. Correlation between body mass index and faecal microbiota from children. Clin Microbiol Infect. 2016;22(3):258.e1-8–258.e8.
- Delzenne NM, Cani PD, Everard A, et al. Gut microorganisms as promising targets for the management of type 2 diabetes. Diabetologia. 2015;58(10):2206–2217.
- Page MJ, Kell DB, Pretorius E. The role of lipopolysaccharide-induced cell signalling in chronic inflammation. Chronic Stress. 2022;6:247054702210763.
- Mohammad S, Thiemermann C. Role of metabolic endotoxemia in systemic inflammation and potential interventions. Front Immunol. 2020;11:594150.
- Dordević D, Jančíková S, Vítězová M, et al. Hydrogen sulfide toxicity in the gut environment: meta-analysis of sulfate-reducing and lactic acid bacteria in inflammatory processes. J Adv Res. 2021;27:55–69.
- Cesario V, Di Rienzo TA, Campanale M, et al. Methane intestinal production and poor metabolic control in type I diabetes complicated by autonomic neuropathy. Minerva Endocrinol. 2014;39(3):201–207. PMID: 25068305.
- Akimbekov NS, Digel I, Sherelkhan DK, et al. Vitamin D and the host-gut microbiome: a brief overview. Acta Histochem Cytochem. 2020;53(3):33–42.
- Yamamoto EA, Jørgensen TN. Relationships between vitamin D, gut microbiome, and systemic autoimmunity. Front Immunol. 2019;10:3141.
- Qin J, Li Y, Cai Z, et al. A metagenome-wide association study of gut microbiota in type 2 diabetes. Nature. 2012;490(7418):55–60.
- Dimova I, Yanachkova V, Boneva T, et al. Microbiome characterization in type 2 diabetes mellitus-relation to vitamin D levels. Diabetes Technol Ther. 2022;24: A186–A186.