Abstract
Heat shock protein 70 (Hsp70) is a crucial member of the Hsp family, which is present in many animals, and acts as a chaperone to protect the organism from damage caused by various environmental stresses, particularly unfavorable temperatures. In this study, we used homologous gene search and domain analysis to identify sixteen Hsp70 genes (named as CiHSP genes) from the genome of grass carp (Ctenopharyngodon idella). These genes were classified into ten subfamilies based on their conserved structures and phylogenetic analysis. To investigate the biological functions of CiHSP genes in grass carp, we analyzed public RNA-Seq data, and found that most members of the CiHSP gene family were highly expressed in the brain and kidney, suggesting potential roles in protecting brain cells and participating in fish immunological processes. Additionally, these CiHSP genes were characterized as responding to high density and high temperature stress, with most members significantly upregulated under high temperature conditions. These findings demonstrate the critical roles of CiHSP genes in grass carp development and their response to environmental stress, which will provide valuable insights for determining their function and potential application in fish production in the future.
Introduction
Grass carp (Ctenopharyngodon idella) is a major freshwater fish species in China, widely distributed throughout the country, and is known as the ‘king of freshwater fish’. It plays a vital role in the aquaculture industry, with annual production reaching 5.5 billion kg, making it the leading fish in terms of production for many years [Citation1]. It’s delicious meat, high nutritional value and affordable price make it popular among Chinese consumers. However, the increasing demand for artificial culture and the continuously high summer temperatures have made culture density and temperature the primary factors affecting the quality of fish growth [Citation2]. High stocking density can make grass carp vulnerable to disease due to a lack of the disease resistance needed for survival [Citation3,Citation4]. High densities also result in competition for feed and space among the fish, leading to stress and other challenges that result in slow growth or even death [Citation3,Citation5,Citation6]. Temperature is another key factor that directly affects metabolism in fish, which are temperature-sensitive animals. Unsuitable temperatures not only impact the growth of fish but also make them more susceptible to attacks from viral pathogens, significantly reducing their survival rate [Citation7,Citation8]. Both heat and cold stress can inhibit the growth and affect the physiological activities of fish, potentially leading to death [Citation9,Citation10]. Previous research has shown that the optimal water temperature for grass carp growth is between 18 °C and 25 °C, and that growth is more favorable as the temperature increases within this range [Citation7].
Heat shock proteins (Hsps) are a class of stress proteins that are widely found in eukaryotes and prokaryotes [Citation11]. Hsps are divided into constitutive proteins expressed by cells under normal conditions and inducible proteins expressed in large quantities when an organism is exposed to stress such as high temperature or hypoxia in order to maintain cellular homeostasis and protect the cell [Citation12,Citation13]. Hsps with a molecular weight of around 70 kDa are known as heat shock protein 70s (Hsp70s) [Citation14]. Hsp70, a crucial member of the Hsps family, has been extensively studied since the 1960s for its role as a molecular chaperone in various biochemical processes, such as the assembly and folding of protein polypeptide chains, protein translocation across membranes, degradation, and helping proteins enter the mitochondria and endoplasmic reticulum, as well as repairing denatured proteins to protect the organism [Citation15–17]. Hsp70 genes act as an effective buffer in tissues in response to density [Citation18] and temperature changes [Citation19] as part of a complex adaptive response. In recent years, with the advancement of high-throughput sequencing techniques, researchers have studied the expression patterns of a large number of Hsp70 genes in fish at the genomic and transcriptomic level. For example, Song et al. [Citation20] have identified Hsp70 genes in channel catfish, and found Hsp70 genes significantly up/down-regulated after bacterial challenges [Citation20]. Similarly, there are 17 members of Hsp70 family identified in the genome of large yellow croaker, which have shown significant upregulation or downregulation after cold or heat stress, suggested their key function in response to temperature stress [Citation21]. The genome of grass carp has been well characterized, providing the opportunity for comprehensive analysis of Hsp70 genes in this species.
In this study, we performed a genome-wide in silico identification and characterization of Hsp70 genes in grass carp, including identification, gene structure, gene duplication, and its expression profiles under different tissues or various stresses.
Materials and methods
Identification of the Hsp70 gene family in grass carp
Annotation information, genome sequences, transcriptome data and amino acid sequences for grass carp were downloaded from grass carp genome website (http://www.ncgr.ac.cn/grasscarp/). The Hsp70 genes from human (Homo sapiens), zebrafish (Danio rerio), mouse (Mus musculus) and chicken (Gallus gallus), were also downloaded from NCBI (https://www.ncbi.nlm.nih.gov/, see Supplemental data). To identify the Hsp70 genes in grass carp, we utilized various bioinformatics tools such as BlastP, HMMER and SMART. First, we used BlastP to search the grass carp genome with the amino acid sequences of Hsp70 genes from four animals (see above description) as query sequences, setting the e-value to 1e-05, and requiring at least 80% coverage. Next, we used HMMER to search for the Hsp70 structural domain in the grass carp genome using the HMM file from the Pfam protein family database (PF00012), with an e-value of 1e-02 [Citation22]. We then screened the predicted Hsp70 protein sequences with SMART to confirm the presence of the Hsp70 structural domain. Finally, we used the nomenclature of Hsp70 genes in four model animals to classify and name the identified Hsp70 genes in grass carp.
Phylogenetic analysis of CiHSP genes
Protein sequences of the Hsp70 gene in grass carp and four model animal species (human, zebrafish, mouse and chicken) were aligned with the ClustalW program with default parameters, and a phylogenetic tree of Hsp70 genes was constructed based on multiple sequences alignment results using the neighbor-joining (NJ) method in MEGA 11 software. The parameters were set as genetic distance, Poisson distance; 1000 Bootstraps [Citation23,Citation24].
Conserved motif analysis of CiHSP genes
The conserved motifs in Hsp70 were identified using MEME software (version 5.5.0), and we set the parameters: (1) the optimum motif width was set to ≥10 and ≤50; (2) the maximum number of motifs was set to identify 10 motifs; (3) occurrences of a single motif distributed among the sequences with model: 0 or 1 per sequence (-modzoops) [Citation25]. The results were displayed using TBtools software [Citation26].
Gene duplication analysis of CiHSP genes
We used BLAST to compare grass carp proteins with each other, and the results were analyzed using MCSanX software were used to identify and characterize gene duplication in grass carp genome [Citation27]. All positional information and gene duplications about CiHsp70 genes were retrieved, and they were displayed and analyzed using CIRCOS software [Citation28].
Gene regulatory network analysis of CiHSP genes
We downloaded the zebrafish gene regulatory network (GRN) from the STRING database with accession number 7955, which included 26424 genes and more than 22.5 million interactions (links). We performed a BLAST search of all grass carp proteins against zebrafish proteins with an e-value cut-off of 1e-05, and identified the hits with the highest scores as homologous genes for the grass carp genes [Citation29]. We also performed a BLAST search of all zebrafish proteins against grass carp proteins using the same parameters, and identified the hits with the highest scores as homologous genes for the zebrafish genes. These two genes were identified as homologous pairs based on the results of the two BLAST searches. We then constructed the GRN of grass carp based on the GRN of zebrafish using the homologous pairs. We retrieved and analyzed sub-networks containing CiHSP70 genes, and viewed the results using the Cytoscape software [Citation30]. We annotated the genes in these sub-networks using gene ontology information based on the grass carp genome annotation information. We subjected the sub-networks to Gene Ontology (GO) enrichment analysis using topGO package on R platform, with a threshold level of 0.05. We displayed the representations of the most significant terms and assigned the high enrichment terms as GRN functions based on the software’s protocol [Citation31].
Expression analysis of the CiHSP genes in tissue development and response to stresses
Three datasets of RNA-Seq experiments were downloaded from public databases to investigate the expression profile of CiHSP genes in grass carp. The expression profiles of tissues during the development of grass carp were downloaded from the grass carp genome website, described as above. This data includes six tissues, such as head kidney, embryo, liver, spleen, brain and kidney, which are TPM values and analyzed using R platform. The high density stress dataset was downloaded from the SRA database of NCBI (Accession number: PRJNA587607), and all reads of this RNA-seq were mapped to the transcript sequences of grass carp genome using the Salmon software (version 0.12.0), and the expression level of each gene (FPKM value) was calculated by Salmon’s subroutine quant, and then analyzed and clustered using the R program as described above [Citation32]. The heat stress dataset was also downloaded from the SRA database of NCBI (Accession number: PRJNA862271), and the data was analyzed as described above. The CiHSP gene expression data were extracted from the above data, and they were listed in the Supplemental Files.
Results
Genome-wide identification of CiHSP genes in grass carp
There are 16 members of the Hsp70 gene family identified in the genome of the grass carp through homologous search and domain analysis (). These genes, referred to as CiHSP, are highly conserved in fish and are named on the basis of their homologous genes in four model animals. The lengths of the CiHSP genes vary greatly, ranging from 438 to 1046 amino acids, and the number of exons also differs among the members. For example, CiHSP1a1, CiHSP1a2 and CiHSP1b have only one or two exons, while other members have more than five exons, with the longest Hsp70 gene (CiHSPhyou1) containing 23 exons. These results suggest that although CiHSP genes are highly conserved across animals, the members of the CiHSP gene family undergo different genetic regulation processes.
Table 1. Summary of the CiHSP genes in grass carp genome.
Phylogenetic analysis and conservative motifs analysis of CiHSP genes
To better understand the phylogenetic relationships among the CiHSP genes, an unrooted phylogenetic neighbor-joining (NJ) tree was generated using the protein sequences of a total of 79 Hsp70 genes from grass carp and four model species (human (Homo sapiens), zebrafish (Danio rerio), mouse (Mus musculus) and chicken (Gallus gallus)). The results of this analysis (), indicate that Hsp70 genes are highly conserved among these species, with most members also present in grass carp, which is consistent with their distribution in other fish species such as channel catfish and large yellow croaker [Citation20,Citation21]. These CiHSP genes were then analyzed using the MEME software, and the results showed that most of them have a conserved HSP domain, which is 602 amino acids in length, but CiHSP12 differ among the members (). For example, CiHSP1a1, CiHSP1b, CiHSP8, CiHSP8a, CiHSP8b, and CiHSP9 all have all the motifs, with similar distribution patterns, while CiHSP1a2 is missing three motifs (motifs 6, 7, and 9), which may contribute to the functional differentiation of these CiHSP genes. Similarly, CiHSP4a is missing motif 5, while CiHSP4b and CiHSP4b are missing motifs 4 and 5, probably leading to divergence in their biological functions.
Figure 1. Phylogenetic analysis of the CiHSP genes in grass carp.
Note: Molecular phylogeny analysis of CiHSP genes was performed using MEGA11. Red circles represent subfamily HSP1; Light green squares represent subfamily HSP8; Blue triangles represent subfamily HSP5; Purple triangles represent subfamily HSP9; Pink diamonds represent subfamily HSP13; Brown green circles represent subfamily HSPh1; Yellow circles represent subfamily HSP4; Blackish green diamonds represent subfamily HSP14; Cyan hollow circles represent HSPhyou1; Deep blue hollow squares represent subfamily HSP12.
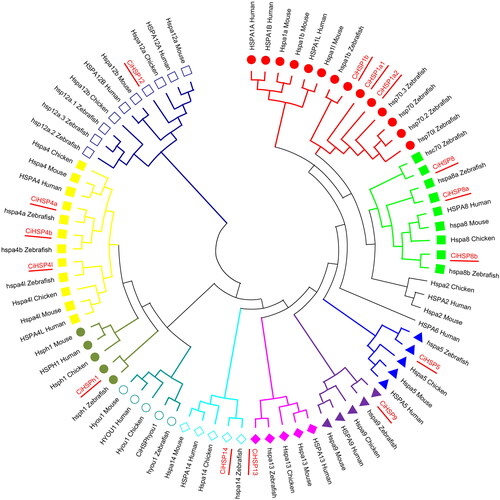
Chromosome distribution and gene duplication analysis of CiHSP genes
According to the results of gene duplication analysis, all 16 CiHSP genes are located on 14 chromosome segments (). Most of the CiHSP genes are located individually on these chromosome segments, with the exception of CiHsp8a and CiHsphyou1, which are located adjacent to each other on chromosome segment CI304. In addition, only one duplication event was detected among these CiHSP genes, specifically the duplication of the HSP8a and HSP8b genes. This suggests that it may be present in fish and may play a role in various biological processes throughout the lifetime of fish.
Figure 3. Chromosomal distribution and gene duplication analysis of the CiHSP genes in grass carp.
Note: All genes duplications were scanned and identified using MCSanX software, and they were displayed using CIRCOS software. All CiHSP genes were labeled with red short line, and genes with duplication event were linked with a black curve.
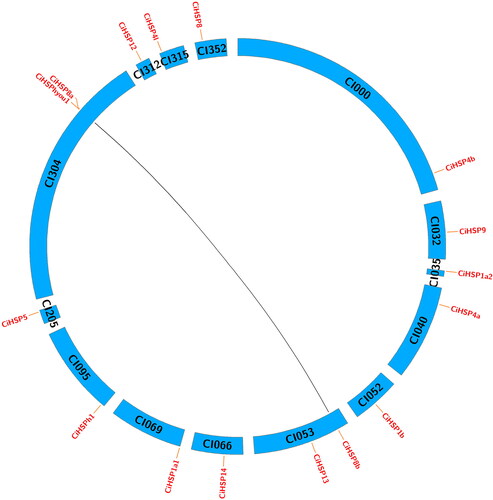
Genetic regulatory and biological function analysis of CiHSP genes
To assess the biological functions of CiHSP genes in grass carp, we reconstructed a genetic regulatory network (GRN) containing CiHSP genes and their interacting genes, consisting of 212 genes and 1344 interactions (). Among these genes, 12 CiHSP genes were identified as important hub genes with numerous interactions, suggesting that CiHSP genes play critical roles in the lifespan of grass carp. The GRN of CiHSP genes was further analyzed through functional enrichment analysis, which revealed that these genes are primarily involved in protein folding and chaperon binding (), which is consistent with the known functions of HSP genes in many animals. Additionally, they were found to play a role in disulfide oxidoreductase activity, which is an indicator of response to various stresses. Similarly, ATPase regulator activity was also identified as an important function in response to growth, development or environmental stress. These results suggest that CiHSP genes play key roles in these pathways.
Figure 4. Gene regulatory network analysis of CiHSP genes in grass carp.
Note: Gene regulatory network of grass carp was reconstructed using zebrafish gene regulatory network (GRN) in STRING database. The pink nodes were CiHSP genes, and the light blue nodes were function genes interacting with CiHSP genes in grass carp.
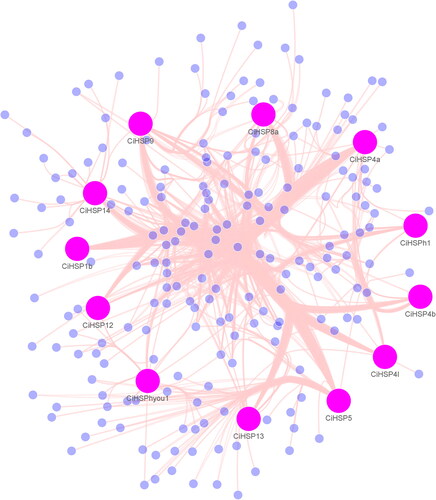
Figure 5. GO analysis of gene regulatory networks of CiHSP genes in grass carp.
Note: GO enrichment analysis was performed using topGO package. Red dot, BP (biological process); green dot, MF (molecular function), and blue dot, CC (cellular component) represent three types of GO terms. The dot size represents the number of genes enriched in the GO term. The ordinate is the term of GO, and the abscissa is the p-value of topGO enrichment analysis, −log10 (p).
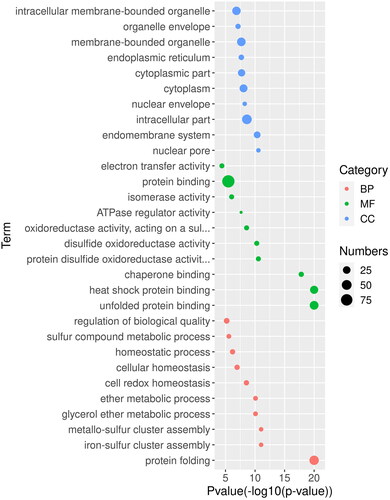
Expression analysis of CiHSP genes in tissue development and response to stress
To further explore the biological functions of these CiHSP genes, we examined publicly available data for their expression levels in six tissues: kidney, liver, head kidney, spleen, brain and embryo (). CiHSP genes were highly expressed in the kidney and brain. The head kidney is responsible for many immunological processes and is exposed to various stimuli, while the brain has high energy requirements [Citation33]. Therefore, it is possible that CiHSP genes play a role in maintaining energy metabolism in the lifespan of grass carp by being highly expressed in tissues with high energy requirements [Citation34]. When grass carp were subjected to high-density culture stress, CiHSP genes were found to have high expression levels in the brain and muscle (). Both the brain and muscle have high energy requirements and can be damaged under energy deficiency conditions. Thus, the high expression of CiHSP genes may help to clear reactive oxygen species produced during energy metabolism, reducing stress in tissues with high energy demands. When grass carp were subjected to high temperature stress, most CiHSP genes were highly expressed under these conditions (). As shown, most CiHSP genes were significantly upregulated at the higher temperature (28 °C) compared to the lower temperature (18 °C), with the exception of three members (CiHSP1b, CiHSP4b and CiHSP4l) that had lower expression levels in all samples. The expression levels of the CiHSP genes in the three RNA-Seq experiments can be found in .
Figure 6. Expression profile of CiHSP genes in grass carp.
Note: The expression levels of CiHSP genes were downloaded from grass carp genome website and SRA database;, all expression data were clustered and plotted using the heatmap function in R platform. (A) Expression profile of CiHSP genes in six tissues; (B), expression profile of CiHSP genes response to high density stress; S group: Grass carp high-density culture (40 kg m−3); B group: normal culture (3 kg m−3); MUS represents muscle in S group, MUB represents muscle in B group; INS represents intestine in S group, INB represents intestine in B group; BRS represents brain in S group, BRB represents brain in B group; (C), expression profile of CiHSP genes response to high temperature stress; T18 represents lower temperature (18 °C) while T28 represents higher temperature (28 °C), R1-R3 represent replaces.
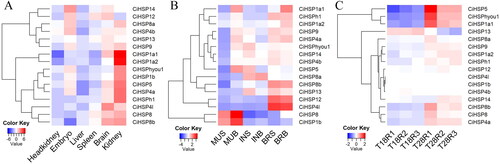
Discussion
Hsp70 is a member of the heat shock protein family, which is highly conserved among animals, with most species containing 12 to 17 members, except for zebrafish, which contains 23 members [Citation35]. In this study, 16 CiHSP genes were identified in grass carp, which is consistent with the number of Hsp70 genes found in other fish species such as channel catfish (16 members) [Citation20], large yellow croaker (17 members) [Citation21] and Japanese flounder (15 members) [Citation34]. The subfamilies HSP1, HSP4 and HSP8 were found to have more members, suggesting that gene duplications occurred during the evolution and expansion of the Hsp70 gene family [Citation36]. Based on the results of conserved motif analysis, CiHSP genes that showed closer phylogenetic relationships had similar conserved motif patterns. For example, both CiHSP1a1 and CiHSP1b are members of the HSP1 subfamily and have similar motif structures, while CiHSP1a2 from the same subfamily is missing three motifs (motifs 6, 7, and 9). The divergence within the HSP1 subfamily also contributes to the expansion of Hsp70 genes. Similar gene phenomena were also observed in the HSP4 and HSP8 subfamilies ( and ).
Previous researches have well characterized Hsp70 genes as molecular chaperones that assist in the folding and refolding of proteins by binding to denatured proteins and preventing misfolding or aggregation [Citation37,Citation38]. This process is essential for tissue development, energy metabolism, stress response and immune processes. Our GRN analysis results showed that most CiHSP genes in grass carp have wide interactions with other functional genes. GO enrichment analysis also revealed their critical roles in protein folding, chaperon binding, oxidoreductase activity and other processes that help to reduce stress in fish growth, development, immune processes and responses to environmental stress. The kidney is a major immune tissue that is highly sensitive to physiological changes or various stresses due to the regulation of key functional genes such as Hsp70 genes [Citation39]. Therefore, it is not surprising that CiHSP genes were found to be highly expressed in the kidney based on transcriptome profiles. This finding is supported by the high expression of CiHSP genes such as CiHSP1a1, CiHSP1a2, CiHSP1b, CiHSP4a, CiHSP4b, CiHSP4l, CiHSP8, CiHSP8a and CiHSP8b in the kidney tissue. These CiHSP genes belong to the three subfamilies HSP1, HSP4 and HSP8, which have expanded in grass carp. This suggests that the expansion of CiHSP genes contributes to immune processes throughout the lifespan of grass carp. In addition, CiHSPhyou1 was also found to be highly expressed in the kidney tissue, a function that is conserved in fish such as rainbow trout [Citation40].and Japanese flounder [Citation41].
Hsp70 genes have also been found to be highly expressed in fish brain tissue, suggesting important functions in this tissue. For example, HSP genes in adult zebrafish brains are induced in response to thermal shock, indicating an increase in HSP gene expression under thermal stress [Citation42]. Hsp70 genes have also been shown to increase in expression in the brain of rainbow trout in response to high water temperatures, regulating physiological changes [Citation43]. In grass carp, most CiHSP genes are highly expressed in the brain tissue, and their expression levels increase under high temperature stress. These observations suggest that there may be a similar molecular regulation mechanism in grass carp that leads to increased expression of CiHSP genes in response to high temperature stress. As shown in , 12 members of the CiHSP gene family (with the exception of CiHSP1b, CiHSP4b, CiHSP4l and CiHSP13) have increased expression levels under high temperature stress, similar to the fivefold increase in Hsp70 gene expression in response to heat stress in grass carp reported in previous research [Citation8]. These findings confirm the critical role of CiHSP genes in the response to high temperature stress, but further research is needed to fully understand the details of this process.
Conclusions
In this in silico study, we identified 16 members of the Hsp70 gene family in the grass carp genome and characterized them in terms of their gene structure, conserved domains and motifs, chromosome distribution, and molecular evolution. Our analysis revealed that these CiHSP genes are conserved in animals and have critical roles in fish growth, development and response to environmental stresses, as demonstrated by the results of our genetic regulatory network analysis and Gene Ontology annotation. Furthermore, public RNA-Seq datasets, including tissue development, high-density culture stress, and high temperature stress, confirmed the high expression levels of CiHSP genes in response to these processes. These findings provide valuable insights into the biological functions of CiHSP genes in grass carp, which could be useful for grass carp genetic breeding in the future.
Authors’ contributions
YS and GH conceived the study and acquired the funding; TZ, PL, FC and LJ performed bioinformatics analysis, YS, GH, TZ and LJ arranged and plotted the results; YS, GH and TZ wrote the manuscript. All authors read and approved the final manuscript.
Supplemental Material
Download Zip (15.8 KB)Disclosure statement
No potential conflict of interest was reported by the authors.
Data availability statement
All data that support the findings reported in this study are available from the corresponding author upon reasonable request.
Additional information
Funding
References
- Duan Y, Zhang Q, Jiang Y, et al. Dynamic transcriptional landscape of grass carp (Ctenopharyngodon idella) reveals key transcriptional features involved in fish development. Int J Mol Sci. 2022;23(19):11547. doi: 10.3390/ijms231911547.
- Boyd CE, McNevin AA, Davis RP. The contribution of fisheries and aquaculture to the global protein supply. Food Secur. 2022;14(3):1–11. doi: 10.1007/s12571-021-01246-9.
- He Y, Yu H, Zhao H, et al. Transcriptomic analysis to elucidate the effects of high stocking density on grass carp (ctenopharyngodon idella). BMC Genomics. 2021;22(1):620. doi: 10.1186/s12864-021-07924-4.
- Wang Z, Mao Z, Li X, et al. Growth performance, nutritional quality, and immune-related gene expression of the chinese mitten crab (eriocheir sinensis) in pond ecosystem as influenced by stocking density. Fishes. 2022;7(6):362. doi: 10.3390/fishes7060362.
- He J, Wang J, Xu M, et al. The cooperative expression of heat shock protein 70 KD and 90 KD gene in juvenile larimichthys crocea under Vibrio alginolyticus stress. Fish Shellfish Immunol. 2016;58:359–369. doi: 10.1016/j.fsi.2016.09.049.
- Dediu L, Docan A, Crețu M, et al. Effects of stocking density on growth performance and stress responses of bester and bester ♀ × beluga ♂ juveniles in recirculating aquaculture systems. Animals (Basel). 2021;11(8):2292. doi: 10.3390/ani11082292.
- Zhang W, Xu X, Li J, et al. Transcriptomic analysis of the liver and brain in grass carp (ctenopharyngodon idella) under heat stress. Mar Biotechnol (NY). 2022;24(5):856–870. doi: 10.1007/s10126-022-10148-6.
- Cui Y, Liu B, Xie J, et al. Effect of heat stress and recovery on viability, oxidative damage, and heat shock protein expression in hepatic cells of grass carp (ctenopharyngodon idellus). Fish Physiol Biochem. 2014;40(3):721–729. doi: 10.1007/s10695-013-9879-2.
- Segnini de Bravo MI, Chung KS. Ecophysiological behavior of caquetaia kraussii (pisces: cichlidae) exposed to different temperatures and salinities. Rev Biol Trop. 2001;49(1):149–156.
- Dettleff P, Zuloaga R, Fuentes M, et al. High-temperature stress effect on the red cusk-eel (geypterus chilensis) liver: transcriptional modulation and oxidative stress damage. Biology (Basel). 2022;11(7):990. doi: 10.3390/biology11070990.
- Li X, Liu S, Wang Y, et al. Genomic and transcriptomic landscape and evolutionary dynamics of heat shock proteins in spotted sea bass (lateolabrax maculatus) under salinity change and alkalinity stress. Biology (Basel). 2022;11(3):353. doi: 10.3390/biology11030353.
- Craig EA, Gross CA. Is hsp70 the cellular thermometer? Trends Biochem Sci. 1991;16(4):135–140. doi: 10.1016/0968-0004(91)90055-z.
- Deng Z, Sun S, Gao T, et al. The Hsp70 gene family in boleophthalmus pectinirostris: genome-wide identification and expression analysis under high ammonia stress. Animals (Basel). 2019;9(2):36. doi: 10.3390/ani9020036.
- Wang F, Li D, Chen Q, et al. Genome-wide survey and characterization of the small heat shock protein gene family in bursaphelenchus xylophilus. Gene. 2016;579(2):153–161. doi: 10.1016/j.gene.2015.12.047.
- Ritossa F. Discovery of the heat shock response. Cell Stress Chaper. 1996;1(2):97–98. doi: 10.1379/1466-1268(1996)001<0097:DOTHSR>2.3.CO;2.
- Zhang J, Zhang K, Huang J, et al. Schizothorax prenanti heat shock protein 27 gene: cloning, expression, and comparison with other heat shock protein genes after poly (I: c) induction. Animals (Basel). 2022;12(16):2034. doi: 10.3390/ani12162034.
- Giri SS, Sen SS, Sukumaran V. Role of HSP70 in cytoplasm protection against thermal stress in rohu, labeo rohita. Fish Shellfish Immunol. 2014;41(2):294–299. doi: 10.1016/j.fsi.2014.09.013.
- Yarahmadi P, Miandare HK, Fayaz S, et al. Increased stocking density causes changes in expression of selected stress- and immune-related genes, humoral innate immune parameters and stress responses of rainbow trout (Oncorhynchus mykiss). Fish Shellfish Immunol. 2016;48:43–53. doi: 10.1016/j.fsi.2015.11.007.
- Liu T, Han Y, Liu Y, et al. Genomewide identification and analysis of heat-shock proteins 70/110 to reveal their potential functions in chinese soft-shelled turtle pelodiscus sinensis. Ecol Evol. 2019;9(12):6968–6985. doi: 10.1002/ece3.5264.
- Song L, Li C, Xie Y, et al. Genome-wide identification of Hsp70 genes in channel catfish and their regulated expression after bacterial infection. Fish Shellfish Immunol. 2016;49:154–162. doi: 10.1016/j.fsi.2015.12.009.
- Xu K, Xu H, Han Z. Genome-wide identification of Hsp70 genes in the large yellow croaker (larimichthys crocea) and their regulated expression under cold and heat stress. Genes (Basel). 2018;9(12):590. doi: 10.3390/genes9120590.
- Finn RD, Clements J, Eddy SR. HMMER web server: interactive sequence similarity searching. Nucleic Acids Res. 2011;39(Web Server issue):W29–37. doi: 10.1093/nar/gkr367.
- Thompson JD, Higgins DG, Gibson TJ. CLUSTAL W: improving the sensitivity of progressive multiple sequence alignment through sequence weighting, position-specific gap penalties and weight matrix choice. Nucleic Acids Res. 1994;22(22):4673–4680. doi: 10.1093/nar/22.22.4673.
- Tamura K, Stecher G, Kumar S. MEGA11: molecular evolutionary genetics analysis version 11. Mol Biol Evol. 2021;38(7):3022–3027. doi: 10.1093/molbev/msab120.
- Bailey TL, Boden M, Buske FA, et al. MEME SUITE: tools for motif discovery and searching. Nucleic Acids Res. 2009;37(Web Server issue):W202–8. doi: 10.1093/nar/gkp335.
- Chen C, Chen H, Zhang Y, et al. TBtools: an integrative toolkit developed for interactive analyses of big biological data. Mol Plant. 2020;13(8):1194–1202. doi: 10.1016/j.molp.2020.06.009.
- Wang Y, Tang H, Debarry JD, et al. MCScanX: a toolkit for detection and evolutionary analysis of gene synteny and collinearity. Nucleic Acids Res. 2012;40(7):e49. doi: 10.1093/nar/gkr1293.
- Krzywinski M, Schein J, Birol I, et al. Circos: an information aesthetic for comparative genomics. Genome Res. 2009;19(9):1639–1645. doi: 10.1101/gr.092759.109.
- Szklarczyk D, Gable AL, Nastou KC, et al. The STRING database in 2021: customizable protein-protein networks, and functional characterization of user-uploaded gene/measurement sets. Nucleic Acids Res. 2021;49(D1):D605–d612. doi: 10.1093/nar/gkaa1074.
- Shannon P, Markiel A, Ozier O, et al. Cytoscape: a software environment for integrated models of biomolecular interaction networks. Genome Res. 2003;13(11):2498–2504. doi: 10.1101/gr.1239303.
- Udhaya Kumar S, Balasundaram A, Anu Preethi V, et al. Integrative ontology and pathway-based approach identifies distinct molecular signatures in transcriptomes of esophageal squamous cell carcinoma. Adv Protein Chem Struct Biol. 2022;131:177–206.
- Patro R, Duggal G, Love MI, et al. Salmon provides fast and bias-aware quantification of transcript expression. Nat Methods. 2017;14(4):417–419. doi: 10.1038/nmeth.4197.
- Kumar G, Hummel K, Razzazi-Fazeli E, et al. Proteome profiles of head kidney and spleen of rainbow trout (Oncorhynchus mykiss). Proteomics. 2018;18(17):e1800101. doi: 10.1002/pmic.201800101.
- Kregel KC. Heat shock proteins: modifying factors in physiological stress responses and acquired thermotolerance. J Appl Physiol (1985). 2002;92(5):2177–2186. doi: 10.1152/japplphysiol.01267.2001.
- Santacruz H, Vriz S, Angelier N. Molecular characterization of a heat shock cognate cDNA of zebrafish, hsc70, and developmental expression of the corresponding transcripts. Dev Genet. 1997;21(3):223–233. doi: 10.1002/(SICI)1520-6408(1997)21:3<223::AID-DVG5>3.0.CO;2-9.
- Günther E, Walter L. Genetic aspects of the hsp70 multigene family in vertebrates. Experientia. 1994;50(11–12):987–1001. doi: 10.1007/BF01923453.
- Liu J, Liu J, Guo SY, et al. HSP70 inhibitor combined with cisplatin suppresses the cervical cancer proliferation in vitro and transplanted tumor growth: an experimental study. Asian Pac J Trop Med. 2017;10(2):184–188. doi: 10.1016/j.apjtm.2017.01.020.
- Coto ALS, Seraphim TV, Batista FAH, et al. Structural and functional studies of the Leishmania braziliensis SGT co-chaperone indicate that it shares structural features with HIP and can interact with both Hsp90 and Hsp70 with similar affinities. Int J Biol Macromol. 2018;118(Pt A):693–706. doi: 10.1016/j.ijbiomac.2018.06.123.
- Celi M, Vazzana M, Sanfratello MA, et al. Elevated cortisol modulates Hsp70 and Hsp90 gene expression and protein in sea bass head kidney and isolated leukocytes. Gen Comp Endocrinol. 2012;175(3):424–431. doi: 10.1016/j.ygcen.2011.11.037.
- Ma F, Luo L. Genome-wide identification of Hsp70/110 genes in rainbow trout and their regulated expression in response to heat stress. PeerJ. 2020;8:e10022. doi: 10.7717/peerj.10022.
- Liu K, Hao X, Wang Q, et al. Genome-wide identification and characterization of heat shock protein family 70 provides insight into its divergent functions on immune response and development of paralichthys olivaceus. PeerJ. 2019;7:e7781. doi: 10.7717/peerj.7781.
- Murtha JM, Keller ET. Characterization of the heat shock response in mature zebrafish (Danio rerio). Exp Gerontol. 2003;38(6):683–691. doi: 10.1016/s0531-5565(03)00067-6.
- Topal A, Özdemir S, Arslan H, et al. How does elevated water temperature affect fish brain? (a neurophysiological and experimental study: assessment of brain derived neurotrophic factor, cFOS, apoptotic genes, heat shock genes, ER-stress genes and oxidative stress genes). Fish Shellfish Immunol. 2021;115:198–204. doi: 10.1016/j.fsi.2021.05.002.